1. Introduction
Northward movement of Baltica and Avalonia continents in early Palaeozoic times reached collision stage with Laurentia in Silurian times (Cocks & Torsvik, Reference Cocks and Torsvik2005). This process caused intensive volcanism in the closing Iapetus Ocean between these continents. Silurian-age igneous rocks are known in the Norwegian Caledonides (Corfu et al. Reference Corfu, Torsvik, Andersen, Ashwal, Ramsay and Roberts2006), but also in Central Europe (Timmerman, Reference Timmerman and McCann2008). Sedimentary rocks of Silurian age crop out in Central Estonia and the Baltic Sea islands Saaremaa and Gotland. Fragmentary outcrop areas occur throughout southern-central Sweden and the Oslo Region in Norway. A large continuous subsurface distribution area of Silurian sedimentary rocks occurs from southern Estonia through Latvia and Lithuania to the northeastern part of Poland. Overviews of the occurrence of Silurian altered volcanic ash beds (K-bentonites, bentonites) around the Iapetus palaeo-ocean were published in Bergström et al. (Reference Bergström, Huff, Kolata and Kaljo1992) and Huff, Bergström & Kolata (Reference Huff, Bergström and Kolata2002).
Studies so far of Wenlock bentonites in northern Europe include Cave & Loydell (Reference Cave and Loydell1998), Batchelor & Jeppsson (Reference Batchelor and Jeppsson1999), Kiipli & Kallaste (Reference Kiipli and Kallaste2006), Ray (Reference Ray2007) and Kiipli et al. (Reference Kiipli, Jeppsson, Kallaste and Söderlund2008a, Reference Kiipli, Radzevičius, Kallaste, Motuza, Jeppsson and Wicksrömc). Due to the large number of volcanic eruption layers, and small number of studied sections, data on Wenlock bentonites are still fragmentary and far from satisfactory for reliable correlation of sections using bentonites. Here we present new results from a geochemical study of 37 bentonite layers sampled from the lower and middle Wenlock of the Ventspils D3 and Vidale 263 drill cores (Latvia) and compare these new data with earlier tephrostratigraphic and geochemical studies in the region. The aim of the present study is a geochemical characterization of lower to middle Wenlock bentonites in the East Baltic and production of a new, more complete list of volcanic ash horizons, that can be used for correlations in future studies in wider areas in northern Europe.
Aspects of the biostratigraphy the Ohesaare core have been published by Kaljo (Reference Kaljo1970) and Loydell, Kaljo & Männik (Reference Loydell, Kaljo and Männik1998), the Ventspils D3 core by Gailite, Ulst & Jakovleva (Reference Gailite, Ulst and Jakovleva1987) and the Ruhnu 500 core by Põldvere (Reference Põldvere2003). The chitinozoans of these cores have been studied by Nestor (Reference Nestor1994). The biostratigraphy of the Vidale 263 core has not been previously published.
2. Material and methods
Thirty-seven thin bentonites varying in thickness from 0.1 to 6.0 cm (online Appendix at http//journals.cambridge.org/geo) were collected from the Ventspils D3 and Vidale 263 cores drilled in West Latvia (Fig. 1). These cores are stored in the Latvian Agency of Environment, Meteorology and Geology. While the host shales are grey, the altered volcanic ashes can be recognized by their yellowish or bluish-grey colour. Biotite phenocrysts visible to the naked eye often confirm the volcanic origin of the bentonites. Very thin volcanic ash beds were sampled together with the host shale, and separation was performed in the laboratory by picking out fragments of different colours. In these cases both volcanic ashes and host shales were analysed.

Figure 1. (a) Location of drill holes. Grey rings – sections studied in this work; black rings – sections studied in Kiipli & Kallaste (Reference Kiipli and Kallaste2006). Wider area (b) with location of studied region. Sketch of Wenlock palaeogeography (c) according to Cave & Loydell (Reference Cave and Loydell1998) showing colliding Baltica and Laurentia with occurrences of Wenlock age K-bentonites, massive volcanics and igneous rocks of Wenlock age. Stippled area indicates orogenic belts.
With the aim of identifying major minerals in the sampled interbeds, randomly oriented bulk samples were analysed by X-ray diffractometry (XRD). An association of illite–smectite and kaolinite as major minerals has been considered to confirm the volcanic origin of the interbeds. More rarely, volcanic ash has been altered to authigenic K-sanidine with minor illite–smectite and kaolinite (Hints et al. Reference Hints, Kirsimäe, Somelar, Kallaste and Kiipli2008). Host shales are composed of a different association of terrigenous minerals including illite, quartz, chlorite and minor K-feldspar.
Magmatic sanidine phenocryst (K,Na,Ca)AlSi3O8 composition has proved to be a very useful indicator of particular volcanic eruption layers (bentonites) in earlier Telychian sections from the East Baltic area (Kiipli & Kallaste, Reference Kiipli and Kallaste2002; Kallaste & Kiipli, Reference Kallaste and Kiipli2006; Kiipli et al. Reference Kiipli, Kallaste, Nestor and Loydell2010). Kastner (Reference Kastner1971) demonstrated that sedimentary authigenic feldspars have pure end-member compositions KAlSi3O8 or NaAlSi3O8 only. Therefore we consider intermediate compositions of the K–Na feldspar (sanidine) as an indication of magmatic origin.
