1. Introduction
Although molecular clock studies estimate the origin and earliest diversification of animals within the Cryogenian Period, evidence from the fossil record reveals that crown members of nearly all animal phyla appear in a relatively rapid diversification event in early Cambrian time (the ‘Cambrian explosion’). This evolutionary radiation follows after a global mass extinction of the Ediacaran fauna (Erwin et al. Reference Erwin, Laflamme, Tweedt, Sperling, Pisani and Peterson2011), a benthic community dominated by sessile, substrate-sticking organisms (Seilacher, Reference Seilacher1999; Fedonkin et al. Reference Fedonkin, Gehling, Grey, Narbonne and Vickers-Rich2007; Yuan et al. Reference Yuan, Chen, Xiao, Zhou and Hua2011). In addition, geological and geochemical data suggest that the Ediacaran–Cambrian transition was not only a transition in biological diversity and ecosystem structure, but was also associated with drastic environmental change. These changes in environment and biology were likely highly intertwined; for instance, the rise of oxygen (e.g. Fike et al. Reference Fike, Grotzinger, Pratt and Summons2006; Canfield et al. Reference Canfield, Poulton, Knoll, Narbonne, Ross, Goldberg and Strauss2008; Komiya et al. Reference Komiya, Hirata, Kitajima, Yamamoto, Shibuya, Sawaki, Ishikawa, Shu, Li and Han2008; Canfield & Farquhar, Reference Canfield and Farquhar2009; Li et al. Reference Li, Love, Lyons, Fike, Sessions and Chu2010) has been suggested to have had profound effects on the evolution and diversification of metazoans (Sperling et al. Reference Sperling, Frieder, Raman, Girguis, Levin and Knoll2013). Further, the innovation of novel ecological strategies, such as ecosystem engineering and predation, in Ediacaran communities nearing the Cambrian boundary may have served to set the stage for the impending Cambrian radiation (e.g. Schiffbauer et al. Reference Schiffbauer, Huntley, O'Neil, Darroch, Laflamme and Cai2016). Thus, the Ediacaran–Cambrian boundary represents a revolutionary transition, wherein the combined effects of environmental, biological and ecological change impart a large influence on phylogenetic patterns for the next c. 540 million years of evolutionary history.
With several reports of the survival of a few taxa from the Ediacaran fauna into the early and middle Cambrian period (e.g. Jensen, Gehling & Droser, Reference Jensen, Gehling and Droser1998; Hagadorn & Waggoner, Reference Hagadorn and Waggoner2000; Shu et al. Reference Shu, Morris, Han, Li, Zhang, Hua, Zhang, Liu, Guo, Yao and Yasui2006; Van Iten et al. Reference Van Iten, De Moraes Leme, Sim Es, Marques and Collins2006), palaeontologists have sought to better understand the nature of biotic replacement at the Ediacaran–Cambrian transition (Laflamme et al. Reference Laflamme, Darroch, Tweedt, Peterson and Erwin2013; Darroch et al. Reference Darroch, Sperling, Boag, Racicot, Mason, Morgan, Tweedt, Myrow, Johnston and Erwin2015), and moreover, to explore the possible extinction hold-overs and their place within this evolutionary story. One such group of possible hold-overs comprises the terminal Ediacaran tubular biomineralizing assemblage (part of the ‘wormworld fauna’, Schiffbauer et al. Reference Schiffbauer, Huntley, O'Neil, Darroch, Laflamme and Cai2016), exemplified by such organisms as Cloudina, Sinotubulites and Namacalathus (e.g. Germs, Reference Germs1972; Signor, Mount & Onken, Reference Signor, Mount and Onken1987; Grant, Reference Grant1990; Grotzinger, Watters & Knoll, Reference Grotzinger, Watters and Knoll2000; Hofmann & Mointjoy, Reference Hofmann and Mointjoy2001; Cortijo et al. Reference Cortijo, Mus, Jensen and Palacios2010; Zhuravlev et al. Reference Zhuravlev, Li, Vintaned, Debrenne and Fedorov2012; Cortijo et al. Reference Cortijo, Mus, Jensen and Palacios2015). Although the presence of these and broadly comparable genera in Cambrian rocks is rare (e.g. Yochelson & Stump, Reference Yochelson and Stump1977; McIlroy, Green & Brasier, Reference McIlroy, Green and Brasier2001; Rogov et al. Reference Rogov, Karlova, Marusin, Kochnev, Nagovitsin and Grazhdankin2015), they may be evolutionarily tied to tubular forms present in small shelly fossil assemblages of the lowest Cambrian, such as the anabaritids.
