1. Introduction
The Qinghai–Tibet Plateau is a complex of multiple aggregated (micro) terranes with a long-term geological evolution (Yin & Harrison, Reference Yin and Harrison2000). The presence of several large ophiolite belts in the region is strong evidence of the existence of ancient oceans and the location of palaeoplate boundaries. Located in the southern margin of the Gangdese magmatic arc, the Yarlung–Zangbo Suture Zone (YZSZ) is generally regarded as a remnant of the Neo-Tethyan oceanic lithosphere that was subducted northward beneath the Lhasa terrane, with several ophiolitic remnants (fragments) distributed in a zonal pattern along the suture zone (Allègre et al. Reference Allègre, Courtillot and Tapponnier1984; McDermid et al. Reference McDermid, Aitchison, Davis, Harrison and Grove2002; Bédard et al. Reference Bédard, Hébert, Guilmette, Lesage, Wang and Dostal2009; Zhou et al. Reference Zhou, Yang, Zhao, Ma, Xiong, Xu, Chen and Tian2015).
Subduction zones are dominant physical and chemical systems (Stern, Reference Stern2002) that are vital for reconstructing the evolution of Earth. The subduction process of the Neo-Tethys Ocean has always been a controversial topic in geological research. To date, with continuous research data acquisition, different Early Cretaceous geodynamic models have been proposed: flat-slab (low-angle) subduction (Coulon et al. Reference Coulon, Maluski, Bollinger and Wang1986; Ding & Lai, Reference Ding and Lai2003; Kapp et al. Reference Kapp, Yin, Harrison and Ding2005, Reference Kapp, Decelles, Gehrels, Heizler and Ding2007; Zhang et al. Reference Zhang, Zhang, Tang and Xia2012; Wang et al. Reference Wang, Ding, Liu, Zhang and Yue2017), high-angle subduction (Zhu et al. Reference Zhu, Zhao, Pan, Lee, Kang, Liao, Wang, Li, Dong and Liu2009), intra-oceanic subduction (Aitchison et al. Reference Aitchison, Badengzhu Davis, Liu, Luo, Malpas, McDermid, Wu, Ziabrev and Zhou2000; McDermid et al. Reference McDermid, Aitchison, Davis, Harrison and Grove2002; Xu et al. Reference Xu, Zheng, Feng, Shan, Wei, Zhang, Zhang, Ma and Pang2009) and stable oblique subduction (Ji et al. Reference Ji, Wu, Chung, Li and Liu2009). Additionally, the beginning of the subduction of the palaeoplate has been proposed to be from the Late Triassic to the Early Cretaceous (Ji et al. Reference Ji, Wu, Chung, Li and Liu2009; Zhu et al. Reference Zhu, Zhao, Niu, Mo, Chung, Hou, Wang and Yuan2011; Kang et al. Reference Kang, Xu, Wilde, Feng, Chen, Wang, Fu and Pan2014; Huang et al. Reference Huang, Xu, Chen, Kang and Dong2015; Zhong et al. Reference Zhong, Dai, Wang, Li and Wei2016; XH Wang et al. Reference Wang, Lang, Klemd, Deng and Tang2021). Therefore, the exact timing of the Neo-Tethys subduction and evolution model during the Early Cretaceous is still inconclusive. In contrast to the central and northern regions of the Lhasa terrane, numerous studies have shown that there are only a few reports of Early Cretaceous magmatic rocks in the southern margin of the Lhasa terrane (Ji et al. Reference Ji, Wu, Chung, Li and Liu2009; Zhu et al. Reference Zhu, Zhao, Niu, Mo, Chung, Hou, Wang and Yuan2011), which might be one of the shortcomings in elucidating the evolution of the Neo-Tethys during the Early Cretaceous (Wang et al. Reference Wang, Ding, Liu, Zhang and Yue2017).
Defined by Defant and Drummond (Reference Defant and Drummond1990), adakite refers to high-silicon, -Sr/Y and -La/Yb volcanic and intrusive rocks that are considered to be formed by the melting of young, hot, subducted slabs. However, recent studies have shown that adakitic rocks can be formed in different tectonic environments and by diverse mechanisms (Stern & Kilian, Reference Stern and Kilian1996; Zhang et al. Reference Zhang, Wang, Liu and Wang2002; Condie Reference Condie2005; Wang et al. Reference Wang, McDermott, Xu, Bellon and Zhu2005; Guo et al. Reference Guo, Wilson and Liu2007; Shahbazi et al. Reference Shahbazi, Taheri, Azizi, Asahara, Siebel, Maanijou and Rezai2021). Therefore, adakites have important research value for subduction zones, crustal thickening mechanisms and lower crustal evolution (Zhang et al. Reference Zhang, Wang, Liu and Wang2002).
Distributed in the Lhasa terrane, a large number of adakitic rocks (adakite) that erupted (or emplaced) in the Late Cretaceous and Cenozoic have been reported (Guan et al. Reference Guan, Zhu, Zhao, Zhang, Liu, Li, Yu and Mo2010; Ma et al. Reference Ma, Wang, Wyman, Li, Jiang, Yang, Gou and Guo2013; Zheng et al. Reference Zheng, Hou, Gong, Wei, Sun, Zhang, Fu, Huang, Li and Li2014; Chen et al. Reference Chen, Yang, Xiong, Zhang, Lai and Chen2015; Lu et al. Reference Lu, He and Klemd2020; Wang et al. Reference Wang, Zhai, Hu, Chung, Tang, Wang, Zhu, Wu and Huang2020), which record the evolution of the Neo-Tethys Ocean subduction and the geological history after the collision of the India–Eurasian plate. Many Late Cretaceous adakite rocks in the southern margin of the Lhasa terrane have gradually clarified the evolution of the Neo-Tethys during the Late Cretaceous (Wen et al. Reference Wen, Chung, Song, Iizuka, Yang, Ji, Liu and Galleta2008; Guan et al. Reference Guan, Zhu, Zhao, Zhang, Liu, Li, Yu and Mo2010; ZM Zhang et al. Reference Zhang, Zhao, Santosh, Wang, Dong and Shen2010; KJ Zhang et al. Reference Zhang, Zhang, Tang and Xia2012; Ma et al. Reference Ma, Wang, Wyman, Li, Jiang, Yang, Gou and Guo2013; Zhao et al. Reference Zhao, Hu, Lu and Wu2013; Zheng et al. Reference Zheng, Hou, Gong, Wei, Sun, Zhang, Fu, Huang, Li and Li2014; Chen et al. Reference Chen, Yang, Xiong, Zhang, Lai and Chen2015; Xu et al. Reference Xu, Zhang, Luo, Guo and Yang2015; Dai et al. Reference Dai, Li, Ding, Huang and Cao2018). In contrast, there are only a handful of reports on Early Cretaceous adakite (or adakitic) rocks (Yao et al. Reference Yao, Li, Wang, Gu, Tang and Hui2006; Kang et al. Reference Kang, Xu, Chen and Wang2009; Zhu et al. Reference Zhu, Zhao, Pan, Lee, Kang, Liao, Wang, Li, Dong and Liu2009). Accordingly, to better constrain the evolution model of the Neo-Tethys during the Early Cretaceous, more data are needed.
Here, we report new zircon U–Pb geochronology, zircon Hf isotope analyses, whole-rock geochemistry and Sr–Nd isotope results for the Early Cretaceous adakitic quartz diorite rocks that occur in the Liuqiong and Gongga areas along the southern margin of the Gangdese magmatic arc. We discuss the petrogenesis, magma evolution and tectonic significance of the Early Cretaceous adakite, providing new data for elucidating the Neo-Tethys evolution during the Early Cretaceous.