A small percentage of Ca substituted for Na and minor Ba substituted for K in alkali feldspar (Ginibre, Wörner & Kronz, Reference Ginibre, Wörner and Kronz2004) can influence the position of the sanidine 201 reflection. Therefore numerical values of sanidine composition, calculated from that reflection, in reality show the content of the Na+Ca component in mol %. Considering this effect as minor in our previous works (Kiipli & Kallaste, Reference Kiipli and Kallaste2002 and other later publications), sanidine composition was expressed in units of Na-component concentration in (K,Na)AlSi3O8. A more accurate expression, Na+Ca component in sanidine, is adopted here. Numerical values herein are still strictly comparable with those in our previous publications.
The phenocrysts were analysed from coarse fractions (0.04–0.1 mm) separated from 2 grams of bentonite. The fraction 0.04–0.1 mm constitutes a very small percentage of the bulk bentonite. Sanidine dominates among phenocrysts with less abundant biotite and quartz. While biotite occurs often as hexagonal plates, sanidine and quartz are commonly represented by irregular fragments (shards) of the original crystals. Minor apatite and zircon are observed in some layers. Plagioclase phenocrysts, which are commonly the most abundant in silicic magmas, are not preserved in the East Baltic ash beds, since they were most likely dissolved and recrystallized into clay minerals. The 201 reflection was measured in a range from 23.5 to 26.0 °2θ using Fe filtered Co Kα radiation. From the position of the 201 reflection, the (Na,Ca)AlSi3O8 content in (K,Na,Ca)AlSi3O8 solid solution (sanidine) was calculated according to Orville (Reference Orville1967). In favourable cases (sharp reflection and low content of authigenic potassium feldspar), the precision of the method was ± 1 %. In less favourable cases the precision was ± 2 %. Checking of the method by EDS microanalyses in two samples showed reasonable accordance within analytical uncertainty of both methods; in Ventspils 715.4 m EDS analysis showed Na0.45Ca0.03K0.52Ba0.00Al1Si3O8 and XRD showed (Na,Ca)0.50(K,Ba)0.50Al1Si3O8; in Ventspils 720.6 m EDS showed Na0.32Ca0.01K0.65Ba0.02Al1Si3O8 and XRD showed (Na,Ca)0.30(K,Ba)0.70Al1Si3O8. XRD analysis is performed simultaneously on 1000–2000 grains of sanidine and therefore represents average composition of the sample better than EDS microanalysis performed on only some tens of grains. All measured XRD spectra are available in the collections database of the Institute of Geology Tallinn University of Technology at http://sarv.gi.ee/reference.php?id=1203.
Many volcanic ash beds show a very wide sanidine reflection which does not permit identification of a particular bed, but nevertheless separates these beds clearly from those with a sharp reflection. Wide reflections indicate heterogeneous composition of the sanidine, caused most probably by presence of the zoned crystals in a source magma. Examples of the measured XRD profiles with curve fitting results from the Wenlock of Latvia are shown in Figure 2.

Figure 2. Selected sanidine spectra from studied bentonites. Points mark measured curve, and solid lines reflections of minerals calculated using three, four or five component curve-fitting program; from left to right: quartz, authigenic potassium feldspar (K-fsp) and magmatic K–Na-sanidine (K–Na fsp) described by one, two or three components. Numbers above reflection represent calculated (Na,Ca)AlSi3O8 content (mol %) in sanidine main component. (a–i) Various types of sanidine spectra from 23.5 to 25.5 degrees. (j) Longer XRD pattern of the sanidine from the Vidale core depth 681.1 m.
Samples of sufficient size, at least 8 g, were analysed by the standard X-ray fluorescence (XRF) method from pressed powders for major and trace elements, applying empirical correction coefficients to the measured intensities of spectral lines, at the Institute of Geology Tallinn University of Technology. Precision of the method was mostly better than ± 5 % of the concentration and for trace elements in the range 10–30 ppm better than ± 10 %. For calibration and quality control, reference materials from France (Govindaraju, Reference Govindaraju1995) and Estonia (Kiipli et al. Reference Kiipli, Batchelor, Bernal, Cowing, Hagel-Brunnstrom, Ingham, Johnson, Kivisilla, Knaack, Kump, Lozano, Michiels, Orlova, Pirrus, Rousseau, Ruzicka, Sandstrom and Willis2000) were used. Long range accuracy of the method is shown in Kiipli et al. (Reference Kiipli, Orlova, Kiipli and Kallaste2008b).
3. Stratigraphy
In terms of chitinozoan biozonation, the studied interval in the Ventspils D3 and Vidale 263 cores belongs to the Margachitina margaritana to Conochitina subcyatha biozones (Figs 3, 4). According to Nestor (Reference Nestor1994), this interval comprises the lower Sheinwoodian to lower Homerian. In Vidale 263 the Conochitina tuba, Cingulochitina cingulata, Eisenackitina spongiosa and Conochitina pachycephala biozones were established (Fig. 3).

Figure 3. Distribution of chitinozoans in the Vidale 263 core.

