1. Introduction
During the Palaeogene (c. 65–23 Ma), an important climatic transition took place from relatively warm early Cenozoic ‘greenhouse’ conditions to late Cenozoic ‘icehouse’ conditions with episodes of transient global warming (Bohaty & Zachos, Reference Bohaty and Zachos2003; Zachos, Dickens & Zeebe, Reference Zachos, Dickens and Zeebe2008; Bijl et al. Reference Bijl, Schouten, Sluijs, Reichart, Zachos and Brinkhuis2009, Reference Bijl, Houben, Schouten, Bohaty, Sluijs, Reichart, Damsté and Brinkhuis2010; Bohaty et al. Reference Bohaty, Zachos, Florindo and Delaney2009). In the Southern Hemisphere, important palaeogeographic changes occurred, such as the deepening of the Drake Passage (Scher & Martin, Reference Scher and Martin2006; Livermore et al. Reference Livermore, Hillenbrand, Meredith and Eagles2007; Lagabrielle et al. Reference Lagabrielle, Goddéris, Donnadieu, Malavieille and Suarez2009; Eagles & Jokat, Reference Eagles and Jokat2014) and the Tasmanian Gateway (Stickley et al. Reference Stickley, Brinkhuis, Schellenberg, Sluijs, Röhl, Fuller, Grauert, Huber, Warnaar and Williams2004b ; Bijl et al. Reference Bijl, Bendle, Bohaty, Pross, Schouten, Tauxe, Stickley, McKay, Röhl, Olney, Sluijs, Escutia and Brinkhuis2013a ; Houben et al. Reference Houben, Bijl, Pross, Bohaty, Passchier, Stickley, Röhl, Sugisaki, Tauxe, Flierdt, Olney, Sangiorgi, Sluijs, Escutia and Brinkhuis2013). These tectonic changes led to important modifications in the ocean circulation, to the onset of the the Antarctic Circumpolar Current (Stickley et al. Reference Stickley, Brinkhuis, Schellenberg, Sluijs, Röhl, Fuller, Grauert, Huber, Warnaar and Williams2004b ; Houben et al. Reference Houben, Bijl, Pross, Bohaty, Passchier, Stickley, Röhl, Sugisaki, Tauxe, Flierdt, Olney, Sangiorgi, Sluijs, Escutia and Brinkhuis2013), and the formation of relatively cold intermediate water (Bijl et al. Reference Bijl, Bendle, Bohaty, Pross, Schouten, Tauxe, Stickley, McKay, Röhl, Olney, Sluijs, Escutia and Brinkhuis2013a ).
In seeking evidence for changing surface oceanographic regimes in the Southern Ocean during this time of drastic climatic changes, much knowledge can be obtained from studies of marine surface microplankton assemblages, notably dinoflagellate cysts. Organic-walled dinoflagellate cysts are a valuable tool for studying past physical and chemical conditions of surface waters, and they complement the information provided by other groups of microfossils. Dinoflagellate cysts have proven especially useful in reconstructing Palaeogene marine palaeoenvironments (Sluijs, Pross & Brinkhuis, Reference Sluijs, Pross and Brinkhuis2005 and references therein) and solving stratigraphic problems (Bijl, Sluijs & Brinkhuis, Reference Bijl, Sluijs and Brinkhuis2013b ). Dinoflagellate cyst assemblages reflect changes in the surface-water temperature and in the ocean-circulation patterns during the Palaeogene. From the late–early Eocene (~50 Ma) to the late Eocene, the dinoflagellate cyst assemblages were dominated by an endemic–Antarctic assemblage, which has been widely recognized at sites over 45°S latitude (e.g. Lentin & Williams, Reference Lentin and Williams1976; Wrenn & Hart, Reference Wrenn and Hart1988; Brinkhuis et al. Reference Brinkhuis, Munsterman, Sengers, Sluijs, Wanaar, Williams, Exon, Kennett and Malone2003a , b; Sluijs et al. Reference Sluijs, Brinkhuis, Stickley, Warnaar, Williams, Fuller, Exon, Kennett and Malone2003; Bijl et al. Reference Bijl, Houben, Schouten, Bohaty, Sluijs, Reichart, Damsté and Brinkhuis2010, Reference Bijl, Pross, Warnaar, Stickley, Huber, Guerstein, Houben, Sluijs, Visscher and Brinkhuis2011, Reference Bijl, Bendle, Bohaty, Pross, Schouten, Tauxe, Stickley, McKay, Röhl, Olney, Sluijs, Escutia and Brinkhuis2013 a; Bijl, Sluijs & Brinkhuis, Reference Bijl, Sluijs and Brinkhuis2013b ; Houben et al. Reference Houben, Bijl, Pross, Bohaty, Passchier, Stickley, Röhl, Sugisaki, Tauxe, Flierdt, Olney, Sangiorgi, Sluijs, Escutia and Brinkhuis2013). Recently, Bijl, Sluijs & Brinkhuis (Reference Bijl, Sluijs and Brinkhuis2013b) calibrated dinoflagellate cyst assemblages from sediment cores drilled in the Tasman Shelf using Vandenberghe, Speuer & Hilgen's (Reference Vandenberghe, Speijer, Hilgen, Gradstein, Ogg, Schmitz and Ogg2012) geomagnetic polarity timescale (GPTS), and proposed a cyst zonation for the early Palaeogene South Pacific Ocean which provides a correlation tool that can be applied to sites around the Southern Ocean (Bijl, Sluijs & Brinkhuis, Reference Bijl, Sluijs and Brinkhuis2013b ).
Regarding the areas close to the actual Drake Passage, Palaeogene sea-level changes caused major transgressions along the South American margin. In particular, the Austral Basin was almost completely flooded during the middle Eocene. This transgression deposited the upper member of the Río Turbio Formation and the Man Aike Formation in the southwestern part of Santa Cruz Province, Argentina (Malumián, Reference Malumián and Caminos1999). Due to their proximity to the Drake Passage (Fig. 1), marine deposits in the basin are a valuable source of information for the assessment of palaeoenvironmental and palaeoclimatic changes during the Eocene in Patagonia.

Figure 1. Map of southern Patagonia showing the extent of the Austral Basin. The inset map shows southern Santa Cruz Province and the location of the upper member of the Río Turbio Formation at: YCF Cores (cores, 50°45´14″S,72°01′39″W; 50°56′21″S,72°02′08″W; 50°35′59″S,72°13′40″W); the Cancha Carrera locality (CC) (outcrop sections, 51°14′34″S, 72°15′26″W); the Highway 40 locality (H40) (outcrop sections, 51°31′13″S, 72°15′11″W); and the outcrop section of the Man Aike Formation (50°21′45″S, 72°14′30″W). Modified from Nullo, Panza & Blasco (Reference Nullo, Panza, Blasco and Caminos1999).
This paper describes the middle to upper Eocene dinoflagellate cyst assemblages from the upper member of the Río Turbio Formation from three localities in southwestern Santa Cruz Province. Its aims are to: (1) provide a detailed biostratigraphic framework for the upper member of the Río Turbio Formation from three localities, based on the dinoflagellate cyst events and ranges determined by Brinkhuis et al. (Reference Brinkhuis, Sengers, Sluijs, Warnaar, Williams, Exon, Kennett and Malone2003b), Sluijs et al. (Reference Sluijs, Brinkhuis, Stickley, Warnaar, Williams, Fuller, Exon, Kennett and Malone2003) and Bijl, Sluijs & Brinkhuis (Reference Bijl, Sluijs and Brinkhuis2013b); (2) compare and correlate the three sections in order to integrate the stratigraphic records of the dinoflagellate cysts found in the Río Turbio Formation type area and define a dinoflagellate cyst zonation for the middle–late Eocene in the Austral Basin; and (3) interpret the palaeoenvironmental evolution of the southwestern Austral Basin during the middle to late Eocene.