2. Geological setting
The Qinghai–Tibet Plateau, which is also referred to as ‘the combined plateau’ (Dewey, Reference Dewey2005; Xu et al. Reference Xu, Yang, Li, Zhang, Zeng and Jiang2006; Fig. 1a), is essentially composed of four blocks: the Songpan–Ganzi, Qiangtang, Lhasa and Himalayan terranes, from north to south (Yin & Harrison, Reference Yin and Harrison2000). The Lhasa terrane (Gangdese) is an E–W-extending structure-magmatic belt that is ∼100–300 km wide and 2500 km long (Pan et al. Reference Pan, Mo, Hou, Zhu, Wang, Li, Zhao, Geng and Liao2006; Zhu et al. Reference Zhu, Pan, Mo, Wang, Liao, Zhao, Dong and Yong2006). As the southern and northern boundaries of the Lhasa terrane, the YZSZ and the Bangong–Nujiang Suture Zone (BNSZ) are remnants of the oceanic crust after the closure of the Yarlung–Zangbo Ocean and Bangong–Nujiang Ocean, respectively (Fig. 1b). Due to the influence of the ancient oceans on both sides of the Lhasa terrane, Mesozoic magmatism is widespread in this region. The internal magmatic rocks become younger from south to north, reflecting the northward migration of magmatic activity, which might be the product of the northward subduction of the Neo-Tethys (Chu et al. Reference Chu, Chung, Song, Liu, O’Reilly and Pearson2006; Ji et al. Reference Ji, Wu, Chung, Li and Liu2009). However, some scholars believe that the Mesozoic magmatism in the Lhasa terrane is mainly related to the southward subduction of the Bangong–Nujiang Ocean, but the effect of this subduction system may have been minimal in the Early Cretaceous (Zhu et al. Reference Zhu, Zhao, Niu, Mo, Chung, Hou, Wang and Yuan2011). Considering the large distance between the southern margin of the Gangdese and the BNSZ before crustal shortening and deformation (caused by the subduction of the Bangong–Nujiang Ocean in the north and the Neo-Tethys Ocean in the south and the collision between Qiangtang and the Lhasa and Himalayan terranes), the magmatic activity near the southern margin of the Gangdese is more likely to be the product of the northward subduction of the Neo-Tethys (Zhu et al. Reference Zhu, Zhao, Niu, Mo, Chung, Hou, Wang and Yuan2011).

Fig. 1. (a) Tectonic outline of the Tibetan Plateau. (b) The distribution of Cretaceous magmatism (modified from Zhu et al. Reference Zhu, Zhao, Niu, Mo, Chung, Hou, Wang and Yuan2011; Mamen adakite-like rocks are cited from Zhu et al. Reference Zhu, Zhao, Pan, Lee, Kang, Liao, Wang, Li, Dong and Liu2009). (c) Geological sketch of the study area (modified from a regional geological map with a scale of 1:50 000; Early Cretaceous volcanic rocks are cited from Wang et al. Reference Wang, Ding, Liu, Zhang and Yue2017).
Focusing on Cretaceous magmatism, we note that Early Cretaceous magmatism is widely distributed in the central and northern parts of the Gangdese but is extremely rare in its southern area (Zhu et al. Reference Zhu, Pan, Mo, Wang, Liao, Zhao, Dong and Yong2006; Ji et al. Reference Ji, Wu, Chung, Li and Liu2009). The magmatic rocks in the southern margin of the Gangdese erupted (or were emplaced) in the Late Cretaceous (Fig. 1b). Recently, the increase of Early Cretaceous magmatic rocks reported within the southern margin of the Gangdese has pushed the study of the subduction model of the Neo-Tethys during the Early Cretaceous to the forefront once again (Kang et al. Reference Kang, Xu, Chen and Wang2009; Zhu et al. Reference Zhu, Zhao, Pan, Lee, Kang, Liao, Wang, Li, Dong and Liu2009; Wang et al. Reference Wang, Ding, Liu, Zhang and Yue2017).
3. Field occurrence and petrography
The study area is located in Liuqiong village, c. 5 km from the north bank of the Yarlung–Zangbo River. Late Cretaceous magmatic rocks and a small amount of Early Cretaceous volcanic rocks are exposed in the study area (Wang et al. Reference Wang, Ding, Liu, Zhang and Yue2017). Additionally, geological mapping surveys have indicated that large-scale Eocene intrusive rocks are exposed towards the northwest (Fig. 1c). The Upper Triassic Changguo Formation (T3cg), Upper Triassic – Lower Jurassic Liuqiong Formation (T3J1l), Upper Jurassic – Lower Cretaceous Linbuzong Formation (J3K1l), Lower Cretaceous Takena Formation (K1t) and Palaeocene Dianzhong Formation (E1d) are the main component units (Fig. 1c). The Liuqiong Formation (T3J1l) features a complex rock assemblage, mainly including basalt, volcanic breccia, breccia crystalline tuff, limestone, breccia limestone and tuffaceous siltstone (Fig. 2). Whole-rock geochemical characteristics indicate that the volcanic rocks within the Liuqiong Formation have arc setting signatures (GM Li et al. Reference Li, Zhang, Wu, Xie, Zhu and Han2020). The sedimentary environment and provenance characteristics of clastic rocks indicate that the Liuqiong Formation was deposited in a fore-arc basin on the southern margin of Gangdese (N Li, unpub. Master’s thesis, Cheng Univ. of Technology, 2021). During the field geological survey, two porphyritic quartz diorite dykes were discovered (note: Samples D0085 and D0087 were collected from the same dyke of section A, while sample 20CG21 was collected from another dyke of section B (Figs 1c and 2)). It is clear that the porphyritic quartz diorite dykes are intrusive into the Liuqiong Formation (Figs 2 and 3); the intrusive contact between the dykes and the surrounding rocks of the Liuqiong Formation is shown in Figure 3.

Fig. 2. Schematic cross-sections.

Fig. 3. Field photographs of the quartz diorite. (a, b, d, e) The contact relations of the quartz diorite and surrounding rocks. (c, f) Fresh surfaces of quartz diorite.
The fresh surface of the Liuqiong quartz diorite dyke is light grey, and there is no obvious phenomenon of later weathering. The investigated rocks are characterized by a porphyritic texture, with phenocrysts mainly composed of grey–white plagioclase, flesh-red alkali feldspar, dark long prismatic hornblende and a small amount of granular quartz (Fig. 3c and f). The particle size of the phenocrysts is mostly c. 1 mm in diameter, and the diameter of certain phenocrysts may be in the 2–3 mm range (Fig. 4). Most phenocrysts of hornblende and feldspars are altered, forming pits and holes in both the edge and core/nucleus of the minerals. The groundmass matrix shows a fine-microcrystalline texture, mainly composed of microcrystalline plagioclase, amphibole and quartz crystals (Fig. 4). According to the strong alteration observed in the thin-section of sample 20CG21 (Fig. 4d–f), whole-rock Sr–Nd isotope analysis was conducted for the dyke from section A only.

Fig. 4. Microphotographs. (a, b) Phenocrysts in diorite D0087. (c) The matrix in the porphyritic texture (D0087). (d, e) Altered amphibole phenocrysts in the diorite of 20CG21. (f) Matrix composed of fine-grained minerals (20CG21). Abbreviations: Am – amphibole; Mic– microcline; Q – quartz; Pl – plagioclase; Ser – sericite.