The lower Cambrian Kuanchuanpu fauna (Fortunian Stage, c. 535 Ma) in South China has become increasingly significant in understanding the evolutionary history of animals in the Ediacaran–Cambrian transition. Apart from small shelly fossils, including various molluscs and protoconodonts (Bengtson et al. Reference Bengtson, Conway Morris, Cooper, Jell and Runnegar1990; Qian, Reference Qian1999), the Kuanchuanpu Formation has yielded several extraordinary discoveries including putative arthropod embryos (Steiner et al. Reference Steiner, Zhu, Li, Qian and Erdtmann2004b ), scalidophorans (Liu et al. Reference Liu, Xiao, Shao, Broce and Zhang2014b ; Zhang et al. Reference Zhang, Xiao, Liu, Yuan, Wan, Muscente, Shao, Gong and Cao2015) and markedly diverse types of cnidarians (Han et al. Reference Han, Kubota, Uchida, Stanley, Yao, Shu, Li and Yasui2010, Reference Han, Kubota, Li, Yao, Yang, Shu, Li, Kinoshita, Sasaki, Komiya and Yan2013; Dong et al. Reference Dong, Cunningham, Bengtson, Thomas, Liu, Stampanoni and Donoghue2013; Han et al. Reference Han, Kubota, Li, Ou, Wang, Yao, Shu, Li, Uesugi, Hoshino, Sasaki, Kano, Sato and Komiya2016a ) with biomineralized exoskeletons. Particularly, the scalidophorans, molluscs and protoconodonts have established phylogenetic connections with the emerging complex Cambrian marine ecosystem, for example, the diversity of arthropods as represented by the Chengjiang fauna (Vannier et al. Reference Vannier, Steiner, Renvoise, Hu and Casanova2007, Reference Vannier, Garc A-Bellido, Hu and Chen2009). Perhaps more importantly, the abundant millimetre-scale tubular fossils in the Kuanchuanpu fauna at least superficially resemble the terminal Ediacaran tubular ecosystem (Fedonkin et al. Reference Fedonkin, Gehling, Grey, Narbonne and Vickers-Rich2007; Yang et al. Reference Yang, Steiner, Zhu, Li, Liu and Liu2016).
Here we describe a three-dimensionally preserved tubular microfossil–Feiyanella manica gen. et sp. nov.–from the lower Cambrian Kuanchuanpu Formation, Shaanxi Province, South China. It exhibits similar funnel-in-funnel tube construction to the late Ediacaran tubular fossil Cloudina (Hua et al. Reference Hua, Chen, Yuan, Zhang and Xiao2005), and also shows broadly comparable dichotomous branching features posited to indicate asexual reproduction. Further, it shows a similar wrinkled/folded tube wall exterior comparable to that of Sinotubulites (Chen et al. Reference Chen, Bengtson, Zhou, Hua and Yue2008; Cai et al. Reference Cai, Xiao, Hua and Yuan2015). This newly described organism may therefore provide an important palaeobiological and palaeoecological link between tubular fossils in the latest Ediacaran Period and earliest Cambrian Period.
2. Stratigraphic setting, fossil material and methods
The specimens described here were recovered from Bed 31 of the lower Cambrian Kuanchuanpu Formation (Fortunian Stage, Terreneuvian Series) at the Shizhonggou section of Ningqiang County, and Bed 2 at the Zhangjiagou section, Xixiang, Shaanxi Province, South China. The Ningqiang and Xixiang areas were palaeogeographically located on the northwestern margin of the Yangtze Platform during Ediacaran and Cambrian times. The fossil-bearing beds belong to the classic Anabarites trisulcatus – Protohertzina anabarica small shelly fossil biozone, indicating a stratigraphic equivalent of the Nemakit–Daldynian interval in Siberia (Steiner et al. Reference Steiner, Li, Qian and Zhu2004a ).
Insoluble phosphatized fossils were liberated from the phosphatic limestone using 10% acetic acid digestion. A Hyolithellus sp. specimen (ELIXX35-465) and a well-preserved specimen of Feiyanella manica gen. et sp. nov. (ELISN141-14) were imaged by scanning electron microscopy (SEM) (Fig. 1), and ELISN141-14 was three-dimensionally analysed using Synchrotron radiation X-ray tomographic microscopy (SRXTM) at SPring-8 in Hyogo, Japan (Figs 2–4). The 3D reconstructions of X-ray data were processed using VG Studio 2.2 Max, allowing us to document interior anatomic details of the tube structure. All specimens are reposited at the Early Life Institute (ELI), Northwest University, Xi'an, China.