2. Biostratigraphic significance of Palaeogene dinoflagellate cysts in the Southern Hemisphere
Dinoflagellate cyst assemblages in the Southern Ocean evidenced major modifications as a consequence of climatic and tectonic changes during the Palaeogene (Sluijs et al. Reference Sluijs, Brinkhuis, Stickley, Warnaar, Williams, Fuller, Exon, Kennett and Malone2003; Guerstein et al. Reference Guerstein, Guler, Brinkhuis, Warnaar, Madden, Carlini, Vucetich and Kay2010a ; Bijl et al. Reference Bijl, Bendle, Bohaty, Pross, Schouten, Tauxe, Stickley, McKay, Röhl, Olney, Sluijs, Escutia and Brinkhuis2013a ; Bijl, Sluijs & Brinkhuis, Reference Bijl, Sluijs and Brinkhuis2013b ). Bijl et al. (Reference Bijl, Pross, Warnaar, Stickley, Huber, Guerstein, Houben, Sluijs, Visscher and Brinkhuis2011, Reference Bijl, Bendle, Bohaty, Pross, Schouten, Tauxe, Stickley, McKay, Röhl, Olney, Sluijs, Escutia and Brinkhuis2013 a) and Bijl, Sluijs & Brinkhuis (Reference Bijl, Sluijs and Brinkhuis2013b) postulated that the Palaeocene and early Eocene circum-Antarctic assemblages were widely dominated by cosmopolitan early Palaeogene taxa, together with a few endemic species. By the late–early Eocene (~50 Ma), dinoflagellate cyst assemblages began to change significantly, resulting in the dominance of an endemic–Antarctic assemblage to southern high latitudes (Wrenn & Beckman, Reference Wrenn and Beckman1982, as ‘Transantarctic Flora’; Bijl et al. Reference Bijl, Pross, Warnaar, Stickley, Huber, Guerstein, Houben, Sluijs, Visscher and Brinkhuis2011; Bijl, Sluijs & Brinkhuis, Reference Bijl, Sluijs and Brinkhuis2013b ). Huber et al. (Reference Huber, Brinkhuis, Stickley, Döös, Sluijs, Warnaar, Schellenberg and Williams2004), Warnaar (Reference Warnaar2006) and Bijl et al. (Reference Bijl, Pross, Warnaar, Stickley, Huber, Guerstein, Houben, Sluijs, Visscher and Brinkhuis2011, p. 5, fig. 3) proposed that the spatial distribution of the endemic–Antarctic dinoflagellate cyst assemblage corresponded to an ocean-circulation regime with broad clockwise gyres that surrounded Antarctica. The endemic–Antarctic assemblage prevailed until the late Eocene, when the endemic species were replaced by Oligocene cosmopolitan taxa, mainly comprising heterotrophic species (Protoperidiniaceae) (Sluijs et al. Reference Sluijs, Brinkhuis, Stickley, Warnaar, Williams, Fuller, Exon, Kennett and Malone2003; Houben et al. Reference Houben, Bijl, Pross, Bohaty, Passchier, Stickley, Röhl, Sugisaki, Tauxe, Flierdt, Olney, Sangiorgi, Sluijs, Escutia and Brinkhuis2013). The demise of the endemic assemblage may have resulted from the deepening of the Drake Passage and the Tasmanian Gateway (Sluijs et al. Reference Sluijs, Brinkhuis, Stickley, Warnaar, Williams, Fuller, Exon, Kennett and Malone2003; Stickley et al. Reference Stickley, Brinkhuis, Schellenberg, Sluijs, Röhl, Fuller, Grauert, Huber, Warnaar and Williams2004b ; Guerstein et al. Reference Guerstein, Guler, Williams, Fensome and Chiesa2008; Houben et al. Reference Houben, Bijl, Guerstein, Sluijs and Brinkhuis2011, Reference Houben, Bijl, Pross, Bohaty, Passchier, Stickley, Röhl, Sugisaki, Tauxe, Flierdt, Olney, Sangiorgi, Sluijs, Escutia and Brinkhuis2013). Such tectonically induced changes and the subsequent development of an unrestricted circumpolar water flow during the early Oligocene could have disrupted the subpolar gyres and altered the environmental conditions that favoured dinoflagellate endemism allowing the arrival and proliferation of cosmopolitan taxa (Huber et al. Reference Huber, Brinkhuis, Stickley, Döös, Sluijs, Warnaar, Schellenberg and Williams2004; Guerstein et al. Reference Guerstein, Guler, Brinkhuis, Warnaar, Madden, Carlini, Vucetich and Kay2010a ).
Brinkhuis et al. (Reference Brinkhuis, Sengers, Sluijs, Warnaar, Williams, Exon, Kennett and Malone2003b), Sluijs et al. (Reference Sluijs, Brinkhuis, Stickley, Warnaar, Williams, Fuller, Exon, Kennett and Malone2003) and Bijl, Sluijs & Brinkhuis (Reference Bijl, Sluijs and Brinkhuis2013b) studied the dinoflagellate cyst assemblages from sediment cores drilled during Ocean Drilling Program (ODP) Leg 189 in the East Tasman Plateau (Site 1172). The dinoflagellate cyst events they recorded have been calibrated with magnetostratigraphic, biostratigraphic and isotope stratigraphic age models (Stickley et al. Reference Stickley, Brinkhuis, McGonigal, Chaproniere, Fuller, Kelly, Nürnberg, Pfuhl, Schellenberg, Schoenfeld, Suzuki, Touchard, Wei, Williams, Lara, Stant, Exon, Kennett and Malone2004a ). The biostratigraphical ranges of dinoflagellate cyst species are based on the following data: the First Occurrence datum (FO), the First Common Occurrence datum (FCO, >25% of total of dinoflagellate cysts), the Last Occurrence datum (LO) and the Last Common Occurrence datum (LCO, >25% of total of dinoflagellate cysts) (Brinkhuis et al. Reference Brinkhuis, Sengers, Sluijs, Warnaar, Williams, Exon, Kennett and Malone2003b ; Sluijs et al. Reference Sluijs, Brinkhuis, Stickley, Warnaar, Williams, Fuller, Exon, Kennett and Malone2003; Bijl, Sluijs & Brinkhuis, Reference Bijl, Sluijs and Brinkhuis2013b ). Based on records from ODP Leg 189, Sluijs et al. (Reference Sluijs, Brinkhuis, Stickley, Warnaar, Williams, Fuller, Exon, Kennett and Malone2003) suggested three Dinocyst Associations from the middle Eocene (late Bartonian) to the early Oligocene. The authors stated that the replacement of the endemic species by a cosmopolitan association started about 35.5 Ma. Subsequently, Bijl, Sluijs & Brinkhuis (Reference Bijl, Sluijs and Brinkhuis2013b) proposed 13 high-resolution dinoflagellate cyst zones for the South Pacific (South Pacific Dinocyst Zones, SPDZ), from the late Palaeocene to the late Eocene (58–36 Ma) and confirmed that the endemic–Antarctic dinoflagellate cyst assemblage was dominant from the middle to late Eocene (c. 45–35 Ma). Thus, the middle Palaeogene dinoflagellate cyst stratigraphic distribution provides a correlation tool that can be applied to other sites in and around the Southern Ocean.
3. Geological setting
3.a. Austral Basin
The Austral Basin is located in the southwestern part of the South American Plate between 45°S and 54°S and underlies southern Patagonia, the island of Tierra del Fuego and the adjacent Argentine Continental Shelf (Malumián, Reference Malumián and Caminos1999; Nullo, Panza & Blasco, Reference Nullo, Panza, Blasco and Caminos1999). This basin is elongated following a NNW–SSE axis and comprises about 160000 km2. It is bounded by the Andes mountains to the west, the Scotia Plate to the south, and the Deseado Massif and the Río Chico High to the northeast (Fig. 1).