4. Analytical techniques
4.a. Whole-rock geochemical analyses
Major and trace element analyses were performed at Wuhan Sample Solution Analytical Technology Co., Ltd, in Wuhan, China. Whole-rock major element analysis was conducted on an X-ray fluorescence (XRF) instrument (Primus II, Rigaku, Japan), and trace element analysis was conducted on an Agilent 7700e ICP-MS (inductively coupled plasma – mass spectrometry) instrument. Whole-rock Sr–Nd isotopes were determined at Beijing GeoAnalysis Technology Co., Ltd. Isotopic composition analyses of Sr and Nd were undertaken using a Thermo Fisher Scientific Neptune Plus multi-collector MC-ICP-MS. 87Sr/86Sr ratios were corrected for instrumental mass fractionation using the exponential fractionation law and assuming 88Sr/86Sr = 8.375209. 143Nd/144Nd ratios were corrected for instrumental mass fractionation using the exponential fractionation law and assuming 146Nd/144Nd = 0.7219. For the analytical details, refer to Yang et al. (Reference Yang, Zhang, Wu, Xie and Zhang2005). The whole-rock major and trace element data and Sr–Nd isotope results are listed in Table S1 (in the Supplementary Material available online at https://doi.org/10.1017/S0016756822000401).
4.b. Zircon U–Pb dating
Zircon U–Pb dating was conducted using LA-ICP-MS at the Beijing GeoAnalysis Technology Co., Ltd. The Resolution SE model laser ablation system (Applied Spectra, USA) was equipped with an ATL (ATLEX 300) excimer laser. The laser ablation system was coupled to an Agilent 7900 ICP-MS (Agilent, USA). Details about the tuning parameters are provided in Thompson et al. (Reference Thompson, Meffre and Danyushevsky2018). The analyses were performed using a 30 μm diameter spot at 5 Hz and a fluence of 2 J cm−2. Zircons 91500 (∼1062 Ma) and GJ-1 (∼602 Ma) were utilized as reference materials. NIST 610 and 91Zr were selected to calibrate the trace element concentrations as external reference materials and internal standard elements, respectively. Common Pb was corrected following the method of Andersen (Reference Andersen2002). Zircon U–Pb ages are given in Table S2 and Figure S1 in the Supplementary Material available online at https://doi.org/10.1017/S0016756822000401. Isoplot/Ex_ver 3 was used to calculate the U–Pb age and the weighted average age of the samples (Ludwig, Reference Ludwig2003).
4.c. Zircon Lu–Hf isotope analyses
In situ zircon Lu–Hf isotope ratio analysis experiments were conducted using a Neptune Plus LA-MC-ICP-MS coupled with an ESI NWR LA system (193 nm) at Beijing GeoAnalysis Technology Co., Ltd. The laser beam diameter was 40 μm, the energy density was 5 J cm−2 and the frequency was 8 Hz. The measured 176Hf/177Hf ratios were normalized to 179Hf/177Hf = 0.7325. The operational conditions, detailed analysis procedures and data correction methods are described in Wu et al. (Reference Wu, Yang, Xie, Yang and Ping2006). Zircon Hf isotope data are shown in Table S3, and reference materials are plotted in Figure S2, in the Supplementary Material available online at https://doi.org/10.1017/S0016756822000401.
5. Results
5.a. Geochemistry
Whole-rock geochemical data of Liuqiong quartz diorites are shown in Table S1 (in the Supplementary Material available online at https://doi.org/10.1017/S0016756822000401). The samples show varying degrees of alteration based on their petrographical characteristics and loss on ignition (LOI). One of the dykes does not show evidence of remarkable alteration (with a low LOI, samples D0087). The second dyke (samples 20CG21), however, has undergone stronger late alteration based on the high LOI values (ranging between 3.57 and 4.36 wt %). All of the samples have high SiO2 (c. 60 wt %) and Al2O3 (16.37–16.85 wt %) contents. All samples are classified as diorite in the total alkali versus silica (TAS) plot (Fig. 5a), and in the classification plot of SiO2 versus K2O, they plot in the low-K tholeiite field (Fig. 5b). The diorite samples contain 2.77–4.55 wt % MgO, and the Mg# ranges from 58 to 66, indicating no obvious fractionation.

Fig. 5. The total alkali versus silica plot (Middlemost, Reference Middlemost1994) (a) and SiO2 versus K2O classification plot (Rollinson, Reference Rollinson1993) (b) of the Liuqiong quartz diorite. Data of Mamen adakite-like rocks are cited from Zhu et al. (Reference Zhu, Zhao, Pan, Lee, Kang, Liao, Wang, Li, Dong and Liu2009).
The trace element compositions of the quartz diorite are characterized by the pronounced fractionation of light and heavy rare earth elements (LREEs and HREEs) (Fig. 6a). The total rare earth element contents (∑REE) of the samples are between 62 ppm and 79 ppm, with an average of 70 ppm. The LREE contents range from 54 ppm to 70 ppm, and the HREE contents are extremely low, with an average of 8 ppm. The LREE/HREE ratios are in the range 6.49 to 8.69, with an average (La/Yb)N ratio of 7.79, indicating prominent fractionation of LREEs over HREEs. There are no obvious Ce and Eu anomalies (Fig. 6a). In the primitive-mantle-normalized plot, the Liuqiong quartz diorite exhibits considerable enrichment in large ion lithophile elements (LILEs) and negative Nb–Ta anomalies, which may suggest an affinity with magmas generated in a subduction-related tectonic setting (Zhu et al. Reference Zhu, Zhao, Pan, Lee, Kang, Liao, Wang, Li, Dong and Liu2009).

Fig. 6. Chondrite-normalized REE patterns (a) and primitive-mantle-normalized trace element spider plots (b) for the Liuqiong quartz diorite. The chondrite values and primitive-mantle values are cited from Sun & McDonough (Reference Sun and McDonough1989). Data of the Late Cretaceous are cited from Guan et al. (Reference Guan, Zhu, Zhao, Zhang, Liu, Li, Yu and Mo2010), Zhang et al. (Reference Zhang, Zhao, Santosh, Wang, Dong and Shen2010), Ma et al. (Reference Ma, Wang, Wyman, Li, Jiang, Yang, Gou and Guo2013) and Xu et al. (Reference Xu, Zhang, Luo, Guo and Yang2015).
Notably, the Liuqiong quartz diorites display low concentrations of HREEs, Y and Yb (Y = 11.1–13.02 ppm, Yb = 1.11–1.27 ppm). In contrast, the samples are enriched in LREEs and Sr (774–1099 ppm). These characteristics, in conjunction with high Sr/Y ratios (63–93), indicate that these samples could be classified as adakites, according to the definition of Defant & Drummond (Reference Defant and Drummond1990) (Fig. 7). Overall, the REE patterns and the spider plot of trace elements of Liuqiong quartz diorite resemble those of the Mamen adakite-like rocks reported by Zhu et al. (Reference Zhu, Zhao, Pan, Lee, Kang, Liao, Wang, Li, Dong and Liu2009) and the widespread Late Cretaceous adakite-like rocks in the Lhasa terrane (Figs. 6 and 7).

Fig. 7. Sr/Y–Y plot for the Liuqiong quartz diorite (after Defant & Drummond, Reference Defant and Drummond1990). Data are from the same source as in Fig. 6.