Figure 4. Geological sections of studied drill cores. 1 – traditional Llandovery/Wenlock boundary at the FAD of Cyrtograptus centrifugus marked by the Aizpute Bentonite (Martinsson, Bassett & Holland, Reference Martinsson, Bassett and Holland1981; Kiipli et al. Reference Kiipli, Kallaste, Nestor and Loydell2010). 2 – Llandovery/Wenlock boundary proposed by Kiipli & Kallaste (Reference Kiipli and Kallaste2006) and Kiipli et al. (Reference Kiipli, Jeppsson, Kallaste and Söderlund2008a) marked by the Ireviken Bentonite. 3–10 – finds of zonal graptolites (Loydell, Kaljo & Männik, Reference Loydell, Kaljo and Männik1998; Gailite, Ulst & Jakovleva, Reference Gailite, Ulst and Jakovleva1987; Põldvere, Reference Põldvere2003); 3 – Cyrtograptus murchisoni, 4 – Monograptus firmus, 5 – Monograptus riccartonensis, 6 – Streptograptus antennularius, 7 – Monograptus flexilis, 8 – Cyrtograptus radians, 9 – Testograptus testis, 10 – Cyrtograptus lundgreni.
4. Results
4.a. General results
In the studied stratigraphic range (in terms of chitinozoan biozones from M. margaritana to C. subcyatha), 71 bentonite interbeds occur in four drill cores (Fig. 4). Using the chitinozoan biozonation as a framework and based on the sanidine properties, these bentonites can be grouped into 47 volcanic eruption layers (Table 1). Previously, in the Ohesaare and Ruhnu cores (Kiipli & Kallaste, Reference Kiipli and Kallaste2006) only 28 eruption layers were recognized in this interval. In the Vattenfallet exposure on Gotland, a bentonite with a sharp sanidine reflection and 28.5 mol % of Na+Ca-component was found within the upper K. ranuliformis conodont Biozone (Kiipli et al. Reference Kiipli, Jeppsson, Kallaste and Söderlund2008a). In Lithuania near the antennularius/flexilis graptolite biozone boundary, a bentonite with a wide sanidine reflection and about 26–27 mol % of Na+Ca component was identified (Kiipli et al. Reference Kiipli, Radzevičius, Kallaste, Motuza, Jeppsson and Wicksröm2008c). These two bentonites have not been found in Estonian and Latvian sections. Therefore the total volcanic record at the level of present knowledge comprises 49 eruption layers in the studied interval. This list is still provisional, because only eight correlations are well proved, based on sharp sanidine reflections (Table 1; Figs 4, 5), and nine correlations are less certain, characterized by wide sanidine reflections. Consequently, the larger number of eruption layers is established only in a single section.
Table 1 Occurrence of Wenlock bentonites in the frame of chitinozoan biozonation

+++ Biotite is abundant; ++ Biotite is rare (10–100 flakes); + Less than 10 flakes; M – muscovite

Figure 5. Sanidine composition in the lower and middle Wenlock. Frames join sanidine from correlated ash beds. Many ash beds with sanidine showing wide reflections are not represented here, because the compositions of these sanidines cannot be reliably expressed in numerical values by the method used. Black circles – Vidale (Vi); black triangles – Ventspils (Ve); grey rhombs – Ohesaare (Oh); empty squares – Ruhnu (Ru) section. Numbers represent depths of correlated bentonites (m).
Several bentonites are also newly recognized with a previously unknown type of sanidine XRD spectrum indicating the presence of sodium-rich, up to 58 mol %, components in alkali feldspar phenocrysts (Figs 2, 5).
4.b. Margachitina margaritana Biozone
This interval includes four widespread eruption layers: Aizpute (ID311), Ohesaare (ID210), Lusklint (ID150) and Ireviken (ID127). Depths of these bentonites in the Ohesaare core are 351.7 m, 345.8 m, 342.1 m and 340.8 m, respectively (Fig. 4). Bentonite names and ID numbers are those of Kallaste & Kiipli (Reference Kallaste and Kiipli2006) and Kiipli et al. (Reference Kiipli, Kallaste, Nestor and Loydell2010). The Ohesaare Bentonite and the Ireviken Bentonite are also found in the Ventspils core at depths 792.8 m and 789.2 m, respectively (Table 1; Fig. 4). Additionally, 5 cm above the Ohesaare Bentonite a new eruption layer with a sharp sanidine reflection and 37.4 mol % of Na+Ca component was found. In Vidale not one of these layers was found. A possible reason is poor core recovery in this interval causing destruction of thin soft volcanic ash beds.
4.c. Conochitina mamilla Biozone
Three bentonites containing sanidine with a very wide reflection occur here. Often these very wide reflections exhibit a specific plateau-like shape with a relatively abrupt fall at the Na-rich end of the spectrum (Fig. 2h). The Ohesaare 323.2 m and Ruhnu 435.84 m beds consist of two layers differing in colour. The first contains sanidine with a plateau-like spectrum and the other sanidine with a sharp reflection with 45–46 mol % of Na+Ca component. In Ventspils 767.15 m and Vidale 709.90 m, only the plateau-like type was found.
The Vidale 703.15 bed, possibly belonging to this biozone, contains sanidine with a sharp reflection.