During the Cenozoic, Patagonia was affected by several transgressions from the Atlantic Ocean (Malumián & Nañez, Reference Malumián, Náñez and Haller2011), one of which resulted in the deposition of the upper member of the Río Turbio Formation during the middle to late Eocene in the westernmost part of the Austral Basin (Fig. 1).
3.b. Río Turbio Formation
The Río Turbio Formation (Leanza, Reference Leanza1972) is represented by a thick shallow-marine and estuarine succession, which reaches a thickness of 600 m in the type section (Furque & Caballé, Reference Furque and Caballé1993). The formation is divided into informal lower and upper members (Fig. 2). The lower member, assigned to an early to middle Eocene age (Malumián, Reference Malumián and Haller2002; Guerstein et al. Reference Guerstein, Rodriguez Raising, Casadío, Marenssi and Cárdenas2010b ), overlies a disconformity that erosionally truncates the shallow marine rocks of the Palaeocene Cerro Dorotea Formation. The upper member of the Rio Turbio Formation has been assigned a late–middle Eocene to early–late Eocene age (Malumián et al. Reference Malumian, Panza, Parisi, Nañez, Carames and Torre2000) and is unconformably overlain by the continental rocks of the late Eocene Río Guillermo Formation (Fig. 2) (Malumián & Caramés, Reference Malumián and Caramés1997; Malumián et al. Reference Malumian, Panza, Parisi, Nañez, Carames and Torre2000; Ramos, Reference Ramos2005).

Figure 2. Chart showing correlation of late Palaeocene–Miocene marine formations in the Austral Basin. Correlations are based on foraminifera (Malumián, Reference Malumián and Caminos1999; Malumián & Nañez, Reference Malumián, Náñez and Haller2011) and dinoflagellate cysts (Guerstein et al. Reference Guerstein, Guler, Williams, Fensome and Chiesa2008, Reference González Estebenet, Guerstein and Alperin2014 a).
The upper member of the Rio Turbio Formation is characterized by fine to coarse sandstones and conglomerates with interbedded clay horizons and abundant plant macrofossils that accumulated in coastal marine, wave- and tide-dominated shallow-water environments (Furque & Caballé, Reference Furque and Caballé1993; Rodríguez Raising, Reference Rodríguez Raising2010; Pujana, Martínez & Brea, Reference Pujana, Martínez and Brea2011) or in tide-dominated, outer-estuarine, coastal-plain environments (Pearson et al. Reference Pearson, Mángano, Buatois, Casadío and Rodriguez Raising2012). According to Malumián (Reference Malumián and Haller2002), the upper member of the Rio Turbio Formation contains sedimentation in subtidal environments corresponding to the middle–late Eocene Atlantic transgression, which is characterized by the presence of a glauconitic horizon widespread in the Austral Basin (Calegari, Baldi & Pioli, Reference Calegari, Baldi and Pioli1993). This horizon can be correlated with the following units: the Man Aike Formation in Santa Cruz Province (Casadío et al. Reference Casadío, Griffin, Marenssi, Parras, Rodriguez Raising and Santillana2009; Guerstein et al. Reference Guerstein, González Estebenet, Alperin, Casadío and Archangelsky2014a ); the La Despedida Formation (Guerstein et al. Reference Guerstein, Guler, Williams, Fensome and Chiesa2008); the lower section of the Cerro Colorado Formation and the ‘Glauconitico B’ in Tierra del Fuego Province (Olivero & Malumián, Reference Olivero and Malumián1999); and the Leña Dura Formation and Loreto Formation in Chile (Fasola, Reference Fasola1969; Archangelsky & Fasola, Reference Archangelsky and Fasola1971) (Fig. 2). The first studies on dinoflagellate cysts of the Río Turbio Formation were carried out by Archangelsky (Reference Archangelsky1968, Reference Archangelsky1969) and Archangesky & Fasola (Reference Archangelsky and Fasola1971) in samples recovered from Yacimientos Carboníferos Fiscales (YCF) Cores. Recently, Guerstein et al. (Reference Guerstein, González Estebenet, Alperin, Casadío and Archangelsky2014a), González Estebenet, Guerstein & Rodríguez Raising (Reference González Estebenet, Guerstein and Rodríguez Raising2014) and González Estebenet, Guerstein & Casadío (Reference González Estebenet, Guerstein and Casadío2015) studied the dinoflagellate cyst assemblages from sections cropping out near the type area of the Río Turbio Formation.
The Río Turbio Formation is economically important because it contains the largest coal reserves in Argentina (Malumián, Reference Malumián and Haller2002). Furthermore, the proximity of the Austral Basin to the Drake Passage makes this marine deposit a valuable source of information in evaluating Eocene palaeoclimatic and palaeoenvironmental changes in southern South America. Despite the economic and geographical importance of the Austral Basin, correlations and palaeogeographic reconstructions of the Palaeogene are limited by the availability of biostratigraphic and isotopic data.
4. Materials and methods
4.a. Analysed sections
The studied samples were obtained from three localities: Ea. Cancha Carrera, Yacimientos Carboníferos Fiscales (YCF) Cores and Highway 40, close to Río Turbio town in the southwest of Santa Cruz Province (Fig. 1; Table 1). Twenty-three samples come from the Highway 40 locality, which involved two integrated outcropping sections; 20 samples were taken from the Ea. Cancha Carrera locality, which comprises four outcropping sections integrated into a composite profile; and 21 samples were collected from three YCF cores. Some of the samples from YCF cores were studied originally by Archangelsky (Reference Archangelsky1968, Reference Archangelsky1969) and re-studied for this work. Following Guerstein et al. (Reference Guerstein, González Estebenet, Alperin, Casadío and Archangelsky2014a) we also include in the integrated section two samples from the uppermost part of the Man Aike Formation, an equivalent unit of the upper member of the Río Turbio Formation that crops out near the area where the cores were drilled (Fig. 1).
Table 1. List of samples showing sources and results. We indicate sterile (E) and fertile samples, containing dinoflagellate cysts (D), non-marine palynomorphs (P) and pollen and zygospores (PZ).

4.b. Palynological procedure and microscopical analysis
Samples from the Highway 40 and the Ea. Cancha Carrera localities were processed for palynological analysis using hydrochloric and hydrofluoric acids. The residues were sieved through 10 and 25 μm screens and stained with Bismarck C. Strew mounts were prepared using gelatin–glycerin as mounting medium. The palynological samples were processed at the Museo Argentino de Ciencias Naturales, Bernardino Rivadavia, Buenos Aires (MACN), and the slides are stored at the Laboratorio de Palinología, Instituto Geológico del Sur, Bahía Blanca (LPUNS). Samples from the YCF Cores locality were processed with hydrochloric acid and Schutze reagent for less than two hours (Archangelsky, Reference Archangelsky1968, Reference Archangelsky1969). Recently, some of the residues were sieved, and strew mounts were prepared using gelatin–glycerin as mounting medium. The slides are stored at LPUNS.
Light microscopy was undertaken using a Nikon Eclipse 600 microscope and an attached Micrometrics high-resolution digital camera. The sample number and slide number followed by the England Finder (EF) references are provided for each illustrated specimen in the caption to Figure 3. Dinoflagellate cyst nomenclature, unless otherwise indicated, is based on Fensome, MacRae & Williams (Reference Fensome, MacRae and Williams2008) and Sluijs et al. (Reference Sluijs, Brinkhuis, Williams and Fensome2009). For each sample, a minimum of 200 dinoflagellate cysts were counted and identified at species level.