Whole-rock Sr–Nd isotope data for five samples from the Liuqiong quartz diorite are listed in Table S1 (in the Supplementary Material available online at https://doi.org/10.1017/S0016756822000401). The initial 87Sr/86Sr and 143Nd/144Nd ratios and ϵNd(t) values were calculated based on the zircon U–Pb ages (c. 141 Ma) obtained in our study (refer to Section 5.b). All five samples have relatively homogeneous Sr and Nd isotope compositions. As shown in Table S1, the initial 87Sr/86Sr ratios of rocks range from 0.705617 to 0.705853, and the samples have moderately positive ϵNd(t) values (+5.78 to +6.24) (figure not shown; refer to Table S1).
5.b. Zircon U–Pb geochronology
To obtain the precise ages of the Liuqiong quartz diorite, three samples were collected (D0085-N3, D0087-N1 and 20CG21-N5). In the cathodoluminescence (CL) images of zircons obtained (Fig. 8), all samples have similar morphological characteristics, and most zircons are euhedral, prismatic crystals and transparent. The typical oscillatory zoning within the zircons shown in the CL images, with no resorption or inherited cores, indicates that they have a magmatic origin. The zircons vary from 110 to 180 μm in length and 40 to 100 μm in width; thus, the ratios of the two are c. 3:1 to 1:1. Twenty, 20 and 23 zircons were analysed for samples D0085-N3, D0087-N1 and 20CG21-N5, respectively. All the results obtained are listed in Table S2 (in the Supplementary Material available online at https://doi.org/10.1017/S0016756822000401). Twenty, 19 and 23 valid data points were obtained after removing the spots with low concordance (<90 %). The Th/U ratios of these zircons range from 0.32 to 0.83 (average of 0.46) (most of the results are greater than 0.4), also indicative of a magmatic origin for the investigated zircons (Belousova et al. Reference Belousova, Griffin, O’Reilly and Fisher2002; Hoskin & Black, Reference Hoskin and Black2010). Therefore, the zircon ages can be regarded as representative of the time of emplacement of the Liuqiong quartz diorite.

Fig. 8. CL images of zircon grains analysed in this study.
Twenty analytical spots on zircons from sample D0085-N3 yielded concordant 206Pb/238U ages of 138.4 ± 2.1 to 145.5 ± 2.5 Ma, with a weighted mean age of 140.2 ± 0.5 Ma (n = 19, MSWD = 1.5) (Fig. 9a, b). Nineteen analytical spots on zircons from sample D0087-N1 yielded concordant 206Pb/238U ages between 138.9 ± 1.8 and 147.5 ± 2.0 Ma, with a weighted mean age of 140.7 ± 0.5 Ma (n = 17, MSWD = 0.95) (Fig. 9c, d). For sample 20CG21-N5, similar ages were obtained from 23 analytical spots, with a weighted mean age of 137.1 ± 0.9 Ma (n = 23, MSWD = 0.09) (Fig. 9e, f). The aforementioned results indicate that the Liuqiong quartz diorite was emplaced in the Early Cretaceous.

Fig. 9. U–Pb age concordia plots.
5.c. Hf isotopes
Hf isotope analysis was performed on (near) the zircon spots for U–Pb dating. (Fig. 8). The results are shown in Table S3 (in the Supplementary Material available online at https://doi.org/10.1017/S0016756822000401). The results show that all 176Lu/177Hf values of the investigated samples are below 0.002 and that the measured 176Hf/177Hf values could represent the Hf isotopic composition of the system during zircon formation (Amelin et al. Reference Amelin, Lee, Halliday and Pidgeon1999; Hou et al. Reference Hou, Li, Zou, Qu, Shi and Xie2007; Wu et al. Reference Wu, Li, Zheng and Gao2007). The zircon ϵHf(t) values vary from +12.5 to +15.4 for sample D0085-N3, +11.5 to +14.8 for sample D0087-N1, and +12.7 to +16.0 for sample 20CG21-N5 (Fig. 10). The single-stage Hf model ages (T DM1) and two-stage Hf model ages (T DM2) range between 166–283 Ma and 181–368 Ma for sample D0085-N3, between 190–323 Ma and 220–433 Ma for sample D0087-N1, and between 137–273 Ma and 137–353 Ma for sample 20CG21-N5.

Fig. 10. ϵHf(t) versus U–Pb age plot for zircons.
6. Discussion
6.a. Alteration effects
According to the whole-rock, geochemical and microscopic characteristics, some of the investigated samples have undergone alteration (especially sample 20CG21). Therefore, it is necessary to estimate the mobility of the elements before discussing the petrogenesis and source properties of the elements. Some LILEs (Sr, Rb, etc.) often migrate during alteration, so it is not suitable to consider them in further analysis and discussion. In contrast, REEs and high-field-strength elements (HFSEs) are relatively stable (Th, Nb, Ta, Zr, Hf, Y, etc.) (Rollinson, Reference Rollinson1993). Binary plots versus LOI are employed as proxies for alteration intensity (Wang et al. Reference Wang, Ding, Zhang, Kapp, Pullen and Yue2016), and the correlation coefficient R = 0.75 is the critical point for element mobility (Polat & Hofmann, Reference Polat and Hofmann2003). In this work, the correlations between REEs (Th, Nd, Nb, Ta, La, Gd, Yb, Sm, etc.) that are commonly employed for the discussion of petrogenesis and source regions are generally weak (correlation coefficients are mostly below 0.75; figures not shown), which indicates that these elements can remain stable (or almost stable) during the process of alteration. Additionally, previous studies have already demonstrated that Zr, Hf, Fe, A1, Th, Nb, Sc and REEs are immobile during weathering (Middelburg et al. Reference Middelburg, Weijden and Woittiez1988). Consequently, these immobile elements are utilized for subsequent discussion.
6.b. Petrogenesis
According to its unique geochemical characteristics, adakite was defined as a volcanic or intrusive rock formed by partial melting of a young (<25 Ma) and hot oceanic crust when first reported (Defant & Drummond, Reference Defant and Drummond1990). Currently, it is proposed that adakite rocks form in different tectonic environments by diverse mechanisms. The main viewpoints about the petrogenesis of adakites include partial melting of the subducted continental crust (Lai & Qin, Reference Lai and Qin2013), partial melting of the (thickened) lower crust (Atherton & Petford, Reference Atherton and Petford1993; Chung et al. Reference Chung, Liu, Ji, Chu, Lee, Wen, Lo, Lee, Qian and Zhang2003; Hou et al. Reference Hou, Gao, Qu, Rui and Mo2004; Wang et al. Reference Wang, McDermott, Xu, Bellon and Zhu2005; Long et al. Reference Long, Wilde, Wang, Yuan, Wang, Li, Jiang and Dan2015; Shahbazi et al. Reference Shahbazi, Taheri, Azizi, Asahara, Siebel, Maanijou and Rezai2021), mixing of felsic and basaltic magma (Guo et al. Reference Guo, Wilson and Liu2007; Streck et al. Reference Streck, Leeman and Chesley2007), crystallization differentiation of basaltic magma (including low-pressure, fractional crystallization of plagioclase and amphibole, and high-pressure, fractional crystallization involving amphibole and garnet) (Castillo et al. Reference Castillo, Janney and Solidum1999; Gao et al. Reference Gao, Klemd, Long, Xiong and Qian2009; Dai et al. Reference Dai, Li, Ding, Huang and Cao2018), and partial melting of subducting oceanic crust (Defant & Drummond, Reference Defant and Drummond1990; Wang et al. Reference Wang, Wyman, Xu, Jian, Zhao, Li, Xu, Ma and He2007).