4.d. Conochitina tuba Biozone
In the lower part of the biozone, two bentonites with wide sanidine reflections occur in the Ohesaare and Ruhnu sections. In the upper part of the biozone in Ohesaare, four bentonites with sharp sanidine reflections have been found. In Vidale 694.90 m a sharp sanidine reflection was also found, being very similar to the Ohesaare 301.95 m and Ohesaare 301.36 m beds. According to the sampling depth records, the Vidale 694.90 m bentonite must belong to the lower part of the C. cingulata Biozone, but this is only 10 cm above the first sample with C. cingulata, and small uncertanties in depth interpretations between bentonite researchers and biostratigraphers are possible. We consider the Vidale 694.90 m bed as belonging in the C. tuba Biozone on the basis of a very similar sanidine XRD reflection with bentonites from the upper part of C. tuba in Ohesaare.
The step-by-step rise of the Na+Ca-component in sanidine in closely spaced eruption layers starting from Vidale 703.15 m to Ohesaare 300.25 m is remarkable. This may indicate the fractional crystallization and settling of potassium-rich sanidine into the lower parts of a magma chamber.
4.e. Cingulochitina cingulata Biozone and lower part of Eisenackitina spongiosa Biozone
Most of the approximately thirteen eruption layers in the C. cingulata Biozone are characterized by sanidines with wide and very wide spectra, and correlations are therefore hypothetical. In the lower part of the biozone an ash bed containing muscovite can be recognized in the Ohesaare 294.23 m, Ventspils 746.10 m and Vidale 689.40 m beds. Another eruption layer containing muscovite occurs in the upper part of the biozone in the Ruhnu core at a depth of 388.40 m.
Remarkable layers occur in the middle and upper part of the C. cingulata Biozone with wide sanidine reflections (Figs 2e–f, 5) requiring five-component fitting of the XRD spectrum (commonly four-component fitting is sufficient). These five fitting profiles (XRD reflections) represent quartz, K-sanidine and three K–Na sanidine components. This type occurs in the Vidale core at depths of 687.80 m, 686.60 m and 685.00 m. In the Ohesaare core this type occurs at a depth 275.32 m. This layer was previously correlated with the Y Bentonite from the Slite quarry on Gotland, Sweden (Kiipli et al. Reference Kiipli, Jeppsson, Kallaste and Söderlund2008a). In the Ventspils core this type of sanidine occurs at a depth of 738.60 m in the C. cingulata Biozone and 737.10 m and 736.40 m in the lower part of the E. spongiosa Biozone. During sampling of the Ventspils core, we noticed that there was low recovery of core at this level, and no clearly understandable depth records. Therefore it cannot be excluded that occurrence of some beds of this type in the E. spongiosa Biozone can be considered as an artefact arising from different interpretations of depths. Future studies of more sections must make clear the real number of eruption beds of this type. In Table 1 and online Appendix Table 3, the fourth (main) component of the sanidine exhibiting 30–33 mol % of the Na+Ca-component is reported. The fifth component has lower intensity and contains about 50 mol % (± 4 mol %) of the Na+Ca-component.
In addition to the beds with wide sanidine reflections, a few with sharp reflections have also been discovered, for example, in beds from Ohesaare 288.44 m and Vidale 681.10 m. The latter is characterized by an unusually high content of the Na+Ca-component, 57.9 mol % (Fig. 2g).
4.f. Eisenackitina spongiosa Biozone
As well as two bentonites containing sanidine with wide reflections in the lower part (see above), we found three bentonites in this biozone in Vidale containing sharp sanidine reflections (Table 1). A bentonite from Ruhnu at 374 m also contains sanidine with a similar Na+Ca-component to that in Vidale 677.60 m, but the reflection is much sharper and this raises some doubt about the correlation of these layers.
4.g. Conochitina pachycephala Biozone
At the lower boundary of the biozone an ash bed containing sanidine with a very wide reflection occurs in the Ventspils (728.00 m) and Vidale (670.70 m) cores. In the upper part of the biozone in the Ohesaare (233.40 m) core, another eruption layer occurs with similar sanidine.
4.h. Conochitina subcyatha Biozone
In the lower and upper part of this biozone several eruption layers occur with sharp sanidine reflections and 23–35 mol % of the Na+Ca component. In the middle of the biozone in the Ventspils (715.40 m, 714.10 m) and Vidale (646.80 m, 644.60 m, 634.10 m) cores occur bentonites with a wide sanidine reflection and around 50 mol % of the Na+Ca component (Fig. 2b, c). This type of sanidine was not found in previous studies (Kiipli & Kallaste, Reference Kiipli and Kallaste2006; Kiipli et al. Reference Kiipli, Jeppsson, Kallaste and Söderlund2008a, Reference Kiipli, Radzevičius, Kallaste, Motuza, Jeppsson and Wicksrömc).