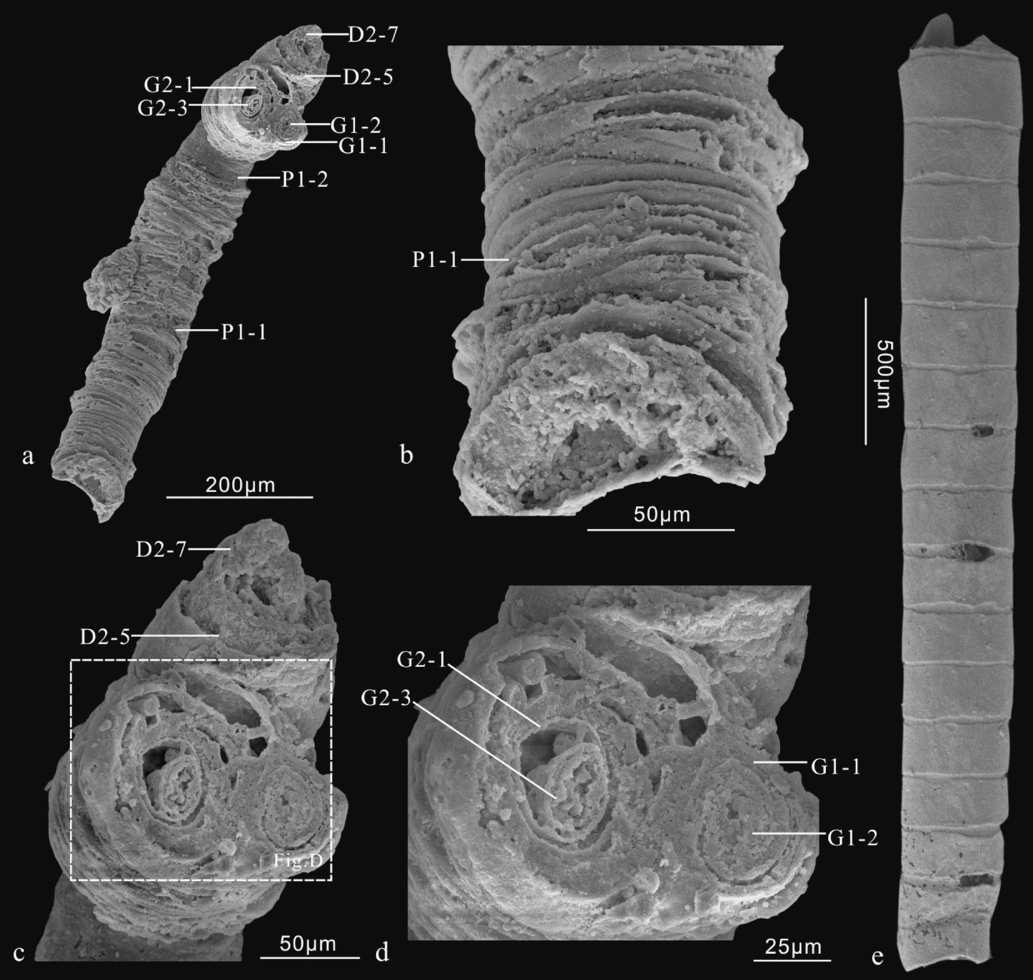
Figure 1. Secondary electron photomicrographs of Feiyanella manica gen. et sp. nov. (a–d) and Hyolithellus sp. (e). Fossils were recovered from the basal Cambrian Kuanchuanpu Formation at the Shizhonggou (a–d; Ningqiang County) and the Zhangjiagou (e; Xixiang County) sections. (a) Holotype specimen (ELISN141-14). Exterior view of the tube. (b) and (c) are enlarged views of the apical and apertural part, respectively. (d) is close up of (c). The tube is generally conical in shape, with the apical end (lower left) slightly tapering and the apertural end flaring (upper right) (a). The outermost layer of the tube is ornamented with transverse corrugations (b). Two daughter tubes (D1 and D2) and two granddaughter tubes (G1 and G2) can be seen in apertural fracture (c–d). (e) Hyolithellus sp. (ELIXX35-465). Abbreviations: P – parent tube; D – daughter tube; G – granddaughter tube. One parent tube (P1), two daughter tubes (D1 and D2) and three granddaughter tubes (G1, G2, and G3) are identified. Numberings suffixed P1, D1, D2, G1, G2 and G3 represent layers of walls in the parent, daughter and granddaughter tubes, respectively.

Figure 2. SRXTM virtual sections of the holotype specimen (ELISN141-14) of Feiyanella manica gen. et sp. nov. (a–d) Vertical bisections of the entire specimen; (e–m) transverse sections. Positions of the sections are indicated in (a). Abbreviations: St – soft tissue. For all other abbreviations, see Figure 1. Scale bars: 350 μm for (a–d) and 60 μm for (e–m).

Figure 3. SRXTM reconstructions of the holotype specimen (ELISN141-14) of Feiyanella manica gen. et sp. nov. (a) External view of entire specimen; (b–c) vertical bisection of the specimen; (d–j) transverse sections. Positions of the sections are indicated in (c). Abbreviations: see Figure 1. Scale bars: 250 μm for (a–c) and 60 μm bar (d–j).

Figure 4. SRXTM reconstruction of the holotype specimen (ELISN141-14) of Feiyanella manica gen. et sp. nov. Different tube layers are in different colours. The outer layers are stepwise removed (from a–l) to expose the inner layers. Abbreviations: see Figure 1. Scale bars: 400 μm for (a–d) and 150 μm for (e–l).
3. Systematic palaeontology
Incertae sedis
Genus Feiyanella new genus
Type species. Feiyanella manica new species, by monotypy
Diagnosis. Minute, multi-layered sub-cylindrical fossil tube consisting of a number of nested funnel-shaped layers. Outermost layer strongly wrinkled/folded, inner layers ornamented with weaker transverse annulations. Tube exhibits two orders of dichotomous branching, forming three generations of tubes. Parent tube layers are fully nested in the preserved length, whereas daughter and granddaughter tube layers are partially stacked/overlapped.
Feiyanella manica gen. et sp. nov.
Figures 1–5
Etymology. Feiyan (Feiyan Zhao), an ancient Chinese beauty famous for her slender build, similar to the slight gross appearance of the tube; manica, Latin, referring to the wrinkled outermost layer of the tube that resembles a folded shirt sleeve.

Figure 5. Schematic drawing of the cross-section of Feiyanella manica gen. et sp. nov. illustrating growth patterns between parent, daughter and granddaughter tubes. Note the orthogonal splitting planes between daughter (rightward arrow) and granddaughter (upward arrow) tubes. Abbreviations: see Figure 1.