Numerous studies have limited the collision time between the Indian continent and the Asian continent to the range of ∼60–50 Ma through multidisciplinary studies (Najman et al. Reference Najman, Jenks, Godin, Boudagher-Fadel, Millar, Garzanti, Horstwood and Bracciali2017 and references therein); that is, the southern margin of the Lhasa terrane did not undergo the evolutionary process of continental crust subduction. In this case, the Liuqiong adakite could not be attributed to partial melting of the subducted continental crust. The SiO2 contents of the Liuqiong adakite are generally high (all c. 60 wt %), and there are no basic enclaves within the dykes. Adakitic rocks formed by the mixing of dacitic and basaltic magmas usually have high MgO contents (>4.5 wt %) and Mg# values (>66; Wang et al. Reference Wang, Xu, Jian, Bao, Zhao, Li, Xiong and Ma2006). The results of our study reveal that only some samples have high MgO and Mg# values (4.37 wt % and 64.8 on average, respectively), which are not attributed to adakites derived from a mixture of dacitic and basaltic magmas (Table S1, in the Supplementary Material available online at https://doi.org/10.1017/S0016756822000401). The above characteristic hampers the process of mixing between dacitic and basaltic magmas as the Liuqiong adakite source (Dong et al. Reference Dong, Wang, Xie, Yu and Hao2020; B Wang et al. Reference Wang, Xie, Dong, Fan, Yu and Duan2021).
In the Sm–La/Sm and La–(La/Yb)N plots (Fig. 11), the investigated samples plot close to each other, showing no trend of fractional crystallization or partial melting. However, considering the limitation of our research data, we take into account the coeval Mamen adakite (136.5 ± 1.7 Ma) (Zhu et al. Reference Zhu, Zhao, Pan, Lee, Kang, Liao, Wang, Li, Dong and Liu2009). As shown in Fig. 11, these Early Cretaceous adakites exhibit an obvious trend of partial melting. In combination with the homogeneous values of the whole-rock, Sr–Nd isotopes and zircon Hf isotope compositions (Fig. 10), we conclude that the Liuqiong adakite cannot be the fractional crystallization product of a more primitive magma (Long et al. Reference Long, Wilde, Wang, Yuan, Wang, Li, Jiang and Dan2015; Dong et al. Reference Dong, Wang, Xie, Yu and Hao2020). The Early Cretaceous Liuqiong adakites (c. 140–137 Ma) reported in this work occur near the YZSZ. After excluding the above processes related to adakite genesis and considering the emplacement age of the Liuqiong adakite, which is consistent with the Neo-Tethys subduction time (Zhu et al. Reference Zhu, Zhao, Pan, Lee, Kang, Liao, Wang, Li, Dong and Liu2009), combined with its moderately positive ϵNd(t) values and highly positive zircon ϵHf(t) values, we propose that the Liuqiong adakite more likely formed by partial melting of the subducted oceanic slab.

Fig. 11. Chemical variation plots of the Liuqiong adakite (after Long et al. Reference Long, Wilde, Wang, Yuan, Wang, Li, Jiang and Dan2015).
To further determine the petrogenesis of the Liuqiong adakite, we show a variety of adakite discriminant plots. Compared with other adakites, slab-melting adakites have higher Mg# values and lower Th contents and Th/Ce ratios (Zhu et al. Reference Zhu, Zhao, Pan, Lee, Kang, Liao, Wang, Li, Dong and Liu2009). In the SiO2–Mg# (Fig. 12a) and Th–Th/Ce (Fig. 12b) plots, all samples from the Liuqiong adakite plot in the area of slab-related adakites, also showing an arc-related affinity. Furthermore, the high contents of Cr (57.0–139.10 ppm) and Ni (22.2–47.63 ppm) for the Liuqiong adakite are similar to those from the Mamen adakite-like rocks (Zhu et al. Reference Zhu, Zhao, Pan, Lee, Kang, Liao, Wang, Li, Dong and Liu2009), which plot in the field of subduction-related adakites (Fig. 12c). The plot of Al2O3–K2O/Na2O (Fig. 12d) clearly shows that the Liuqiong adakite has a high content of Al2O3 and a low ratio of K2O/Na2O, which is also consistent with subduction-related adakites. This evidence contradicts the process of partial melting of the thickened lower crust. The relatively high, homogeneous zircon ϵHf(t) values (Fig. 10) and whole-rock ϵNd(t) values (+5.78 to +6.24) are consistent with those from Tethyan-related basalt (Mahoney et al. Reference Mahoney, Frei, Tejada, Mo, Leat and Nägler1998) and suggest that the Liuqiong adakite source magmas mainly originated from the partial melting of the Tethyan subducted oceanic slab.

Fig. 12. Discrimination plots for the Liuqiong adakite. (a) Mg#–SiO2 (after Zhu et al. Reference Zhu, Zhao, Pan, Lee, Kang, Liao, Wang, Li, Dong and Liu2009). (b) (Th/Ce)–Th (after Zhu et al. Reference Zhu, Zhao, Pan, Lee, Kang, Liao, Wang, Li, Dong and Liu2009). (c) Cr–Ni (after Guan et al. Reference Guan, Zhu, Zhao, Dong, Zhang, Li, Liu, Mo, Liu and Yuan2012). (d) (K2O/Na2O)–Al2O3 (after Jamshidi et al. Reference Jamshidi, Ghasemi, Miao and Sadeghian2018). Data are from the same source as in Fig. 6.
In summary, we propose here that the formation process of the Liuqiong adakite is related to the partial melting of the Neo-Tethyan oceanic slab.
6.c. Nature of the source region
The Liuqiong adakite exhibits considerably negative Nb–Ta anomalies, suggesting an affinity with magmas generated in a subduction-related tectonic setting (Kelemen et al. Reference Kelemen, Hanghoj and Greene2007). The values of Nb/U for the Liuqiong adakite vary from 2.51 to 2.68 (with an average of 2.61), which is between the values of fluid derived from the subduction zone (≈0.22) (Ayers, Reference Ayers1998) and those of subducted sediments (≈5.0) (Plank & Langmuir, Reference Plank and Langmuir1998), demonstrating an affinity with the subduction-related setting. Studies have shown that both slab-derived fluids and subducted-sediment melts can cause important changes in the composition of subduction-related magmas (Zhu et al. Reference Zhu, Zhao, Pan, Lee, Kang, Liao, Wang, Li, Dong and Liu2009). In the Th/Zr–Nb/Zr plot (Fig. 13a), the Liuqiong adakite shows a trend of subduction-related fluid metasomatism, which implies the addition of subducting fluids to the genesis of these rocks. Subducted sediment melts have relatively high Th and Pb contents and low Ce/Th (≈8), Ce/Pb (≈3) and Ba/Th ratios (≈111) (Mao et al. Reference Mao, Wang, Xiao, Fang, Wang and Yu2014). The Ce/Th and Ce/Pb ratios of our samples range from 9.40 to 10.14 (with an average of 9.72) and from 1.08 to 5.42 (with an average of 2.93), respectively. In addition, the average Ba/Th value of the samples is 48. Based on the aforementioned ratios, we suggest that the source of the Liuqiong adakites also has a certain amount of sediment melt. In addition, Woodhead et al. (Reference Woodhead, Hergt, Davidson and Eggins2001) proposed that the addition of sediment melt into the magma source may cause a significant increase in the Th/Yb ratio (>2). The Th/Yb values of the Liuqiong adakite are greater than 2, with an average of 2.41, reinforcing the addition of sediment melt in its magma source. The Th/Sm–Th/Yb plot (Fig. 13b) shows that Liuqiong samples have similar elemental compositions to those of the Mamen adakite-like rocks; both are considered a mixture between the Dazhu–Langceling basalts from the YZSZ and the partial melt of subducted sediment (Zhu et al. Reference Zhu, Zhao, Pan, Lee, Kang, Liao, Wang, Li, Dong and Liu2009).