4.i. Geochemistry of major components and the discrimination between volcanic ash interbeds and host rock
The host rocks in the Wenlock part of these cores are represented by shales containing 15–30 % of carbonate material. Illite, chlorite, quartz, calcite and dolomite are major minerals in the host shales. Major minerals in the bentonites are kaolinite, illite–smectite, K-feldspar and pyrite. XRF analyses (online Appendix at http://journals.cambridge.org/geo) showed unusually high contents of sulphur in bentonites reaching 9 % (Fig. 6a). Host marlstones contain on average only 0.5 % sulphur. Judging from the composition of bentonites showing low sulphur content, altered volcanic ashes contain only about 1–2 % of silicate Fe2O3. Host shales contain 3–5 % of silicate Fe2O3. A larger deviation of the bentonite regression line from the pyrite line at higher concentrations is caused by weathering of pyrite and partial removal of sulphur in drill-core boxes during the long storage time (20 years for Vidale 263 and 40 years for the Ventspils D3 core). This is confirmed by the occurrence of jarosite and gypsum reflections on XRD patterns from samples with the highest content of sulphur. The probable reason for the higher content of pyrite in bentonites compared with host shales is that the rapid accumulation of volcanic ash changes the decay of organic matter from oxic on the sea floor to sulphate reducing in the sediment.

Figure 6. Comparison of major components in altered volcanic ash beds and host shales and marlstones. Black triangles – Wenlock bentonites from the Ventspils D3 and Vidale 263 drill cores. Crosses – host shales from the same cores. Rock fields on (b) are composed using analytical data from Kiipli et al. (2008b, d).
Bentonites in the Ventspils and Vidale cores are characterized by a low (1–5 %) K2O content in 90 % of the analysed samples and a high (24–35 %) Al2O3 content in 80 % of the samples. The abundance of kaolinite and consequently the low-potassium high-aluminium type of altered volcanic ash beds is typical of Palaeozoic deep shelf environments in the East Baltic (Kiipli et al. Reference Kiipli, Kiipli, Kallaste, Hints, Somelar and Kirsimäe2007; Hints et al. Reference Hints, Kirsimäe, Somelar, Kallaste and Kiipli2008). This composition means that in this report we favour using the term ‘bentonite’ instead of ‘K-bentonite’, the latter being characterized by much higher (5–12 %) potassium concentrations (Kiipli et al. Reference Kiipli, Orlova, Kiipli and Kallaste2008b). High-aluminium kaolinite-rich altered volcanic ash beds found in coal formations are called tonsteins (Bohor & Triplehorn, Reference Bohor and Triplehorn1993). In Figure 6b the contents of Al2O3 and K2O in the studied samples are recalculated to a pyrite-free composition. The background rock type fields on the chart are composed according to analytical data in Kiipli et al. (Reference Kiipli, Orlova, Kiipli and Kallaste2008b, Reference Kiipli, Soesoo, Kallaste and Kiiplid). The host rocks cluster in a clearly different field caused by the higher content of quartz and carbonates. Within the central and southern part of the Baltic Basin between depths 600 and 800 m (studied depth range in Ventspils and Vidale) in bentonites, the content of smectite layers in illite–smectite ranges between 25 and 45 % (Somelar, Reference Somelar2009).
4.j. Geochemistry of trace elements, interpretation of source magma and tectonic setting
4.j.1. General
Volcanic ash alteration on the seafloor and in sediments leads to the formation of clay minerals. During this process 50 % or more of the original SiO2 and cations are leached out (Huff, Kolata & Bergström, Reference Huff, Kolata and Bergström1996; Kiipli, Kiipli & Kallaste, Reference Kiipli, Kiipli and Kallaste2006). Therefore, easily soluble elements in bentonites cannot be used for interpreting the source magma. In contrast, concentrations of elements of low solubility increase in the forming bentonites. For example, the Al2O3 content in silicic magmatic rocks varies mostly between 10 and 18 %, but in altered volcanic ashes (bentonites) the Al2O3 content is much higher being within a range of 18–36 % (Fig. 6b). Alternatively, immobile trace elements (Ti, Zr, Y, Nb, Ce, Ga, Sc) can be used tentatively to interpret initial magma compositions (Winchester & Floyd, Reference Winchester and Floyd1977). The problem is that concentrations of immobile elements also rise during the formation of clay from volcanic ash. In this paper, following the approach proposed by Kiipli et al. (Reference Kiipli, Soesoo, Kallaste and Kiipli2008d), we are using corrected values for immobile trace elements calculated as follows:

where IS is approximate concentration of immobile trace element in source magma; IB is immobile trace element concentration in bentonite; 14 is average content of Al2O3 in silicic magmatic rocks (%), (Turekian & Wedepohl, Reference Turekian and Wedepohl1961); Al2O3 is content of aluminium oxide in bentonite (%).
This calculation gives values on average 50 % lower for immobile trace elements than analysed in bentonites, and these values are considered to be closer to the original concentrations. Scaling immobile trace element concentrations back to the supposed initial Al2O3 content enables more accurate use of many trace element diagrams. This method enables the use of element ratio diagrams as well as element concentration diagrams for altered volcanic rocks such as bentonites and K-bentonites.
4.j.2. Comparison with Pliocene to Quaternary volcanism in Italy
Intensive volcanic activity during recent geological time in Italy is represented by variable compositions of magmas forming all known volcanic rock types (Peccerillo, Reference Peccerillo2005). As a hypothesis, the tectonomagmatic environment in the Mediterranean may be considered similar to the environment in a remnant of the Iapetus Ocean between colliding Baltica and Laurentia in Wenlock times.