Holotype. ELISN141-14
Type locality and horizon. The Shizhonggou section in Ningqiang County, Shaanxi Province, South China. Lower Cambrian Kuanchuanpu Formation (Fortunian Stage).
4. Description
The holotype specimen of Feiyanella manica gen. et sp. nov. (ELISN141-14) is three-dimensionally preserved through authigenic phosphatization. The fossil is incomplete (Fig. 1a), with both apical and apertural ends not intact (Fig. 1b–d). The preserved portion of the tube is roughly conical and gently curved (Fig. 1a). The apertural end flares with three slightly divergent, concentric sub-units (Fig. 1a, c, d). The tube is multi-layered and nested, composed of a number of stacked layers with varying overlap (Figs 1–5). The outermost layer of the tube is corrugated with closely spaced transverse ridges, showing stronger folds or wrinkles (Figs 1a, b, 2a–d, 3a–c). Only weaker transverse annulations can be seen in inner layers (Fig. 4b–l). SRXTM analysis reveals that three units of tube layers with unambiguously different lengths and diameters can be identified in the holotype specimen. Although these three sets of tubes are of quite different sizes, they all show the funnel-in-funnel tube construction. They are here interpreted as representing three generations – namely the parent, the daughter and the granddaughter tubes, respectively – which are described separately below.
4.a. Parent tube
The parent tube is sub-cylindrical in gross morphology, with the apertural end slightly flaring. It consists, from exterior to interior, of four nested funnel-shaped layers (the outermost four layers of the tube of Feiyanella, marked with P1-1, P1-2, P1-3, P1-4 in all figures). The preserved length of the parent tube is c. 1379 µm (= the length of the longest third layer; P1-3). The four layers of funnels, ranging from 193 to 304 µm in diameter, are fully stacked and overlapped, reminiscent of the ‘tube-in-tube’ construction of late Ediacaran tubular fossil Sinotubulites (Cai et al. Reference Cai, Xiao, Hua and Yuan2015). The first layer (P1-1) is strongly wrinkled and/or folded, forming stronger, closely spaced, irregular, transverse corrugations on the exterior surface of the tube (Fig. 1a, b), strongly distinct from those on the inner layers. Transverse corrugations often bear a few secondary transverse irregular folds (Fig. 1b), which form complex exterior corrugations and make this layer appear to be much thicker than any other layers (Figs 2a–d, 3b, c). The notably stronger corrugation on the outermost layer of Feiyanella is a diagnostic feature characterizing this taxon. The second, third and fourth parent layers (P1-2–P1-4) share a similar morphology with the first layer. However, layers P1-2–P1-4 are ornamented with weaker transverse annulations (Fig. 4b–d), quite different from those in layer P1-1. The second parent layer is c. 120 and c. 259 µm in minimum and maximum diameters, respectively. The third parent layer (P1-3) is the longest one (1379 µm) in the preserved specimen (Fig. 4c). The fourth parent layer is obviously shorter (631 µm) and smaller (77 and 108 µm in minimum and maximum diameters) than the other three parent funnels (Fig. 4d).
4.b. Daughter tube
Two daughter tubes (denoted as D1 and D2 in all figures) are discernible at the apertural end of the tube of Feiyanella (Figs 1c, 2, 3, 4), which extend from the apertural opening of the fourth parent funnel (Fig. 4d). The two daughter tubes stand side-by-side, and the outermost funnel of each daughter tube appears nearly cemented together (Fig. 2e). The daughter tubes consist of a number of nested, funnel-shaped layers, sharing similarities in tube wall morphology and nesting patterns with the parent tube. The two daughter tubes are not equal in size. The larger one (D1) consists of two tube wall layers (Fig. 2f) and is irregular in cross-section (Fig. 2e–g). The two layers (D1-1 and D1-2) are only situated in the apertural part of Feiyanella. Its width increases greatly towards the apertural end (Fig. 4a–d). The smaller daughter tube (D2) is composed of seven tube wall layers (D2-1–D2-7 in Figs 2d–j, 3b–c, 4d–f). In contrast, ornamentations on the daughter funnels are noticeably diminished, with closely spaced transverse annulations but without complicated corrugations (e.g. funnel D2-1), as compared to the parent funnels.
4.c. Granddaughter tube
Four granddaughter tubes are identified in Feiyanella: two (G1 and G2) originated from the larger daughter tube (D1) and the other two (G3 and G4) from the smaller daughter tube (D2). The granddaughter tubes G1 and G2 are situated at the upper half of the preserved parent tube (Fig. 3b, c), whereas G3 and G4 are at the apertural end (Figs 2e, 3d). Morphological details of G3 and G4 are very limited, as they can only be identified in cross-sections near the apertural end of the tube (Figs 2e, 3d). Granddaughter tubes G1 and G2 are deeply nested into the daughter tube D1 (located at the middle to apertural portion of the parent tube; Fig. 2e–k). G1 and G2 are more or less equal in size (c. 39 µm). Notably, the splitting plane along the tube between the two granddaughter tubes G1 and G2 is orientated perpendicular to the splitting plane of the daughter tubes (Figs 2e, 5). G1 contains two layers of funnels (G1-1 and G1-2) and G2 has three layers (G2-1–G2-3) (Fig. 4d–i). The innermost layer of the granddaughter tube G2 (G2-3) displays four lobes separated by four longitudinal furrows in cross-section (Figs 2i, j, 3g). This tetraradial symmetry only occurs at the apical end, and traverses approximately one-fourth of the granddaughter tube G2 (Fig. 4g–i). A spindle-shaped structure was preserved in the innermost funnel of the granddaughter tube G1 (denoted as St in Figs 2b, h, i, 3g, 4g). It is situated in the central portion of the granddaughter tube G1 and is oval (Fig. 2h, i) in cross-section. The spindle exhibits a bright phase under SRXTM observation, c. 39 µm in diameter and 188 µm in length, occupying nearly one-third of the full length of granddaughter tube G1 (Fig. 4g).