Fig. 13. Th/Zr – Nb/Zr plot (after Woodhead et al. Reference Woodhead, Hergt, Davidson and Eggins2001) (a) and Th/Sm–Th/Yb plot (after Zhu et al. Reference Zhu, Zhao, Pan, Lee, Kang, Liao, Wang, Li, Dong and Liu2009) (b) for the Liuqiong adakite.
Oceanic crust basalts and ocean–pelagic sediments have significantly different Sr and Nd isotope compositions. Therefore, subducted sediment melts not only cause a change in the trace elements of arc-related magmas but also leave a remarkable fingerprint on their isotope composition (Plank & Langmuir, Reference Plank and Langmuir1998). A large amount of Sr and Nd isotope data enables quantification of the relative contribution of sediments to the arc magma associated with subduction. Accordingly, the Dazhu–Langceling basalts from the YZSZ (Zhang et al. Reference Zhang, Mahoney, Mo, Ghazi, Milani, Crawford, Guo and Zhao2005) and the Indian Ocean pelagic sediment (Ben Othman et al. Reference Ben Othman, White and Patchett1989) are considered the normal mid-ocean ridge basalt (N-MORB) and Neo-Tethyan oceanic sediment source components, respectively. Thus, we can clearly determine from the 87Sr/86Sri versus ϵNd(t) plot that the source magma of the Liuqiong adakite could be regarded as a mixture of Neo-Tethyan subducting sediments (c. 5–10 %) and Tethyan basalt (Fig. 14a). Furthermore, the Indian MORB, which probably represents newly formed oceanic crust, exhibits zircon ϵHf(t) values consistent with those of the Liuqiong adakite (Fig. 14b) (Zhu et al. Reference Zhu, Zhao, Pan, Lee, Kang, Liao, Wang, Li, Dong and Liu2009). Of course, we note that the Sr isotope compositions of the sample have a small deviation from the modelled curve, and we believe that an alternative and reasonable explanation could be the previous interaction of the Tethyan oceanic crust with seawater, which could raise the Sr isotope composition of the oceanic floor basalts (Fig. 14a).

Fig. 14. ϵNd(t)–(87Sr/86Sr)i and ϵNd(t)–ϵHf(t) plots for the Liuqiong adakite (after Zhu et al. Reference Zhu, Zhao, Pan, Lee, Kang, Liao, Wang, Li, Dong and Liu2009).
The subducted oceanic slab-derived adakite might be influenced by slight interaction with peridotite in the overlying mantle wedge during magma ascent (Zhu et al. Reference Zhu, Zhao, Pan, Lee, Kang, Liao, Wang, Li, Dong and Liu2009). Notably, the Cr, Ni and MgO contents of the Liuqiong adakite (with averages of 99 ppm, 35 ppm and 3.65 wt %, respectively) are higher than those of typical adakites (Defant et al. Reference Defant, Richerson, de Boer, Stewart, Maury, Bellon, Drummond, Feigenson and Jackson1991). The experimental results show that during ascent through the mantle wedge, the contents of Cr, Ni and MgO increase significantly because of the metasomatic reactions within the overlying mantle wedge (Rapp et al. Reference Rapp, Shimizu, Norman and Applegate1999). In the case of a low level of interaction with peridotite in the mantle wedge during magma ascent, the increase in the above-mentioned elements may not be significant enough (B Wang et al. Reference Wang, Xie, Dong, Fan, Yu and Duan2021). Such an interpretation is enlightening for determining the evolution of the Liuqiong adakite, indicating that the Liuqiong adakite magma clearly interacted with peridotite in the mantle wedge during ascent.
The Liuqiong adakite magma was derived from the partial melting of the Neo-Tethyan oceanic slab (MORB + sediment + fluid) and interacted with peridotite in the mantle wedge during magma ascent.
6.d. Geodynamic implications
The subduction initiation of the Neo-Tethys has always a widely debated issue in geosciences and has been proposed, by many studies, to have occurred sometime from the Late Triassic to the Early Cretaceous (Ji et al. Reference Ji, Wu, Chung, Li and Liu2009; Zhu et al. Reference Zhu, Zhao, Niu, Mo, Chung, Hou, Wang and Yuan2011; Kang et al. Reference Kang, Xu, Wilde, Feng, Chen, Wang, Fu and Pan2014; Huang et al. Reference Huang, Xu, Chen, Kang and Dong2015; Zhong et al. Reference Zhong, Dai, Wang, Li and Wei2016; Meng et al. Reference Meng, Mooney, Fan, Liu and Wei2021; XH Wang et al. Reference Wang, Lang, Klemd, Deng and Tang2021); other scholars believe that the Neo-Tethys was still not subducting during the Early Cretaceous (Wu et al. Reference Wu, Zhao, Barosh and Ye2016). With the affinities of subduction-related adakites, the Liuqiong quartz diorites reported in this work are closely related to the subduction of the Neo-Tethyan oceanic slab. The emplacement age of c. 141 Ma from our samples illustrates that the northward subduction of the Neo-Tethyan oceanic crust was already active prior to 141 Ma or even earlier.
The subduction pattern of the Neo-Tethys Ocean during the Early Cretaceous has also been debated in the literature. The prevalent viewpoints about the subduction model include flat-lying (low-angle) subduction (Coulon et al. Reference Coulon, Maluski, Bollinger and Wang1986; Ding & Lai Reference Ding and Lai2003; Kapp et al. Reference Kapp, Yin, Harrison and Ding2005, Reference Kapp, Decelles, Gehrels, Heizler and Ding2007; Zhang et al. Reference Zhang, Zhang, Tang and Xia2012; Wang et al. Reference Wang, Ding, Liu, Zhang and Yue2017), high-angle subduction (Zhu et al. Reference Zhu, Zhao, Pan, Lee, Kang, Liao, Wang, Li, Dong and Liu2009) and intra-ocean subduction (Aitchison et al. Reference Aitchison, Badengzhu Davis, Liu, Luo, Malpas, McDermid, Wu, Ziabrev and Zhou2000; McDermid et al. Reference McDermid, Aitchison, Davis, Harrison and Grove2002; Xu et al. Reference Xu, Zheng, Feng, Shan, Wei, Zhang, Zhang, Ma and Pang2009). Additionally, an oblique subduction setting has been proposed (Ji et al. Reference Ji, Wu, Chung, Li and Liu2009). Based on the available data, Early Cretaceous magmatism was widely distributed in the central and northern parts of the Lhasa terrane (Ding et al. Reference Ding, Kapp, Zhong and Deng2003; Kapp et al. Reference Kapp, Decelles, Gehrels, Heizler and Ding2007). Although Early Cretaceous magmatism has been reported (Zhu et al. Reference Zhu, Zhao, Pan, Lee, Kang, Liao, Wang, Li, Dong and Liu2009; Wang et al. Reference Wang, Ding, Liu, Zhang and Yue2017), evidence of this magmatic activity is rare at the southern margin of the Lhasa terrane, which might not be attributed to stable oblique subduction (Gutscher et al. Reference Gutscher, Maury, Eissen and Bourdon2000).