In Figure 7 the fractionation index, the Zr/TiO2 ratio, is plotted against Nb concentrations interpreted as an alkalinity index (Winchester & Floyd, Reference Winchester and Floyd1977; Pearce & Norry, Reference Pearce and Norry1979). Using about 1500 analyses of volcanic rocks published by Peccerillo (Reference Peccerillo2005) as a framework (Fig. 7), the source magmas of Wenlock bentonites from the East Baltic can be interpreted as subalkaline, ranging from andesite to rhyolite. Overlapping of compositional ranges in Italian rocks does not exclude some mildly alkaline source magmas for East Baltic bentonites, but also does not prove it. Clearly, strongly alkaline source magmas did not provide the volcanic ash for the East Baltic bentonites. Comparison with earlier Telychian bentonites (Kiipli et al. Reference Kiipli, Soesoo, Kallaste and Kiipli2008d) reveals essentially overlapping compositional ranges for both ages.

Figure 7. Comparison of Wenlock volcanism (black triangles) and earlier Telychian volcanism (empty rhombs) in the East Baltic with Pliocene to Quaternary volcanism in Italy using the Zr/TiO2 ratio and Nb. Grey rings represent the geometric mean of the calc-alkaline rocks and grey quadrangles alkaline rocks from Italy. One standard deviation (as a coefficient) is shown by bars. Data from Italian volcanic rocks are from Peccerillo (Reference Peccerillo2005), Telychian bentonites from Kiipli et al. (Reference Kiipli, Soesoo, Kallaste and Kiipli2008d) and Wenlock bentonites in addition to the present study are from Batchelor & Jeppson (Reference Batchelor and Jeppsson1999) and Kiipli et al. (Reference Kiipli, Jeppsson, Kallaste and Söderlund2008a, Reference Kiipli, Radzevičius, Kallaste, Motuza, Jeppsson and Wicksrömc, Reference Kiipli, Soesoo, Kallaste and Kiiplid).
4.j.3. Zr/TiO2–Ga chart
Winchester & Floyd (Reference Winchester and Floyd1977) proposed Ga in addition to Nb as an alkalinity index for magmatic rocks. Plotting the East Baltic bentonite data onto the framework composed by Winchester & Floyd (Reference Winchester and Floyd1977; Fig. 8) provides confirmation of the interpretation made from comparison with Italian rocks, that most source magmas of East Baltic bentonites were subalkaline. Corrected Ga concentrations are too low for alkaline magmas. Batchelor & Jeppsson (Reference Batchelor and Jeppsson1999), based on the composition of apatite phenocrysts, proposed alkaline as well as calc-alkaline magma as a source for the some Wenlock bentonites from Gotland. Wenlock bentonites show, on average, lower corrected Ga concentrations than Telychian ones.

Figure 8. Comparison of Wenlock and Telychian volcanism from the East Baltic on the Zr/TiO2–Ga chart according to Winchester & Floyd (Reference Winchester and Floyd1977). Black triangles – Wenlock bentonites; empty rhombs – Telychian bentonites.
4.j.4. Interpretation of tectonic setting
Using corrected values of Y and Nb on the diagram for granitic rocks proposed by Pearce, Harris & Tindle (Reference Pearce, Harris and Tindle1984), these data indicate mostly volcanic arc and syn-collisional tectonic environments for the volcanic sources of the Wenlock bentonites (Fig. 9). A smaller number of points fall in the within-plate granites field.

Figure 9. Comparison of Wenlock and Telychian volcanism from the East Baltic on the Y–Nb plot for granitic rocks according to Pearce, Harris & Tindle (Reference Pearce, Harris and Tindle1984). Black triangles – Wenlock bentonites; empty rhombs – Telychian bentonites. VAG+syn-COLG – volcanic arc and syn-collisional granites; WPG – within plate granites; ORG – ocean ridge granites.
Wenlock and earlier Telychian volcanism shows a largely overlapping distribution of concentrations with the exception of some Telychian bentonites that reveal lower Y values.
4.j.5. Sanidine composition compared in Wenlock and Telychian bentonites
Sanidine composition in Wenlock bentonites shows a bimodal distribution clustering dominantly between 28–40 mol % and 44–58 mol % of the Na+Ca component (Fig. 10). Sanidine in Telychian bentonites also shows a bimodal distribution of composition clustering at 20–30 and 38–48 mol %. Sanidine in Wenlock bentonites is on average more sodic compared to that of Telychian bentonites.

Figure 10. Frequency of the sanidine composition in the East Baltic bentonites. For explanation of separation line between K-dominated and Na-dominated source magma, see text and Figure 12.
Comparison of the sanidine compositions with the Zr/TiO2 ratio (fractionation index) shows that the less evolved dacitic Telychian magmas had more potassic compositions of the sanidine and the highly evolved rhyolitic magmas had more sodic sanidine compositions (Fig. 11). By contrast, later in Wenlock times, less evolved magmas included more sodic sanidine phenocrysts than the highly evolved rhyolitic magmas.