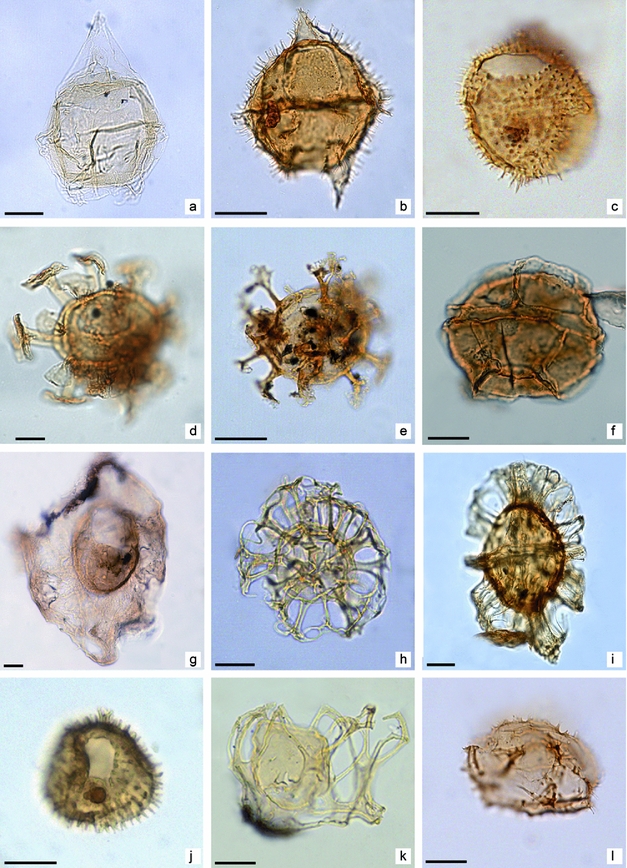
Figure 3. Dinoflagellate cysts from the upper member of the Río Turbio Formation. Specimens are identified by sample number/England Finder references. Scale bar is 20 μm. (a) Deflandrea antarctica; YCF 312/N21-3. (b) Spinidinium macmurdoense; YCF 48/P37-4. (c) Vozzhennikovia apertura; RT 14/1-1/P44-2. (d) Hystrichosphaeridium truswelliae; RT 13/1-1/G40-3. (e) Enneadocysta dictyostila; YCF 113/X39-2. (f) Impagidinium parvireticulatum; RT 15/1-6/R48-1. (g) Thalassiphora pelagica; CC 1/Y43. (h) Nematosphaeropsis sp. A; CC 14/M32-1. (i) Turbiosphaera filosa; YCF 12/Y21-1. (j) Operculodinium sp. CC 10/W24-0. (k) Arachnodinium antarcticum RT 12/1-4/R46-2. (l) Selenopemphix sp. CC 14/J39-1.
The quantitative results of each studied section are presented in a dissociated frequencies diagram indicating the percentages of the total number of dinoflagellate cysts counted (Fig. 4). The percentage of peridinoid cysts (P-cysts) in the dinoflagellate cyst assemblage was used to estimate productivity (Brinkhuis et al. Reference Brinkhuis, Bujak, Smit, Versteegh and Visscher1998; Crouch et al. Reference Crouch, Dickens, Brinkhuis, Aubry, Hollis, Rogers and Visscher2003). Dinoflagellate cyst species were classified as endemic and cosmopolitan, based on Bijl et al. (Reference Bijl, Pross, Warnaar, Stickley, Huber, Guerstein, Houben, Sluijs, Visscher and Brinkhuis2011), Bijl, Sluijs & Brinkhuis (Reference Bijl, Sluijs and Brinkhuis2013b) and Houben et al. (Reference Houben, Bijl, Pross, Bohaty, Passchier, Stickley, Röhl, Sugisaki, Tauxe, Flierdt, Olney, Sangiorgi, Sluijs, Escutia and Brinkhuis2013). The percentage of endemic dinoflagellate cysts over the total number of dinoflagellate cysts was calculated in order to characterize the sea-surface temperature. The number of dinoflagellate cysts / total palynomorphs, expressed as percentage was calculated in order to estimate the proximity to the shoreline. We follow the geological timescale for the Palaeogene by Vandenberghe, Speuer & Hilgen (Reference Vandenberghe, Speijer, Hilgen, Gradstein, Ogg, Schmitz and Ogg2012).

Figure 4. Quantitative distribution of dinoflagellate cysts within samples from the Río Turbio Formation at the sections outcropping at (a) Cancha Carrera; (b) YCF Cores and the Man Aike Formation (samples MA 5 and MA 6); and (c) Highway 40. P-cysts/total: percentages of peridinioid cysts (P-cysts) over total of dinoflagellate cysts; Endemic/total dinocyts: percentages of endemic dinoflagellate cysts over total of dinoflagellate cysts. The bars at the right show the percentages of dinoflagellate cysts over total of palynomorphs. Modified from González Estebenet, Guerstein & Alperin (Reference González Estebenet, Guerstein and Alperin2014), Guerstein et al. (Reference Guerstein, González Estebenet, Alperin, Casadío and Archangelsky2014a) and González Estebenet et al. (Reference González Estebenet, Guerstein and Casadío2015).
5. Sections
This work integrates 52 samples analysed from the upper member of the Río Turbio Formation and two samples from the upper part from the Man Aike Formation outcropping near the YCF Cores. All the samples bear assemblages rich in dinoflagellate cysts; four of them contain only non-marine palynomorphs (mainly pollen, spores and zygospores of fresh-water green algae), and eight were barren of palynomorphs (Table 1). Table 2 lists a total of 36 species recovered including their biogeographical distribution (endemic or cosmopolitan), most of which are illustrated in Figure 3. Figure 4a–c plots the quantitative composition of the dinoflagellate cyst assemblages through each studied section. The dinoflagellate cyst events recorded in our sections and their comparison with their biostratigraphical ranges based on calibrated data from the ODP Leg 189 cores are shown in Figure 5.
Table 2. List of species of dinoflagellate cysts cited in the text.

The latitudinal distribution is based on Bijl et al. (Reference Bijl, Pross, Warnaar, Stickley, Huber, Guerstein, Houben, Sluijs, Visscher and Brinkhuis2011), Bijl, Sluis & Brinkhuis, (Reference Bijl, Sluijs and Brinkhuis2013 b) and Houben et al. (Reference Houben, Bijl, Pross, Bohaty, Passchier, Stickley, Röhl, Sugisaki, Tauxe, Flierdt, Olney, Sangiorgi, Sluijs, Escutia and Brinkhuis2013). The localities where the species are present are indicated with asterisk (*).Or: orden; G: Gonyaulacales; P: Peridiniales; LD: latitudinal distribution; C: cosmopolitan distribution; E: endemic–Antarctic distribution; CC: Ea. Cancha Carrera locality; YCF: Yacimientos Carboníferos Fiscales Cores locality; HN: Highway 40 locality.

Figure 5. Dinoflagellate cyst events and zones recorded in the upper member of the Río Turbio Formation compared and correlated with those recorded in ODP cores from South Pacific Ocean. DA: Dinocyst Association (Sluijs et al. Reference Sluijs, Brinkhuis, Stickley, Warnaar, Williams, Fuller, Exon, Kennett and Malone2003); SPDZ: South Pacific Dinocyst Zones (Bijl, Sluijs & Brinkhuis,. Reference Bijl, Sluijs and Brinkhuis2013b ); MAF: Man Aike Formation (Guerstein et al. Reference Guerstein, González Estebenet, Alperin, Casadío and Archangelsky2014a ); RTF: dinoflagellate cyst zones of the upper member of the Río Turbio Formation; Enne: Enneadocysta; Vozzh: Vozzhennikovia; S. macm: Spinidinium macmurdoense.
5.a. Cancha Carrera
The stratigraphic framework for the composed Cancha Carrera section is based on Rodríguez Raising (Reference Rodríguez Raising2010), and the dinoflagelate cysts assemblages were studied by González Estebenet, Guerstein & Casadío (Reference González Estebenet, Guerstein and Casadío2015).