5. Discussion
The incomplete preservation of the Feiyanella tube leaves uncertainties as to its full morphology and nesting patterns of the parent layers. Two overlapping patterns can be identified from the preserved portion, however: the outer parent tube layers (P1-1–P1-4) fully overlap (Fig. 4a–d), whereas all of the other inner tube layers only partially overlap (Fig. 4d–l). Two contrasting degrees of ornamentation are observed in Feiyanella: the outer parent tube layers (P1-1–P1-4) are visibly folded/wrinkled and form stronger transverse and/or slightly oblique corrugations (Fig. 4a–d), whereas all of the other inner tube layers are ornamented with weaker transverse annulations (Fig. 4d–l). The outermost layer of the tube in particular shows strongly folded/wrinkled corrugations (Figs 1a, b, 3a, 4a), making this layer appear much thicker (Figs 2a–d, 3b–c). With regard to nesting, although the four funnel-shaped parent layers (P1-1–P1-4) are completely nested in the preserved portion of the Feiyanella tube, it is uncertain whether their full morphology is funnel-shaped and whether they are fully or partially stacked.
As an Örsten-type Lagerstätte, the Kuanchuanpu Formation biota is characterized by the selective preservation of refractory cuticular tissues of meiofauna (see review in Schiffbauer et al. Reference Schiffbauer, Wallace, Broce and Xiao2014) and only fragments of larger organisms (i.e. putative grasping spines of Protohertzina anabarica; see Steiner et al. Reference Steiner, Li, Qian and Zhu2004a ). However, Feiyanella was not likely a fragment of a more complex organism: for example, an appendage of an annelid or ecdysozoan (panarthropod cycloneuralian). Several lines of evidence are summarized here: (1) Both annelids and ecdysozoans have a cuticularized integument overlying the epidermis, particularly a tri-layered cuticle (epi-, exo- and endocuticles) in cycloneuralians (Bereiter-Hahn, Matoltsy & Richards, Reference Bereiter-Hahn, Matoltsy and Richards1984; Peterson & Eernisse, Reference Peterson and Eernisse2001). The appendages of these animals, if fossilized, would not be preserved as loosely multi-layered, funnel-in-funnel structures. (2) The cuticle of a complex organism usually bears complex ornaments, such as sensory organs, chaetae, glands, scalids and net-like structures. These ornaments are absent in Feiyanella. (3) The branched, segmented appendages in arthropods differ from the unsegmented funnel of Feiyanella. (4) The parapodium of annelids, although varying among species, tapers apparently towards one end, in visible contrast with the sub-cylindrical shape of Feiyanella. (5) Some species of cycloneuralians (Liu et al. Reference Liu, Xiao, Shao, Broce and Zhang2014b ; Zhang et al. Reference Zhang, Xiao, Liu, Yuan, Wan, Muscente, Shao, Gong and Cao2015) have been discovered from the Kuanchuanpu Formation. These fossils, together with a large number of specimens in our collection, collectively exhibit specific cuticle ornaments that are markedly different from Feiyanella. In addition, the integument of the cycloneuralian fossils is preserved as a single layer. In short, the characteristic multi-layered, funnel-in-funnel structure of Feiyanella is more appropriately interpreted as a dwelling tube of an organism, comparable to those known from the upper Ediacaran (e.g. Hua, Pratt & Zhang, Reference Hua, Pratt and Zhang2003; Cai et al. Reference Cai, Schiffbauer, Hua and Xiao2011, Reference Cai, Hua, Schiffbauer, Sun and Yuan2014, Reference Cai, Xiao, Hua and Yuan2015), rather than a body fragment of a larger complex organism.
Feiyanella is distinct from other early Cambrian tubular fossils, i.e. Hyolithelminths, Byronia and Sphenothallus. Hyolithelminths, a problematic group of mineralized tubular fossils characterized by more or less regular annulations or growth lines (Bengtson, et al. Reference Bengtson, Conway Morris, Cooper, Jell and Runnegar1990; Li, Reference Li2004), exhibit a multi-laminated tube wall, and in some species, a cone-in-cone structure (see Kouchinsky et al. Reference Kouchinsky, Bengtson, Clausen and Vendrasco2015, fig. 67). However, the micro-laminations of the tube wall are closely cemented together and each lamella (in some species) is composed of fine fibres (i.e. Bengtson et al. Reference Bengtson, Conway Morris, Cooper, Jell and Runnegar1990; Vinn, Reference Vinn2006; Skovsted & Peel, Reference Skovsted and Peel2011). Hyolithellus from the Kuanchuanpu Formation – a common element in the Kuanchuanpu Formation in the Xixiang and Ningqiang areas Steiner et al. Reference Steiner, Li, Qian and Zhu(2004a) – is another tubular fossil sharing a similar morphology with Feiyanella. But the tubes of Hyolithellus are much larger in size (> 2 mm in length) than Feiyanella and the exterior smooth surface bears a number of wide-spaced circular grooves (Fig. 1e). In addition, ellipse-shaped pores occasionally occur in the grooves, which may be produced by soft tissue inside the tubes. Hyolithellus may have been a more complex organism (e.g. an annelid; Skovsted & Peel, Reference Skovsted and Peel2011). The smooth tube, transverse grooves and ellipse-shaped pores indicate contrasting morphological and palaeobiological relationships with Feiyanella.