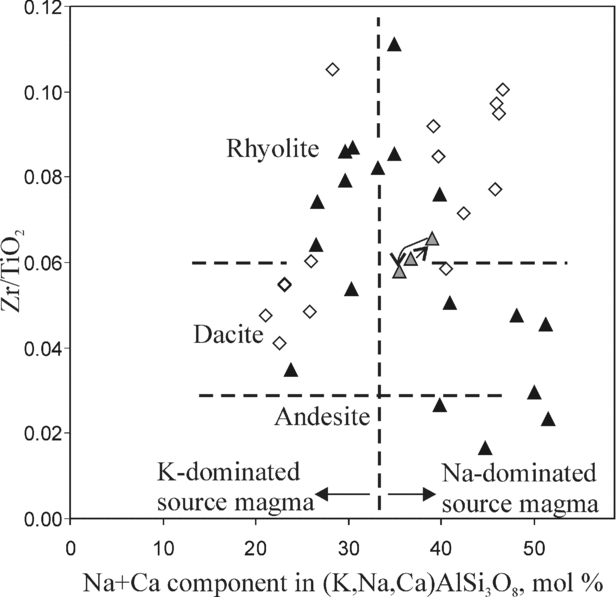
Figure 11. Sanidine composition and Zr/TiO2 in the East Baltic bentonites with tentative interpretation of source magma. Approximate separation lines between different source magma types are according to Figures 7, 8 and 12. Grey triangles connected with arrows – the lowermost successive bentonites (Aizpute, Ohesaare and Lusklint) originated most probably from the same source. Arrows show temporal succession of eruptions.
5. Discussion
5.a. Use of sanidine phenocryst composition for geochemical fingerprinting of volcanic eruptions and correlation of sections
High sanidine forms in magma chambers during cooling at high temperatures and is a metastable mineral at Earth surface temperatures (Gill, Reference Gill1996). After the fast cooling during volcanic eruption, recrystallization of the mineral slows down significantly and has not proceeded much in the East Baltic area, even during the 400 Ma from the Silurian to the present day. This may not be the case everywhere. For example, attempts to analyse sanidine by XRD from the Silurian bentonites of the Oslo region (Norway) correlated by trace elements with bentonites containing sanidine in Estonia (Kiipli et al. Reference Kiipli, Männik, Batchelor, Kiipli, Kallaste and Perens2001) did not reveal measurable reflections. Evidently the rocks were heated too much during Caledonian orogenesis or Permian magmatism. Another reason for the lack of sanidine may be a highly chemically reactive environment in some organic rich black shales, for example, in Bornholm, where we also could not find sanidine by XRD. Sanidine may already have been absent in source magma. The precise causes of a lack of sanidine in particular cases are unknown and must be the topic of future studies. Our present knowledge indicates that XRD measurements of the sanidine composition can be used successfully for geochemical fingerprinting of volcanic ash layers in Estonia, Latvia, Lithuania and Gotland (Sweden). This method can probably also be used in some areas of the mainland of Sweden, as suggested by dating of the Ordovician bentonite from Kinnekulle (southern Sweden) by the K–Ar method using sanidine (Byström-Asklund, Baadsgaard & Folinsbee, Reference Byström-Asklund, Baadsgaard and Folinsbee1961).
For establishing correlations of sections by chemical and/or mineralogical fingerprinting of the volcanic ash beds in Palaeozoic sections, two approaches can be used:
(1) Analysing as many geochemical and mineralogical parameters as possible with the aim of finding the unique signature of the particular eruption layer. This approach was used by Bergström et al. (Reference Bergström, Huff, Kolata and Bauert1995), Hetherington, Nakrem & Batchelor (Reference Hetherington, Nakrem and Batchelor2004), Batchelor (Reference Batchelor2009) and Inanli, Huff & Bergström (Reference Inanli, Huff and Bergström2009). Searching for the unique fingerprint is certainly important, but is time consuming and expensive work, and cannot be easily done for a large number of ash beds. This method is well applicable where a few eruption layers occur in sections.
(2) Another approach, analysing with maximum possible accuracy only a single significant parameter, is preferable in sections containing a large number of ash beds (Kiipli et al. Reference Kiipli, Kallaste, Nestor and Loydell2010). The problem is that in this case the same composition (sanidine composition in our study) can occur repetitively in a section. For example, sharp sanidine reflections with a similar content of the Na+Ca component between 28 and 32 mol % occur in the East Baltic Silurian in 12 eruption layers (Kiipli & Kallaste, Reference Kiipli and Kallaste2006; Kiipli et al. Reference Kiipli, Kallaste, Nestor and Loydell2010; present study). As the East Baltic Silurian contains more than 100 established volcanic eruption layers, determination of the sanidine composition restricts possible variants for correlation considerably. Additional constraints can be obtained from the biostratigraphical information. Analysing all occurring layers for a single primary magmatic signature with very precise methods enables establishment of a unique succession of many eruptions. The unique temporal succession of several volcanic ash beds can be used, together with biostratigraphy, for correlation of sections even when the analysed parameter shows the same value in several ash beds.
5.b. Integrated petrogenetic interpretation and possible location of source volcanoes
Analysis of immobile trace elements has enabled a provisional estimate of the source magma of the Wenlock bentonites as being subalkaline, ranging from dacite to rhyolite, originating in volcanic arc and syncollisional tectonic settings. Andesite as a source magma indicated by some low Zr/TiO2 ratios is less probable, because East Baltic Silurian volcanic ashes commonly contain sanidine as a major phenocryst. In recent andesites, sanidine occurs only rarely as a minor phenocryst (Winter, Reference Winter2001, p. 303).