From the base to 286 m high correspond to the lower member of the Río Turbio Formation (Rodriguez Raising et al. Reference Rodríguez Raising, Griffin, Marenssi and Casadío2008). The interval from 286 m to the top of the section (774 m) represents the upper member of the formation.The dinoflagellate cyst assemblages from 286 to 290 m high, measured from the base of the section, (samples CC 1 to CC 2) show different dinoflagellate cysts typical of the endemic–Antarctic association (Deflandrea antarctica, Enneadocysta dictyostila and Vozzhennikovia apertura) (Fig. 4a). The base of this interval is defined by the FO of Enneadocysta dictyostila, which has not been recorded in the lower member of the Río Turbio Formation (Guerstein et al. Reference Guerstein, Rodriguez Raising, Casadío, Marenssi and Cárdenas2010b ), and the presence of Hystrichosphaeridium tubiferum, with its LO recorded in the sample CC 2. In research conducted in the South Pacific Ocean, Brinkhuis et al. (Reference Brinkhuis, Sengers, Sluijs, Warnaar, Williams, Exon, Kennett and Malone2003b ) and Bijl, Sluijs & Brinkhuis (Reference Bijl, Sluijs and Brinkhuis2013b) established the FO of Enneadocysta dictyostila at the Magnetochron C20r (c. 45.5 Ma). Williams et al. (Reference Williams, Brinkhuis, Pearce, Fensome, Weegink, Exon, Kennett and Malone2004) recorded the LO of Hystrichosphaeridium tubiferum at the top of Magnetochron C21n, at about 45.6 Ma according to Vandenberghe, Speuer & Hilgen (Reference Vandenberghe, Speijer, Hilgen, Gradstein, Ogg, Schmitz and Ogg2012). The coexistence of both species constrains the age of this part of the section to the middle Lutetian. Sample CC3 (at 352 m from the base of the section) shows the FCO of Enneadocysta dictyostila dated at 45.2 Ma (middle Lutetian) by Bijl, Sluijs & Brinkhuis (Reference Bijl, Sluijs and Brinkhuis2013b). In sample CC 6, at 452 m from the base of the section, the presence of Hystrichosphaeridium truswelliae allows us to propose a minimum age of this stratigraphic part of the Cancha Carrera section of the middle Bartonian (or early Priabonian). Brinkhuis et al. (Reference Brinkhuis, Sengers, Sluijs, Warnaar, Williams, Exon, Kennett and Malone2003b ) recorded the LO of this species at the Magnetochron C18n1n, at c. 38.6 Ma.
In the middle part of the section (493–558 m from the base; samples CC 7 to CC 10) the abundance of Enneadocysta dictyostila is replaced by Vozzhennikovia apertura with maximum abundances throughout this stratigraphic interval. Acmes of this species in the South Pacific Ocean were recognized as part of a complex of species, Vozzhennikovia spp., between 50 Ma and 33.5 Ma (Bijl, Sluijs & Brinkhuis, Reference Bijl, Sluijs and Brinkhuis2013b ).
Towards the top of the section (617–670 m above the base; samples CC 11 to CC 14) the endemic–Antarctic assemblages are replaced by species with cosmopolitan distribution such as Turbiosphaera filosa, Protoperidiniaceae (species of Selenopemphix and Lejeunecysta) together with Impagidinium dispertitum, Operculodinium spp., Spiniferites spp. (mostly S. pseudofurcatum) and Nematosphaeropsis sp. A. Sluijs et al. (Reference Sluijs, Brinkhuis, Stickley, Warnaar, Williams, Fuller, Exon, Kennett and Malone2003), Stickley et al. (Reference Stickley, Brinkhuis, Schellenberg, Sluijs, Röhl, Fuller, Grauert, Huber, Warnaar and Williams2004b) and Houben et al. (Reference Houben, Bijl, Pross, Bohaty, Passchier, Stickley, Röhl, Sugisaki, Tauxe, Flierdt, Olney, Sangiorgi, Sluijs, Escutia and Brinkhuis2013) related the replacement of cosmopolitan species by endemic taxa with the beginning of the deepening of the Tasmanian Gateway, dated through magneto-stratigraphy at c. 35.5 Ma (Priabonian). The maximum age proposed agrees with the FCO of Turbiosphaera filosa at the base of the uppermost stratigraphic interval, recorded by Sluijs et al. (Reference Sluijs, Brinkhuis, Stickley, Warnaar, Williams, Fuller, Exon, Kennett and Malone2003) at 35.5 Ma. Turbiosphaera filosa is abundant throughout the interval, with its LO in sample CC 14 (667 m). This event was recorded at Magnetochron C13r, at c. 34 Ma in the South Pacific Ocean (Brinkhuis et al. Reference Brinkhuis, Sengers, Sluijs, Warnaar, Williams, Exon, Kennett and Malone2003b ).
5.b. YCF Cores
Guerstein et al. (Reference Guerstein, González Estebenet, Alperin, Casadío and Archangelsky2014a) compared the samples of YCF Cores and some samples from the Highwat 40 section with those assigned to the Man Aike Formation outcropping in the same area. These authors determined the equivalence of the dinoflagellate cyst assemblages from the uppermost part of the Man Aike Formation with those from the lower part of the YCF Cores and the lower part of Highway 40. Figure 4b shows the dinoflagellate cyst quantitative distribution data from the YCF Cores and the Man Aike Formation in an integrated section.
The assemblages from the lower and middle parts of the integrated section (including 11 samples between 346 and 280 m depth and the two samples from the Man Aike Formation) are dominated by Enneadocysta dictyostila, indicating an age for this stratigraphic interval younger than 45.2 Ma, based on Bijl, Sluijs & Brinkhuis (Reference Bijl, Sluijs and Brinkhuis2013b). The dinoflagellate cyst events recognized in this part of the integrated section are the LOs of Arachnodinium antarcticum and Hystrychosphaeridium truswelliae. Both events restrict the maximum age of this stratigraphic interval to the middle Bartonian (Fig. 5). Moreover, dinoflagellate cysts together with calcareous microfossil biostratigraphy, mollusc affinities and the 87Sr/86Sr data constrain the age of the Man Aike Formation and the lower part of the YCF Cores section to c. 42 to 39 Ma (Guerstein et al. Reference Guerstein, González Estebenet, Alperin, Casadío and Archangelsky2014a ).
A significant dinoflagellate cyst replacement is observed in the assemblages between 270 and 12 m depth, including nine samples. The dinoflagellate cyst assemblages are dominated by Vozzhennikovia apertura and Spinidinium macmurdoense, both members of the endemic–Antarctic assemblage, together with species included in the Operculodinium spp. and Turbiosphaera complex (Fig. 4). The FCO of Spinidinium macmurdoense is recorded in the South Pacific Ocean at c. 37 Ma, suggesting that this part of the integrated core section cannot be older than Priabonian. The LCO of Spinidinium macmurdoense determined at 33.5 Ma by Brinkhuis et al. (Reference Brinkhuis, Sengers, Sluijs, Warnaar, Williams, Exon, Kennett and Malone2003b ) allows us to propose an age no younger than late Priabonian for the entire analysed section. It is noticeable that the uppermost stratigraphic interval has no record of Enneadocysta dictyostila, Deflandrea antarctica or Thalassiphora pelagica.