Located near the sampling site of our study, the Liqiongda Early Cretaceous volcanic rocks were most likely produced in a typical active continental margin setting (Wang et al. Reference Wang, Ding, Liu, Zhang and Yue2017), which does not support the suggestion of intra-ocean subduction during the Early Cretaceous. The Cuocun gabbro in the southern Lhasa terrane also supports the conclusion that the Early Cretaceous magmatic rocks reassembled to a continental margin arc setting (He et al. Reference He, Lang, Li Liang, Wang, Deng, Yin, Xie, Yang and Zhang2020). Furthermore, previous studies have suggested that the Zedong terrane is a remnant of a Cretaceous intra-ocean subduction system in the southern margin of the Lhasa terrane (Aitchison et al. Reference Aitchison, Badengzhu Davis, Liu, Luo, Malpas, McDermid, Wu, Ziabrev and Zhou2000). A subsequent study has show that the Zedong terrane formed in the Late Jurassic (McDermid et al. Reference McDermid, Aitchison, Davis, Harrison and Grove2002) and is more likely to be a part of the Gangdese arc (Zhang et al. Reference Zhang, Liu, Wu, Ji and Wang2014). Recently, Liu et al. (Reference Liu, Zhong, Sun, Yakymchuk, Gu, Tang, Zhong, Cao, Liu and Xia2020) also suggested that the Zedong ophiolite, which may represent an intra-oceanic arc system, formed in the Late Jurassic. We can thus consider that models of intra-ocean subduction and stable oblique subduction are not suitable for explaining the evolution of the Neo-Tethys during the Early Cretaceous.
Different angles of oceanic slab subduction will produce different effects in the overlying plate. Normally, the contact surface between a low-angle (flat-lying) subducting oceanic plate and the overlying plate is large; in this case, the coupling between the two plates is tight, so the corresponding arc magmatism is relatively scarce. The overlying plate is strongly compressed and is the locus of significant orogeny (Yan et al. Reference Yan, Chen, Xiong, Wang, Xie and Xu2020). Therefore, the lull of arc magmatism and landward migration and the deformation of the overlying plate are important geological bases for recognizing (or proposing) the low-angle subduction of oceanic crust. Based on comprehensive regional data, the Mesozoic magma activities within the Gangdese arc shifted from south to north, showing a trend of landward migration (Wang et al. Reference Wang, Ding, Liu, Zhang and Yue2017); they may be the response to the location of the front part of the flat-lying Neo-Tethyan oceanic slab (Dai, Reference Dai2012). Specifically, volcanic activity migrated northwards in response to the shallowing of the subduction angle of the downgoing Neo-Tethyan slab from the latest Jurassic through the Early Cretaceous (Coulon et al. Reference Coulon, Maluski, Bollinger and Wang1986; Kapp et al. Reference Kapp, Yin, Harrison and Ding2005; Leier et al. Reference Leier, Decelles, Kapp and Gehrels2007). In addition, many leucogranites within the Gangdese batholith record the thickening of the crust and mountain-building process, which is the response of the northward Neo-Tethyan subduction during the Early Cretaceous (∼140–130 Ma) (Ding & Lai, Reference Ding and Lai2003). From our study data, we conclude that the subduction of the Neo-Tethys began before the Early Cretaceous (∼141 Ma). Stratigraphic data indicate the uplift and deformation of the Lhasa terrane in the Early Cretaceous (Leier et al. Reference Leier, Decelles, Kapp and Gehrels2007). We favour the interpretation that the Neo-Tethyan oceanic crust was subducted beneath the Lhasa terrane at a low angle during the Early Cretaceous.
The discovery of Mamen adakite-like rocks (∼137 Ma) and contemporary volcanic rocks (∼130 Ma; Zhu et al. Reference Zhu, Mo, Zhao, Xu, Zhou, Sun, Wang, Chen, Dong and Zhou2008) in the study region has prompted some researchers to support the model of high-angle subduction of the Neo-Tethyan oceanic crust (Zhu et al. Reference Zhu, Zhao, Pan, Lee, Kang, Liao, Wang, Li, Dong and Liu2009). Considering that there is no report of large-scale, Early Cretaceous magma on the southern margin of the Gangdese batholith, we believe that the high-angle subduction model may not be appropriate. Adakites form under high temperatures (above 700 °C), even corresponding to a relatively shallow subducted-slab angle (Gutscher et al. Reference Gutscher, Maury, Eissen and Bourdon2000), which may account for the sporadic Early Cretaceous adakites in the southern margin of the Lhasa terrane. Systematic temperature changes are revealed by variations in zircon Ti content. The Ti contents of the investigated Liuqiong quartz diorite zircons vary from 1.88 to 7.00 ppm. According to the crystallization thermometer for zircon (Watson et al. Reference Watson, Wark and Thomas2006), we can infer that the average crystallization temperature of these zircons is 745 °C. Considering the crystallization process of minerals in the magma system, the zircon crystallization temperature could serve as the lower temperature of melting originating from the subducted oceanic slabs. Hence, the partial melting temperature should be higher than the temperature obtained by the zircon Ti thermometer (CW Li et al. Reference Li, Zeng, Li and Chen2020). Therefore, the partial melting temperature to form the Liuqiong adakite melt should be higher than ∼745 °C, reaching the temperature conditions for adakite formation considered by previous studies (Gutscher et al. Reference Gutscher, Maury, Eissen and Bourdon2000).
Recent geological survey reveals that the ages of accretionary complexes gradually become younger from the Tangjia–Sumdo area in the north, to the Yarlung–Zangbo River in the south (GM Li et al. Reference Li, Zhang, Wu, Xie, Zhu and Han2020), for example, the Permian – Middle Triassic (P–T2) accretionary complex, Late Triassic – Early Jurassic (T3–J1) accretionary complex (Fig. 15) and Cretaceous (K1–2) accretionary complex (unpublished data), from north to south. Therefore, some studies consider that continuous southward accretion took place at the SE margin of Gangdese after the Late Palaeozoic and that the Tangjia–Sumdo Palaeo-Tethys and the Neo-Tethys might represent different evolutionary phases of the same ocean (GM Li et al. Reference Li, Zhang, Wu, Xie, Zhu and Han2020; Xie et al. Reference Xie, Li, Li, Zhang, Wang, Dong and Hao2020). Recent studies have identified numerous Jurassic granites in the Sumdo area, which are attributed to remelting of the crust caused by the underlying mass of mantle-derived material (Song et al. Reference Song, Xie, Gao, Yu, Wang, Duan and Hao2022), and suggest that the Neo-Tethyan oceanic plate was not in a state of flat-slab subduction in the Jurassic (since the upwelling of large amounts of mantle-derived material could not be achieved during flat-slab subduction). In addition, volcanic rocks of the Yeba Formation (YBF) (∼183–174 Ma) suggest a back-arc extensional setting for the Early–Middle Jurassic (Wei et al. Reference Wei, Zhao, Niu, Zhu, Liu, Wang, Hou, Mo and Wei2017). These studies indicate that the Neo-Tethys Ocean subducted at a normal angle in the Jurassic. Moreover, fore-arc extension produced ophiolite in the Zedang area during the period 160–150 Ma (Liu et al. Reference Liu, Zhong, Sun, Yakymchuk, Gu, Tang, Zhong, Cao, Liu and Xia2020; Dai et al. Reference Dai, Wang, Stern, Yang and Shen2021), also demonstrating that the Neo-Tethys Ocean did not enter the stage of flat-slab subduction at this time (Fig. 15a). In our study, the Liuqiong adakite magma (∼140 Ma) shows some degree of peridotite interaction within the overlying mantle wedge. Considering the above studies, we believe that the Neo-Tethys Ocean was most likely formed during this stage (c. 140 Ma), transitioning to a flat-slab setting soon after but maintaining a tongue-shaped mantle wedge to account for the peridotite interaction during magma ascent, which may account for the slight interaction with the tongue-shaped mantle wedge (Fig. 15b). Subsequently, the mantle wedge disappeared, and magmatism ceased, especially during the period between 137 and 120 Ma in the study area. The latest evidence of ocean subduction-related magmatic rocks was found in upper Lower Cretaceous (∼120–112 Ma) strata in the Zedang Trench basin in the form of detrital zircons (Y Zhong, unpublished data).