Byronia, a sessile tube-dwelling organism characterized by a lenticular cross-section and a deep split along the broader end (Bengtson et al. Reference Bengtson, Conway Morris, Cooper, Jell and Runnegar1990), differs from Feiyanella in its wide-spaced transverse ridges and longitudinal ribs (see fig. 6 in Skovsted & Peel, Reference Skovsted and Peel2011) that resemble the peridermal tube of olivooids (e.g. Olivooides and Quadrapyrgites; Liu et al. Reference Liu, Li, Shao, Zhang, Wang and Qiao2014a ; Steiner et al. Reference Steiner, Qian, Li, Hagadorn and Zhu2014) from the Kuanchuanpu Formation. Both Byronia and olivooids have been proposed to have close affinities with thecate medusozoans in the phylum Cnidaria (see Bengtson et al. Reference Bengtson, Conway Morris, Cooper, Jell and Runnegar1990; Zhu et al. Reference Zhu, Van Iten, Cox, Zhao and Erdtmann2000; Dong et al. Reference Dong, Cunningham, Bengtson, Thomas, Liu, Stampanoni and Donoghue2013; Han et al. Reference Han, Li, Kubota, Ou, Toshino, Wang, Yang, Uesugi, Hoshino, Sasaki, Kano and Komiya2016b ).
Sphenothallus, a cone-shaped tube characterized by a more or less elliptical cross-section and a basal holdfast and proposed as a thecate medusozoan (Van Iten, Cox & Mapes, Reference Van Iten, Cox and Mapes1992; Zhu et al. Reference Zhu, Van Iten, Cox, Zhao and Erdtmann2000; Li, Reference Li2004), differs from Feiyanella in its finely laminated and cemented tube wall consisting of alternating apatite and organic laminae.
Instead, Feiyanella shares similarities in tube morphology and construction with some late Ediacaran tubular fossils, including Cloudina (Hua et al. Reference Hua, Chen, Yuan, Zhang and Xiao2005; Cortijo et al. Reference Cortijo, Mus, Jensen and Palacios2010, Reference Cortijo, Mus, Jensen and Palacios2015), Conotubus (Cai et al. Reference Cai, Schiffbauer, Hua and Xiao2011) and Sinotubulites (Chen et al. Reference Chen, Bengtson, Zhou, Hua and Yue2008; Cai et al. Reference Cai, Xiao, Hua and Yuan2015). Each of these taxa shows a nested tube construction, cylindrical or oval-shaped cross-section and the absence of transverse internal structures such as septae or tabulae.
The perceived nesting pattern and the wrinkled tube-wall features of the parent layers (particularly P1-1) of Feiyanella are visibly similar to those of Sinotubulites (Cai et al. Reference Cai, Xiao, Hua and Yuan2015). This may indicate that the parent layers of Feiyanella fully overlap, which would thus be comparable to the nesting patterns of Sinotubulites. On the other hand, the funnel-shaped tube layers of Feiyanella are strikingly similar to those of Cloudina (Hua, Pratt & Zhang, Reference Hua, Pratt and Zhang2003; Hua et al. Reference Hua, Chen, Yuan, Zhang and Xiao2005; Cortijo et al. Reference Cortijo, Mus, Jensen and Palacios2010) and Conotubus (Cai et al. Reference Cai, Schiffbauer, Hua and Xiao2011). Furthermore, the dichotomous branching of Feiyanella, which is most likely indicative of an asexual reproduction strategy, is also comparable to that of Cloudina (Hua et al. Reference Hua, Chen, Yuan, Zhang and Xiao2005; Cortijo et al. Reference Cortijo, Mus, Jensen and Palacios2010, Reference Cortijo, Mus, Jensen and Palacios2015).
While similar, Feiyanella also shows distinct differences from Cloudina, Conotubus and Sinotubulites. First, as compared to Sinotubulites, Feiyanella differs in tube layer morphology and overlapping patterns: Feiyanella is composed exclusively of funnel-shaped layers with partial overlap between adjacent layers (except for those of the parent funnels), whereas Sinotubulites is composed exclusively of cylinder-shaped layers with full overlap between two adjacent layers. The funnels of Feiyanella differ from the funnels of Cloudina and Conotubus in that the former lacks thickened rims on the apical and apertural ends of the funnels (Hua et al. Reference Hua, Chen, Yuan, Zhang and Xiao2005; Cortijo et al. Reference Cortijo, Mus, Jensen and Palacios2010; Cai et al. Reference Cai, Schiffbauer, Hua and Xiao2011). In addition, Feiyanella differs from Cloudina in the manner of asexual reproduction. Cloudina is characterized by two manners of asexual reproduction strategies: dichotomous branching of daughter tubes in the same parent tube (see fig. 1L of Hua et al. Reference Hua, Chen, Yuan, Zhang and Xiao2005 and fig. 8 of Cortijo et al. Reference Cortijo, Mus, Jensen and Palacios2010, Reference Cortijo, Mus, Jensen and Palacios2015) and budding of a daughter tube between two adjacent funnels (see fig. 1P of Hua et al. Reference Hua, Chen, Yuan, Zhang and Xiao2005). Feiyanella, however, displays a pattern of multiple-ordered dichotomous branching of the younger generation of tubes within the older generation tubes.