5.c. Highway 40
The stratigraphic framework for the study of this section is based on Rodríguez Raising (Reference Rodríguez Raising2010) and Rodríguez Raising et al. (Reference Rodríguez Raising, Casadío, Pearson, Mangano, Buatois and Griffin2014). The results of the dinoflagellate cyst analysis from two integrated sections were presented in González Estebenet, Guerstein & Rodríguez Raising (Reference González Estebenet, Guerstein and Rodríguez Raising2014) and are summarized in Figure 4c. Almost the complete section from the base to 135 m high, represented by 17 productive palynological samples (from RT 12/1-1 to RT 15/1-6), shows an alternation of assemblages dominated by Enneadocysta dictyostila and those with high abundances of Vozzhennikovia apertura. Enneadocysta dictyostila is abundant from the base of the section, where the FCO is determined at 45.2 Ma by Bijl, Sluijs & Brinkhuis (Reference Bijl, Sluijs and Brinkhuis2013b). Moreover, as mentioned for YCF Cores, the base of the Highway 40 section can be defined at 42 Ma due to its correlation with the Man Aike Formation (Guerstein et al. Reference Guerstein, González Estebenet, Alperin, Casadío and Archangelsky2014a ). The most significant dinoflagellate cyst events recognized in the samples RT 13/1-1 to RT 13/1-3 (35– 39 m from the base of the section) are the LOs of Arachnodinium antarcticum and Hystrychosphaeridium truswelliae. According to Brinkhuis et al. (Reference Brinkhuis, Munsterman, Sengers, Sluijs, Wanaar, Williams, Exon, Kennett and Malone2003b ), both species presented their LOs at 38.6 Ma. These events allow us to establish a late Bartonian age for the middle part of the Highway 40 section. The RT 15/1-5 sample contains the uppermost assemblage with a high proportion of Enneadocysta dictyostila. Impagidinium parvireticulatum is dominant in the RT 15/1-5 and RT 15/1-6 samples. The FO of Impagidinium parvireticulatum is defined at 44 Ma by Bijl, Sluijs & Brinkhuis (Reference Bijl, Sluijs and Brinkhuis2013b). These authors defined the LO of Impagidinium parvireticulatum and the LCO of Enneadocysta dictyostila at 35.5 Ma. Towards the top of the section (RT-15-1-7 and RT 15-1-8) the dinoflagellate cyst assemblages are characterized by Vozzhennikovia apertura as the unique species.
6. Zonation
The stratigraphic sections are placed along a N–S transect c. 120 km long. Therefore, bearing in mind the size of the basin, we can asume that the dinoflagellate cyst events are nearly synchronic among the three localities. Considering the dinoflagellate cyst quantitative distribution and their biostratigraphic events we are able to correlate the three analysed sections and propose four Dinoflagellate Cyst Zones, labelled RTF 1 to RTF 4, for the middle to upper Eocene in the Austral Basin (Fig. 5). We determine the top of each zone by dinoflagellate cyst events that also state the base of the overlying zone. A type section with a base and top samples is assigned for each dinoflagellate cyst zone. Where possible, we correlate these events and assemblage shifts with those in other sections from the Austral Basin. Finally, we compare our dinoflagellate cyst zones with the middle–late Eocene South Pacific Dinocyst Zones (Bijl, Sluijs & Brinkhuis, Reference Bijl, Sluijs and Brinkhuis2013b ) and the middle–late Eocene to late Eocene dinoflagellate cyst associations described by Sluijs et al. (Reference Sluijs, Brinkhuis, Stickley, Warnaar, Williams, Fuller, Exon, Kennett and Malone2003).
6.a. Zone RTF 1
Definition. The base of this zone is marked by the FO of Enneadocysta dictyostila and the LO of Hystrichosphaeridium tubiferum; the top of the zone is defined by the FCO of Enneadocysta dictyostila.
Type locality. Cancha Carrera section. Base sample: CC 1 (286 m from the base of the section). Top sample: CC 2 (290 m from the base of the section).
Characteristic species. Deflandrea antarctica, Deflandrea granulata, Enneadocysta dictyostila, Vozzhennikovia apertura, Thalassiphora pelagica, Hystrichosphaeridium tubiferum, Spiniferites ramosus.
Age. Middle Lutetian (c. 46 Ma).
Correlation. Zone RTF 1 was not recognized in the sections along Highway 40 or in the YCF Cores. This zone can be correlated with the base of La Despedida Fm., Tierra del Fuego Province (Guerstein et al. Reference Guerstein, Guler, Williams, Fensome and Chiesa2008), and with the Leña Dura Formation in Chile (Cookson & Cranwell, Reference Cookson and Cranwell1967). Both formations, and likewise Zone RTF 1, show the co-dominance of Deflandrea antarctica and Enneadocysta dictyostila. Within the Austral Ocean realm, Zone RTF 1 can be correlated with the Zone SPDZ10 (middle Lutetian, 46.2– 45.2 Ma) proposed by Bijl, Sluijs & Brinkhuis (Reference Bijl, Sluijs and Brinkhuis2013b). The authors defined the Zone SPDZ10 by dinoflagellate cyst assemblages alternating between Deflandrea antarctica, Enneadocysta multicornuta and Enneadocysta dictyostila, and with the FO of Enneadocysta dictyostila as a significative event.
6.b. Zone RTF 2
Definition. The base of this zone is marked by the FCO of Enneadocysta dictyostila; the top of the zone is defined by the LOs of Arachnodinium antarcticum and Hystrichosphaeridium truswelliae.
Type locality. Cancha Carrera section. Base sample: CC 2 (290 m from the base of the integrated section); top sample: CC 6 (452 m from the base of the integrated section).
Characteristic species. Enneadocysta dictyostila, Vozzhennikovia apertura, Deflandrea antarctica, Arachnodinium antarcticum, Hystrichosphaeridium truswelliae, Achomosphaera sp. A.
Age. Middle Lutetian (46 Ma) to late Bartonian (39 Ma).
Correlation. Zone RTF 2 is recognized along the Highway 40 section, from the base up to 42 m from the base of the section, and in the YCF Cores, from 346 to 280 m depth. This zone is characterized by the dominance of Enneadocysta dictyostila in most of the dinoflagellate cyst assemblages. Guerstein et al. (Reference Guerstein, González Estebenet, Alperin, Casadío and Archangelsky2014a) proposed an age between 42 and 39 Ma for the Man Aike Formation and the equivalent parts of the Río Turbio Formation that represent only the upper part of Zone RTF 2 (Fig. 5). Thus, the lower part of Zone RTF 2 is merely represented in the type section (Cancha Carrera locality), as shown in Figure 5. The age of this stratigraphic interval cannot be precisely determined. The high abundances of Enneadocysta dictyostila allow us to correlate this zone with the upper part of La Despedida Formation in Tierra del Fuego Province (Guerstein et al. Reference Guerstein, Guler, Williams, Fensome and Chiesa2008). The base of Zone RTF 2 can be considered equivalent to the base of Zone SPDZ11 (Bijl, Sluijs & Brinkhuis, Reference Bijl, Sluijs and Brinkhuis2013b ), both defined by the FCO of Enneadocysta dictyostila. Considering the age range and the characteristic species included in the upper part of the RTF 2 it can be correlated with the SPDZ12 of Bijl, Sluijs & Brinkhuis (Reference Bijl, Sluijs and Brinkhuis2013b).
Remarks. Bijl et al. (Reference Bijl, Houben, Schouten, Bohaty, Sluijs, Reichart, Damsté and Brinkhuis2010) studied a sedimentary record spanning the Middle Eocene Climate Optimum (MECO) recovered from ODP Site 1172. Subsequently, Bijl, Sluijs & Brinkhuis (Reference Bijl, Sluijs and Brinkhuis2013b) considered that the MECO was found at the top of the SPDZ12. Thus, this global hyperthermal episode lasting c. 500000-years-, and dated at c. 40 Ma (Bohaty & Zachos, Reference Bohaty and Zachos2003; Bohaty et al. Reference Bohaty, Zachos, Florindo and Delaney2009), may be included in the upper part of the RTF 2 recognized in the three sections analysed in this study.
6.c. Zone RTF 3
Definition. The base of this zone is defined by the LOs of Arachnodinium antarcticum and Hystrichosphaeridium truswelliae. The top corresponds to the FCO of Turbiosphaera filosa and the LCO of the species of the endemic–Antarctic assemblage.