Analysis of sanidine composition provides an additional tentative possibility of estimating the potassium/sodium ratio in source magmas. When temperature decreases in a binary NaAlSi3O8–KAlSi3O8 system, the first crystallizing phase is a potassium-rich sanidine, followed later by a more sodic one (Bowen & Tuttle, Reference Bowen and Tuttle1950). Real magmas are multicomponent systems and crystallization is more complicated. However, empirical comparison of sanidine composition with coexisting volcanic glass and whole rock shows, although strongly scattered, the same relationship (Fig. 12). Pyroclastic sanidine in the East Baltic Silurian forms commonly only a small percentage of the bentonite, indicating that eruptions occurred at the initial stages of sanidine crystallization. Therefore we may suppose higher Na/K ratios in source magma than in sanidine. Based on Figure 12, we can deduce that bentonites containing sanidine with less than approximately 33 mol % of Na+Ca component probably crystallized from potassium-dominated magma and those with more sodium-rich sanidine from the magma where sodium dominates over potassium.

Figure 12. Comparison of the Na/(Na+K) atom ratio in sanidine and coexisting volcanic glass or whole rock. Black – comparison with glass; grey – comparison with whole rock. Rhombs – alkaline rocks; squares – rhyolites; triangles – dacites. Data sources: Anderson, Davis & Lu (Reference Anderson, Davis and Lu2000); Basu & Vitaliano (Reference Basu and Vitaliano1976); Carmichael (Reference Carmichael1967); Chesner (Reference Chesner1998); Christiansen (Reference Christiansen2001); Gaeta (Reference Gaeta1998); Henry, Price & Smyth (Reference Henry, Price and Smyth1988); Landi, Bertagnini & Rosi (Reference Landi, Bertagnini and Rosi1999); Maughan et al. (Reference Maughan, Christiansen, Best, Gromme, Deino and Tingey2002); Macdonald, Rogers & Tindle (Reference Macdonald, Rogers and Tindle2007); McHenry (Reference McHenry2009); Morgan et al. (Reference Morgan, Blake, Rodger, De Vivo, Rolandi and Davidson2006); Pappalardo, Ottolini & Mastrolorenzo (Reference Pappalardo, Ottolini and Mastrolorenzo2008); Sacchi et al. (Reference Sacchi, Insinga, Milia, Molisso, Raspini, Torrente and Conforti2005); Smith (Reference Smith1974, pp. 47, 50); Zellmer & Clavero (Reference Zellmer and Clavero2006).
Intrusive rocks of Wenlock age are known from the Scandinavian Caledonides in South Central Norway around Trondheim (Corfu et al. Reference Corfu, Torsvik, Andersen, Ashwal, Ramsay and Roberts2006). Massive metavolcanic rocks of Silurian age occur in the Northern Phyllite Zone in Germany (Timmerman, Reference Timmerman and McCann2008) and in the Holy Cross mountains in Poland (Krawczyk, Reference Krawczyk, McCann, Cocks, England, McBride, Wybraniec and McCann2008). Therefore at the level of present knowledge, volcanic sources for the East Baltic bentonites of Wenlock age could have been in a collision zone between Baltica and Laurentia or in the Central European Caledonides. Mapping of the underlying Telychian and overlying upper Wenlock volcanic ash layers indicated an origin from the Iapetus palaeo-ocean (Kiipli et al. Reference Kiipli, Jeppsson, Kallaste and Söderlund2008a, Reference Kiipli, Orlova, Kiipli and Kallasteb, Reference Kiipli, Radzevičius, Kallaste, Motuza, Jeppsson and Wicksrömc, Reference Kiipli, Soesoo, Kallaste and Kiiplid). To enable composition of volcanic ash distribution maps, the lower–middle Wenlock bentonites need to be studied in a wider area in the future.
6. Conclusions
Study of sanidine in the lower and middle Wenlock part of the Ventspils D3 and Vidale 263 drill cores reveals about 20 previously unknown eruption layers and gives, in combination with the formerly studied Ohesaare and Ruhnu cores, the most complete list available at this time of volcanic beds for the East Baltic area constrained by the chitinozoan biozonation. XRD measurements revealed several new types of sanidine spectra, in particular, samples with a high Na+Ca (48–58 mol %) content. Sanidine in the studied part of the Wenlock in the East Baltic is of variable composition, having excellent potential for geochemical fingerprinting of volcanic eruption layers.
Immobile trace elements and the sanidine composition indicate subalkaline volcanism generated in volcanic arc and syncollisional tectonic environments. Sanidine composition suggests that both potassium- and sodium-dominated source magmas occurred in the Silurian at the margins of the Baltica plate.
Acknowledgements
This study is a contribution to the Estonian Science Foundation grant 7605 and target financing projects SF0140016s09 and SF0140020s08. We thank A. Murnieks, R. Pomeranceva and R. Einasto for kindly helping in sampling in the Latvian Agency of Environment, Meteorology and Geology, the late K. Orlova for XRF analyses, D. K. Loydell for correcting language and two anonymous referees for help.