Most broadly, Feiyanella shares similarities in the tube morphology, nesting patterns and presence of asexual reproduction strategies with Cloudina, and in the corrugation and nesting patterns of outer layers with Sinotubulites. Considering its similarities with Cloudina (Cai et al. Reference Cai, Hua, Schiffbauer, Sun and Yuan2014) and Conotubus (Cai et al. Reference Cai, Schiffbauer, Hua and Xiao2011), Feiyanella is thus interpreted as a sessile organism with periodic growth by secretion of new funnels within the older funnels (Grant, Reference Grant1990), with the animal presumed to have lived within the innermost, most recently secreted funnel (Cortijo et al. Reference Cortijo, Mus, Jensen and Palacios2010). The tube of Feiyanella is hollow, indicating the soft tissue of the organism likely was able to move up and down within the tube, rather than being fixed within an isolated chamber. Although the full morphology of the tube is unknown, the apical end may have been closed, comparable to the basally closed apical end of Cloudina (Cortijo et al. Reference Cortijo, Mus, Jensen and Palacios2015).
6. Zoological affinities and evolutionary implications
Together, Cloudina, Conotubus, Sinotubulites and Feiyanella may constitute a monophyletic group, as they are morphologically quite different from other Ediacaran and Cambrian tubular fossils (see summary in Cai et al. Reference Cai, Schiffbauer, Hua and Xiao2011; Cai, Hua & Zhang, Reference Cai, Hua and Zhang2013). The zoological placement of these Cloudina-type organisms, however, has been controversial. For example, Cloudina has been compared with both serpulid annelids (e.g. Glaessner, Reference Glaessner1976; Hua et al. Reference Hua, Chen, Yuan, Zhang and Xiao2005) and cnidarians (e.g. Vinn & Zaton, Reference Vinn and Zaton2012; Van Iten et al. Reference Van Iten, Marques, Leme, Pacheco and Sim Es2014); the lack of preserved soft tissues in these fossils hinders convincing establishment of a palaeobiological interpretation.
While not reported in any other similar fossils, the spindle-shaped structure reported here in the innermost funnel of the granddaughter tube G1 may help provide a clue for phylogenetic placement of Feiyanella and, by extension, potentially other Cloudina-type organisms. This structure is three-dimensionally replicated by phosphate minerals, and its spindle-shaped morphology and massive size compared to the tube volume does not support an interpretation of an incompletely preserved funnel or other mineralized structure. Instead, we suggest that the spindle-shaped mass may be the phosphatized remains of the long-awaited tube dweller. Although it is difficult to depict the full morphology of the soft tissue inside the hollow tube, this spindle-shaped structure may represent the degraded organic remains of the organism that lived within these tubes. This interpretation is consistent with the morphological and palaeoecological reconstruction in Conotubus and Cloudina (Cai et al. Reference Cai, Schiffbauer, Hua and Xiao2011, Reference Cai, Hua, Schiffbauer, Sun and Yuan2014); they all possess hollow tubes which allowed for the tube dwellers to move up and down freely. This interpretation is also in accordance with the periodic growth of the tubes; the funnels were not simultaneously secreted by the soft issue, but instead were episodically secreted in the innermost layers.
A cnidarian planula interpretation for Feiyanella is largely compatible, and can be supported by several lines of evidence: (1) The asexual reproduction by longitudinal and transverse fissions of the soft body is more popular in extant cnidarians than in bilaterians. In addition, transverse fission has been reported from the sea-anemone-like cnidarians in the lower Cambrian Kuanchuanpu Formation in South China (Han et al. Reference Han, Kubota, Uchida, Stanley, Yao, Shu, Li and Yasui2010). (2) The corrugated outermost layer of the tube broadly resembles the periderm of medusozoan polyps (Werner, Reference Werner1973; Jarms, Reference Jarms1991). (3) The four-lobed morphology seen in granddaughter tube G2 of Feiyanella shows symmetry comparable to another coeval fossil, Carinachites spinatus Qian, Reference Qian1977 (Qian, Reference Qian1977; Conway Morris & Chen, Reference Conway Morris and Chen1992) – a Cambrian medusozoan with a phosphatized skeleton showing triradial, tetraradial and pentaradial symmetries (Conway Morris & Chen, Reference Conway Morris and Chen1992; Qian et al. Reference Qian, Van Iten, Cox, Zhu and Zhou1997; Liu et al. Reference Liu, Li, Shao, Zheng, Zheng, Wang, Wang and Qwang2011). (4) A colonial life habit proposed for Feiyanella is common in cnidarian hydropolyps (Hyman, Reference Hyman1940) (5) The funnel-in-funnel tube architecture of Feiyanella has also been observed in a few hydrotheca (e.g. Sertularelia quadrata; plates 15 and 16 of Nutting, Reference Nutting1900). (6) The proposed closed apical end of Feiyanella is compatible with a cnidarian body plan, but inconvenient for defecating of a bilateral worm with a through gut.