Type locality. Cancha Carrera section. Base sample: CC 6 (452 m from the base of the integrated section); top sample: CC 10 (558 m from the base of the integrated section).
Characteristic species. In the type section the assemblages are highly dominated by Vozzhennikovia apertura. The palynological recovery and the dinoflagellate cyst event in this zone are hampered by the scarcity of fine clastic horizons.
Age. Late Bartonian (39 Ma) to middle Priabonian (36 Ma).
Correlation. Zone RTF 3 has been recognized in Highway 40 (74–183 m from the base of the integrated section) and in the YCF Cores (12–270 m depth). In the Highway 40 section the LO of Impagidinium parvireticulatum and the LCO of Enneadocysta dictyostila are two significant events dated at the middle Priabonian (Bijl, Sluijs & Brinkhuis, Reference Bijl, Sluijs and Brinkhuis2013b ). In the YCF Cores two significant events are the FCO and the LCO of Spinidinium macmurdoense. The FCO of Spinidinium macmurdoense allows us to correlate with SPDZ 13 of Bijl, Sluijs & Brinkhuis (Reference Bijl, Sluijs and Brinkhuis2013b), and its LCO is a remarkable event recorded in the South Pacific Ocean at c. 36 Ma (Sluijs et al. Reference Sluijs, Brinkhuis, Stickley, Warnaar, Williams, Fuller, Exon, Kennett and Malone2003). Abundance of Vozzhennikovia apertura and the FCO of Spinidinium macmurdoense are significant bioevents of the dinoflagellate cyst Zone DA 1 of Sluijs et al. (Reference Sluijs, Brinkhuis, Stickley, Warnaar, Williams, Fuller, Exon, Kennett and Malone2003). This composition is also observed in the dinoflagellate cyst assemblages of the Loreto Formation in Chile (Archangelsky & Fasola, Reference Archangelsky and Fasola1971).
Remarks. The upper part of Zone RTF 3 in the three sections is characterized by the presence of a coal seam recorded both by Archangelsky (Reference Archangelsky1969) and Rodríguez Raising (Reference Rodríguez Raising2010).
6.d. Zone RTF 4
Definition. The base of this zone is defined by the FCO of Turbiosphaera filosa and the LCO of the species of the endemic–Antarctic assemblage. The top is determined by the LO of Turbiosphaera filosa.
Type locality. Cancha Carrera section. Base sample: CC 10 (558 m from the base of the integrated section); top sample: CC 14 (667 m from the base of the section).
Characteristic species. Turbiosphaera filosa, species of Selenopemphix and Lejeunecysta, Operculodinium centrocarpum / israelianum spp., Impagidinium dispertitum, Spiniferites spp. and Lingulodinium machaerophorum. The assemblages from the base of this zone have only a few specimens of the endemic species Vozzhennikovia apertura.
Age. Middle to late Priabonian (c. 35.5–33.5 Ma).
Correlation. The RTF 4 has not been recognized along the Highway 40 sections or YCF Cores. The FCO of Turbiosphaera filosa is one of the events delimiting the base of Zone DA 2 (c. 35.5–33.5 Ma) in the South Pacific Ocean (Sluijs et al. Reference Sluijs, Brinkhuis, Stickley, Warnaar, Williams, Fuller, Exon, Kennett and Malone2003). According to Sluijs et al. (Reference Sluijs, Brinkhuis, Stickley, Warnaar, Williams, Fuller, Exon, Kennett and Malone2003), Zone DA 2 is a transition from assemblages dominated by endemic–Antarctic species to assemblages dominated by protoperidiniaceae and other cosmopolitan taxa.
Remarks. Sluijs et al. (Reference Sluijs, Brinkhuis, Stickley, Warnaar, Williams, Fuller, Exon, Kennett and Malone2003), Stickley et al. (Reference Stickley, Brinkhuis, Schellenberg, Sluijs, Röhl, Fuller, Grauert, Huber, Warnaar and Williams2004b) and Houben et al. (Reference Houben, Bijl, Pross, Bohaty, Passchier, Stickley, Röhl, Sugisaki, Tauxe, Flierdt, Olney, Sangiorgi, Sluijs, Escutia and Brinkhuis2013) related the replacement of endemic taxa by cosmopolitan species to the beginning of the deepening of the Tasmanian Gateway, dated through magneto-stratigraphy at c. 35.5 Ma (Priabonian).
7. Palaeoenvironmental evolution during the middle and late Eocene
In the biostratigraphic scheme defined above for the upper member of the Río Turbio Formation, palaeoenvironmental changes can be recognized based on the palaeoecological preferences of several dinoflagellate cyst taxa (Table 3).
Table 3. List of distinctive species of the upper member of the Río Turbio Formation and its significance as palaeoenvironmental indicators.

M: monospecific; A: abundant; P: presence.
Zones RTF 1 to RTF 3 show the dominance of species of the endemic–Antarctic assemblage. The changes in the abundance of Enneadocysta dictyostila versus Vozzhennikovia apertura – Spinidinium macmurdoense – Deflandrea antarctica have been mentioned by Röhl et al. (Reference Röhl, Brinkhuis, Stickley, Fuller, Schellenberg, Wefer, Williams, Exon, Malone and Kennett2004) and Sluijs, Pross & Brinkhuis (Reference Sluijs, Pross and Brinkhuis2005) as a characteristic alternation of the middle to late Eocene at high latitudes of the Southern Hemisphere. The high percentages of Enneadocysta dictyostila related to neritic sediments rich in CaCO3 suggest the presence of warm surface waters and offshore settings (Röhl et al. Reference Röhl, Brinkhuis, Stickley, Fuller, Schellenberg, Wefer, Williams, Exon, Malone and Kennett2004; Sluijs, Pross & Brinkhuis, Reference Sluijs, Pross and Brinkhuis2005; Guerstein et al. Reference Guerstein, Guler, Brinkhuis, Warnaar, Madden, Carlini, Vucetich and Kay2010a ). On the other hand, species of Deflandrea, Vozzhennikovia and Spinidinium, corresponding to more CaCO3-depleted sediments, indicate coastal environments characterized by surface temperate waters with high dissolved nutrient availability (Röhl et al. Reference Röhl, Brinkhuis, Stickley, Fuller, Schellenberg, Wefer, Williams, Exon, Malone and Kennett2004; Pross & Brinkhuis, Reference Pross and Brinkhuis2005; Sluijs, Pross & Brinkhuis, Reference Sluijs, Pross and Brinkhuis2005; Warnaar et al. Reference Warnaar, Bijl, Huber, Sloan, Brinkhuis, Röhl, Sriver and Visscher2009), possibly related to continental freshwater inputs (González Estebenet, Guerstein & Rodríguez Raising Reference González Estebenet, Guerstein and Rodríguez Raising2014; Rodríguez Raising et al. Reference Rodríguez Raising, Casadío, Pearson, Mangano, Buatois and Griffin2014).
The age determined for the upper part of Zone RTF 2 (~42 and 39 Ma) suggests that it encompasses the Middle Eocene Climate Optimum (MECO). Zone RTF 2 is characterized by an increase of Enneadocysta dictyostila, a warm-water-tolerant species. This assumption is consistent with a significant representation of Enneadocysta dictyostila in samples from the Colorado (~38°S), Punta del Este (~36°S) and Pelotas basins (Guerstein & Daners, Reference Guerstein and Daners2010; Premaor et al. Reference Premaor, Souza, Ferreira and Guerstein2013; Guerstein et al. Reference Guerstein, González Estebenet, Daners, Premaor, Pedrão Ferreira and Belgaburo2014b ). However, higher-resolution studies are required in order to more closely constrain the part of the upper member of the Río Turbio Formation containing the MECO.