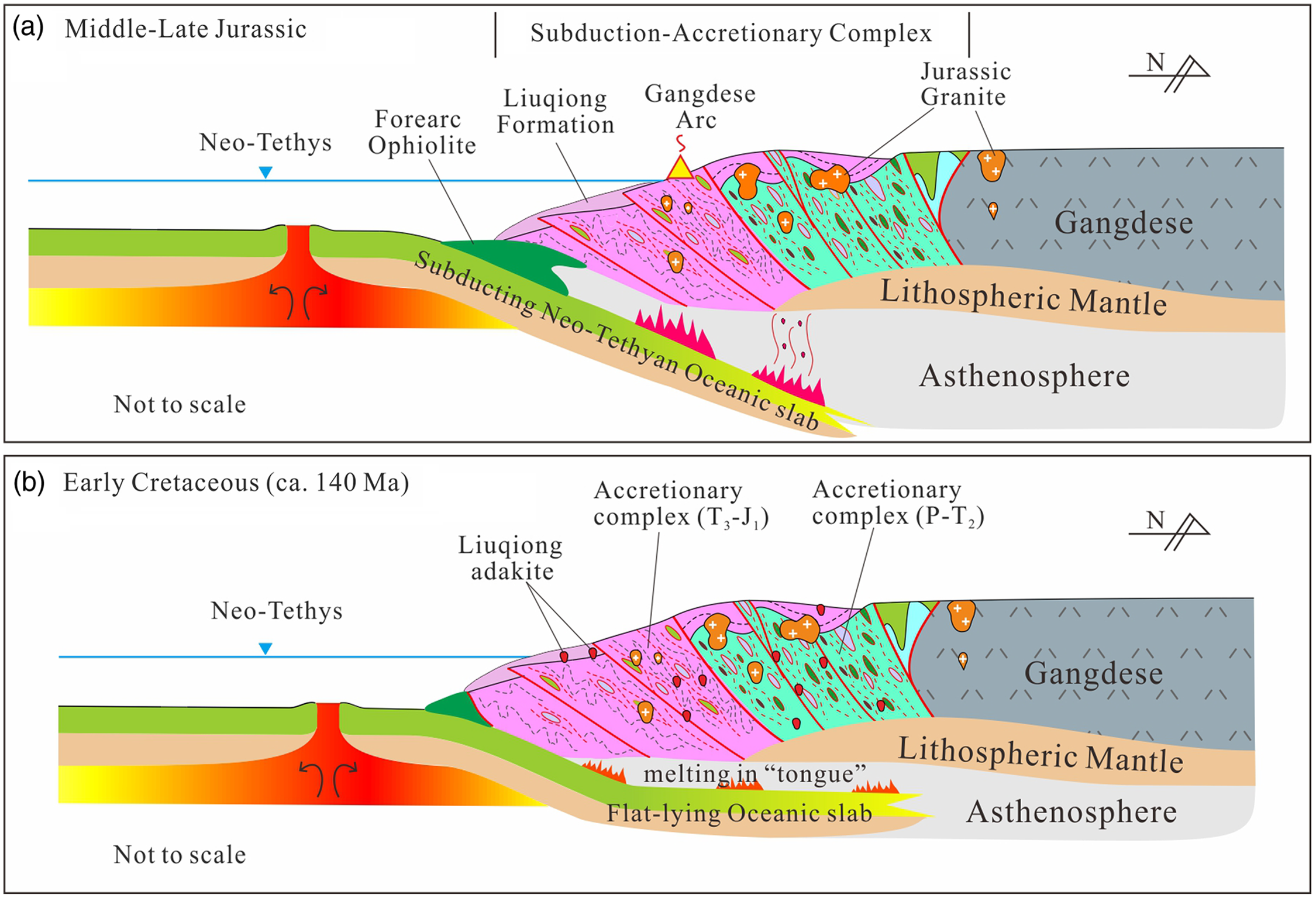
Fig. 15. Schematic tectonic evolution models of the SE margin of Gangdese. (a) Normal oblique subduction of the Neo-Tethys Ocean during the Middle–Late Jurassic. (b) Flat-slab subduction of the Neo-Tethyan oceanic slab and the generation of the Liuqiong adakite in the Early Cretaceous. More details are provided in the text.
Nevertheless, the classical flat-slab subduction model predicts a ‘wide adakitic magma arc’, whereas a narrow calc-alkaline arc (volcanic line) is predicted in the normal situation (Gutscher et al. Reference Gutscher, Maury, Eissen and Bourdon2000). The sporadic Early Cretaceous rocks in the southern margin of the Lhasa terrane are not compatible with a steeply dipping Neo-Tethyan slab. In our proposed tectonic model, we emphasize a tongue-shaped mantle wedge during the Early Cretaceous (Fig. 15b), and we interpret the slight interaction with the mantle wedge from this finding. Additionally, because of the tongue-shaped mantle wedge, the magmatism caused by flat-slab subduction would have been small-scale, which is not widely reported for normal arc magmatic rocks. Although a ‘wide magma arc’ is predicted by Gutscher et al. (Reference Gutscher, Maury, Eissen and Bourdon2000), we may consider that this limited magmatism resulted in only small-scale intrusive rocks. For example, the flat subduction of the Palaeo-Pacific Plate during the Middle Triassic produced localized granitic intrusions in South China (Zhang et al. Reference Zhang, Zhang, Dilek, Zhu, Wu, Chen and Chen2021).
Consequently, we conclude that the sporadic Early Cretaceous adakites at the southern margin of the Lhasa terrane were triggered by low-angle subduction of the Neo-Tethyan oceanic lithosphere.
7. Conclusions
-
(1) LA-ICP-MS zircon U–Pb dating indicates that the porphyritic Liuqiong quartz diorite was emplaced c. 141-137 Ma, providing evidence for Early Cretaceous magmatism along the southern margin of the Lhasa terrane.
-
(2) Liuqiong quartz diorite has high contents of SiO2, Al2O3 and Sr, in combination with low concentrations of HREEs and Y, which is similar to the geochemical signature of typical adakites.
-
(3) The Liuqiong adakite was probably derived from the partial melting of the subducted Neo-Tethyan slab (MORB + sediment + fluid) and the subsequent interaction with mantle peridotite within the overlying mantle wedge.
-
(4) We favour the viewpoint that the Neo-Tethyan oceanic lithosphere was flat-lying beneath the Lhasa terrane during the Early Cretaceous.
Supplementary material
To view supplementary material for this article, please visit https://doi.org/10.1017/S0016756822000401
Acknowledgements
We thank Bo Li, Junge Qin, Yuanyin Jia, Tengxiao Ma and Zhichao Cao for their assistance in writing. We also thank the editor and two anonymous reviewers for their handling of the manuscript and constructive comments. This study was supported financially by the National Natural Science Foundation of China (Grant No. 41972118) and the Gangdese-Himalayan Copper Resource Base Investigation Project (Grant No. DD20160015).
Conflict of interest
The authors declare that they have no known competing financial interests or personal relationships that could have appeared to influence the work reported in this paper.