The ontogeny of Feiyanella indicates a possible transition from cylindrical radial symmetry to tetraradial symmetry. A similar transition from cylindrical radial symmetry to triradial symmetry was observed from a coeval fossil, Anabarites, which is also an early Cambrian tubular small shelly fossil suspected to be a cnidarian (Kouchinsky et al. Reference Kouchinsky, Bengtson, Feng, Kutygin and Val'kov2009).
It is well known that Cambrian communities are quite different from those of the Ediacaran Period. Cambrian communities, exemplified by the Chengjiang and Burgess Shale biotas, are characterized by a complex food web with diverse types of feeding behaviours (Vannier et al. Reference Vannier, Steiner, Renvoise, Hu and Casanova2007; Hou, Siveter & Aldridge, Reference Hou, Siveter and Aldridge2008) and complex reproduction strategies (Duan et al. Reference Duan, Han, Fu, Zhang, Yang, Komiya and Shu2014; Caron & Vannier, Reference Caron and Vannier2015) similar to modern ocean ecosystems. Ediacaran communities, however, were instead comprised of slow creeping mat feeders and sessile benthic suspension feeders and/or osmotrophic feeders (e.g. Fedonkin et al. Reference Fedonkin, Gehling, Grey, Narbonne and Vickers-Rich2007; Laflamme, Xiao & Kowalewski, Reference Laflamme, Xiao and Kowalewski2009; Rahman et al. Reference Rahman, Darroch, Racicot and Laflamme2015; Wood & Curtis, Reference Wood and Curtis2015). Although the early Cambrian small shelly fossil community – exemplified by the Kuanchuanpu Formation organisms – contains mat feeders (molluscs) (Qian & Bengtson, Reference Qian and Bengtson1989) and microscopic predators (cycloneurians) (Liu et al. Reference Liu, Xiao, Shao, Broce and Zhang2014b ; Zhang et al. Reference Zhang, Xiao, Liu, Yuan, Wan, Muscente, Shao, Gong and Cao2015), this ecosystem was characterized by a high richness and diversity of sessile organisms (i.e. predominantly cnidarians). Although a zoological placement remains unresolved, Feiyanella represents a Cambrian relict containing significant similarities in tube morphology, tube construction and reproduction strategy to some terminal Ediacaran tubular fossils (e.g. Cloudina, Conotubus and Sinotubulites). The early Cambrian small shelly fossil community revealed in the Kuanchuanpu Formation exhibits palaeobiological and palaeoecological features seemingly intermixed between both late Ediacaran and early Cambrian faunas. As such, it may support the notion that the late Ediacaran tubular biotas and their descendants represent important elements in a broader evolutionary prelude of the Cambrian explosion (Shu et al. Reference Shu, Isozaki, Zhang, Han and Maruyama2014; Schiffbauer et al. Reference Schiffbauer, Huntley, O'Neil, Darroch, Laflamme and Cai2016), rather than disappearing from the fossil record in an end-Ediacaran extinction (Smith et al. Reference Smith, Nelson, Strange, Eyster, Rowland, Schrag and Macdonald2016).
7. Conclusions
The early Cambrian tubular fossil Feiyanella manica gen. et sp. nov. shares morphological similarities with the late Ediacaran tubular fossils Cloudina, Conotubus and Sinotubulites. Together, these tubular organisms may constitute a monophyletic group in the late Ediacaran through early Cambrian periods. The ‘funnel-in-funnel’ tube construction and the two-ordered dichotomous branching of Feiyanella manica gen. et sp. nov. are all comparable to those reported in Cloudina. The strongly corrugated outermost funnel and the gradational variations in the degrees of folding and/or wrinkling from exterior to interior tube layers of Feiyanella manica gen. et sp. nov. resembles those of Sinotubulites. Feiyanella thus embraces evolutionary continuity of typical late Ediacaran ‘funnel-in-funnel’ tube construction (exemplified by Cloudina and Conotubus), asexual reproduction by dichotomous branching of the tube (exemplified by Cloudina) and a gradational decrease in degrees of wrinkles and/or ornamentation from exterior to interior layers (exemplified by Sinotubulites). These continuities provide pivotal palaeobiological and palaeoecological insights into our understanding of the evolutionary history of the Ediacaran–Cambrian transition.
Acknowledgements
We thank H. Gong, J. Luo, N. Liu and M. Cheng (State Key Laboratory for Continental Dynamics, Northwest University (NWU), Xi'an, China) for their assistance in both field and lab works. We are grateful to Prof. Li Guoxiang (Nanjing Institute of Geology and Palaeontology, Chinese Academy of Sciences) for invaluable advice. Current work was supported by the Natural Science Foundation of China (Nos. 41621003, 41272019 and 41572012), the ‘973 project’ of the Ministry of Science and Technology of China (2013CB835002), and the State Key Laboratory of Palaeobiology and Stratigraphy (Nanjing Institute of Geology and Palaeontology, CAS) (No. 163107).