In Zone RTF 4, the endemic middle Eocene dinoflagellate cyst taxa are largely replaced by cosmopolitan species. There are species of Turbiosphaera filosa characterized by well-developed processes, suggesting normal marine conditions (González Estebenet, Guerstein & Casadío, Reference González Estebenet, Guerstein and Casadío2015; Table 3). This zone also shows higher proportions of protoperidiniaceans associated with nutrient-rich surface waters (Pross & Brinkhuis, Reference Pross and Brinkhuis2005; Zonneveld, Susek & Fischer, Reference Zonneveld, Susek and Fischer2010; Zonneveld et al. Reference Zonneveld, Marret, Versteegh, Bogus, Bonnet, Bouimetarhan, Crouch, de Vernal, Elshanawany, Edwards, Esper, Forke, Grøsfjeld, Henry, Holzwarth, Kielt, Kim, Ladouceur, Ledu, Chen, Limoges, Londeix, Lu, Mahmoud, Marino, Matsouka, Matthiessen, Mildenhal, Mudie, Neil, Pospelova, Qi, Radi, Richerol, Rochon, Sangiorgi, Solignac, Turon, Verleye, Wang, Wang and Young2013), and the number of endemic peridinoids is considerably reduced. Gonyaulacoids such as Impagidinium and Nematosphaeropsis, which are typical of outer neritic and oceanic environments (Dale, Reference Dale, Jansonius and McGregor1996; Marret & Zonneveld, Reference Marret and Zonneveld2003), occur together with Operculodinium spp., and Lingulodinium machaerophorum, which today are distributed in inner to outer neritic environments (Pross & Brinkhuis, Reference Pross and Brinkhuis2005). Hence, the top of the upper member of the Río Turbio Formation (Zone RTF 4) could have been deposited in an outer-shelf setting that was subject to upwelling processes, leading to high nutrient concentrations in surface waters. Upwelling episodes in subsurface waters on the modern continental shelf off Argentina associated with the northward-flowing Malvinas Current on the continental slope have been described by numerical ocean-circulation modelling of the southwestern Atlantic Ocean (Matano & Palma, Reference Matano and Palma2008; Palma, Matano & Piola, Reference Palma, Matano and Piola2008; Combes & Matano, Reference Combes and Matano2014) and hydrographic observations (Piola et al. Reference Piola, Martinez Avellaneda, Guerrero, Jardon, Palma and Romero2010).
The decrease in endemic species in high southern latitudes and the advance and evolution of modern cosmopolitan taxa could be a consequence of the deepening of the Tasman Gateway and the Drake Passage (Sluijs et al. Reference Sluijs, Brinkhuis, Stickley, Warnaar, Williams, Fuller, Exon, Kennett and Malone2003; Stickley et al. Reference Stickley, Brinkhuis, Schellenberg, Sluijs, Röhl, Fuller, Grauert, Huber, Warnaar and Williams2004b ; Guerstein et al. Reference Guerstein, Guler, Williams, Fensome and Chiesa2008; Houben et al. Reference Houben, Bijl, Guerstein, Sluijs and Brinkhuis2011, Reference Houben, Bijl, Pross, Bohaty, Passchier, Stickley, Röhl, Sugisaki, Tauxe, Flierdt, Olney, Sangiorgi, Sluijs, Escutia and Brinkhuis2013). These changes would have led to the development of an unconstrained current (the proto- Circum Antarctic Current) around Antarctica in the earliest Oligocene. In turn, such modifications in the surface oceanic circulation patterns would have disrupted the subpolar gyres that originated during the middle Eocene, leading to the extinction of endemic species and the arrival and installation of cosmopolitan taxa (Huber et al. Reference Huber, Brinkhuis, Stickley, Döös, Sluijs, Warnaar, Schellenberg and Williams2004; Guerstein et al. Reference Guerstein, Guler, Brinkhuis, Warnaar, Madden, Carlini, Vucetich and Kay2010a ).
8. Conclusions
The dinoflagellate cyst events that we recorded in the upper member of the Río Turbio Formation were compared with the biostratigraphic ranges published by Brinkhuis et al. (Reference Brinkhuis, Sengers, Sluijs, Warnaar, Williams, Exon, Kennett and Malone2003b ), Sluijs et al. (Reference Sluijs, Brinkhuis, Stickley, Warnaar, Williams, Fuller, Exon, Kennett and Malone2003) and Bijl, Sluijs & Brinkhuis (Reference Bijl, Sluijs and Brinkhuis2013b) for the South Pacific Ocean. Based on these comparisons it is possible to determine an age ranging from 46 Ma (mid-Lutetian) to 33.5 Ma (late Priabonian) for the upper member of the Rio Turbio Fm.
We have recognized four dinoflagellate cyst zones, RTF 1 to RTF 4 dated as middle Lutetian (ca. 46 Ma); middle Lutetian (~45.2 Ma) to late Bartonian (39 Ma); late Bartonian (39 Ma) to middle Priabonian (36 Ma); and middle to late Priabonian (c. 35.5–33.5 Ma), respectively. The dinoflagellate cyst zones recorded in the upper member of the Río Turbio Formation can be correlated with Zone SPDZ 10 to Zone SPDZ 13 as determined by Bijl, Sluijs & Brinkhuis (Reference Bijl, Sluijs and Brinkhuis2013b), and with DA 1 to DA 2 as defined by Sluijs et al. (Reference Sluijs, Brinkhuis, Stickley, Warnaar, Williams, Fuller, Exon, Kennett and Malone2003).
Alternating abundances of the gonyaulacoid Enneadocysta dictyostila and the extinct peridinioids Deflandrea Antarctica, Vozzhennikovia apertura and Spinidinium macmurdoense in Zones RTF 1 to RTF 3 indicate changes within an inner–outer neritic palaeoenvironment. High abundances of Enneadocysta dictyostila characterize offshore settings and low nutrient availability, whereas high proportions of the extinct peridinioids can be related to shallow marine palaeoenvironments and enhanced nutrient availability, likely related to fluvial discharges.
The endemic–Antarctic species are abundant in the assemblages from zones RTF 1 to RTF 3, while Zone RTF 4 shows a marked replacement of endemic species by cosmopolitan taxa. These assemblages are dominated by T. filosa, protoperidinaceans, and species of Impagidinium and Nematosphaeropsis, possibly related to an oceanic environment influenced by upwelling. This turnover in the dinoflagellate cyst assemblages seems to have been forced by the deepening of the Drake Passage and the Tasman Gateway, and changes in the global ocean circulation patterns (Sluijs et al. Reference Sluijs, Brinkhuis, Stickley, Warnaar, Williams, Fuller, Exon, Kennett and Malone2003; Stickley et al. Reference Stickley, Brinkhuis, Schellenberg, Sluijs, Röhl, Fuller, Grauert, Huber, Warnaar and Williams2004b ; Guerstein et al. Reference Guerstein, Guler, Williams, Fensome and Chiesa2008; Houben et al. Reference Houben, Bijl, Guerstein, Sluijs and Brinkhuis2011, Reference Houben, Bijl, Pross, Bohaty, Passchier, Stickley, Röhl, Sugisaki, Tauxe, Flierdt, Olney, Sangiorgi, Sluijs, Escutia and Brinkhuis2013; González Estebenet, Guerstein & Alperin, Reference González Estebenet, Guerstein and Alperin2014).
Acknowledgements
The authors thank O. Cárdenas for palynological technical assistance, and J. Cornago for improving the language of the manuscript before submission. Rob Fensome and Peter Bijl are thanked for useful comments and suggestions that led to significant improvements to the new version of the manuscript. This study was funded by grants from the Agencia Nacional de Promoción Científica y Tecnológica (PICT 89/09), from Consejo Nacional de Investigaciones Científicas y Técnicas (PIP: 112-201101-00566) and from Universidad Nacional del Sur (PGI 24/H125).