1. Introduction
The Albian Age (112.0–99.6 Ma, Gradstein, Ogg & Smith, Reference Gradstein, Ogg and Smith2004) is generally viewed as a time characterized by a long-term increase of global temperatures, finally culminating in the Late Cretaceous thermal maximum (Huber, Hodell & Hamilton, Reference Huber, Hodell and Hamilton1995; Pucéat et al. Reference Pucéat, Lécuyer, Sheppard, Dromart, Reboulet and Grandjean2003; Forster et al. Reference Forster, Schouten, Baas and Sinninghe Damsté2007). Stratigraphically well-calibrated marine proxy records provide evidence for significant short-term fluctuations in tropical and boreal ocean water temperatures, which occur superimposed on the general warming trend during Albian times (Clarke & Jenkyns, Reference Clarke and Jenkyns1999; Bice et al. Reference Bice, Birgel, Meyers, Dahl, Hinrichs and Norris2006; Erbacher et al. Reference Erbacher, Friedrich, Wilson, Lehmann and Weiss2011). Furthermore, deposition in the Tethys and the evolving North Atlantic Ocean was characterized by the repeated formation of organic-carbon-rich deposits, particularly during earliest and latest Albian times, known as Oceanic Anoxic Events 1b and d, respectively (e.g. Wilson & Norris, Reference Wilson and Norris2001; Herrle et al. Reference Herrle, Pross, Friedrich and Hemleben2003). These short-lasting events had a profound impact on marine biogeochemical cycles and were often associated with significant temperature anomalies (Erbacher et al. Reference Erbacher, Huber, Norris and Markey2001; Wagner et al. Reference Wagner, Wallmann, Herrle, Hofmann and Stuesser2007; Hofmann et al. Reference Hofmann, Stuesser, Wagner, Schouten and Sinninghe Damsté2008). Major marine bio-events taking place in the course of the Albian affect planktonic foraminifera, radiolarians and calcareous nannofossils (Erbacher, Thurow & Littke, Reference Erbacher, Thurow and Littke1996; Tiraboschi, Erba & Jenkyns, Reference Tiraboschi, Erba and Jenkyns2009; Huber & Leckie, Reference Huber and Leckie2011). On land, this time interval was associated with the rising dominance of angiosperms in terrestrial ecosystems (Crane, Friis & Pedersen, Reference Crane, Friis and Pedersen1995). The radiation and diversification of early angiosperms during Albian times had a strong impact on the coexisting plant communities, clearly visible in the concomitant decline of gymnosperm pollen and pteridophyte spores (Crane & Lidgard, Reference Crane and Lidgard1989).
Compared to the marine realm, information on the climatic conditions prevailing on the continents during Albian times is relatively scarce. This results mainly from the difficulties associated with the stratigraphic assignment of Lower Cretaceous continental deposits, essentially caused by the lack of suitable age-diagnostic marker fossils. Furthermore, correlation with stratigraphically well-constrained marine strata is hampered by the frequent occurrence of hiatuses, highlighting the incompleteness of the continental sedimentary record. However, some of these problems can be circumvented by studying near-shore marine deposits, which provide terrestrial climate signals in the form of spore-pollen assemblages and clay minerals.
The stratigraphic assignment of shallow marine palynomorph-bearing deposits can be significantly improved by the use of Sr-isotope stratigraphy (Burla et al. Reference Burla, Oberli, Heimhofer, Wiechert and Weissert2009). Sr-isotope values of low-Mg calcite fossils (e.g. brachiopods, belemnites, bivalves) reflect past seawater 87Sr/86Sr ratios (e.g. Jones et al. Reference Jones, Jenkyns, Coe and Hesselbo1994; Veizer et al. Reference Veizer, Ala, Azmy, Bruckschen, Buhl, Bruhn, Carden, Diener, Ebneth, Godderis, Jasper, Korte, Pawellek, Podlaha and Strauss1999; Korte et al. Reference Korte, Kozur, Bruckschen and Veizer2003). Hence, temporal fluctuations in 87Sr/86Sr ratios can serve as an independent chronostratigraphic tool. In the last couple of years, the 87Sr/86Sr ratios of well-preserved rudist shells have been successfully utilized to date Cretaceous shoal-water carbonate platform deposits lacking accurate biostratigraphic constraints (Steuber et al. Reference Steuber, Rauch, Masse, Graaf and Malkoč2005; Huck et al. Reference Huck, Heimhofer, Rameil, Bodin and Immenhauser2011). In more detritus-influenced depositional systems, the use of 87Sr/86Sr ratios from oyster shell low-Mg calcite represents a valuable alternative. A number of studies have demonstrated that oyster shells represent a reliable carrier of the Mesozoic seawater Sr-isotope signal (Jones et al. Reference Jones, Jenkyns, Coe and Hesselbo1994; Denison et al. Reference Denison, Miller, Scott and Reaser2003; Burla et al. Reference Burla, Oberli, Heimhofer, Wiechert and Weissert2009; Schneider, Fürsich & Werner, Reference Schneider, Fürsich and Werner2009) and therefore hold a significant potential for the stratigraphic refinement of Mesozoic near-shore successions.
The Lusitanian Basin of western Portugal is a key area for the study of early angiosperm remains. Here, the discovery of a series of exceptionally preserved angiosperm mesofossil floras in the northern part has strongly influenced the current understanding of the evolutionary relationships and biology of early angiosperms (Friis, Pedersen & Crane, Reference Friis, Pedersen and Crane2010) (Fig. 1). In contrast, the southern part of the basin bears near-shore marine deposits, which contain a well-preserved late Early Cretaceous palynoflora including a rich angiosperm pollen assemblage (Groot & Groot, Reference Groot and Groot1962; Medus & Berthou, Reference Medus and Berthou1980; Medus, Reference Medus1982; Trincão, Reference Trincão1990; Heimhofer et al. Reference Heimhofer, Hochuli, Burla, Dinis and Weissert2005, Reference Heimhofer, Hochuli, Burla and Weissert2007). Together with the classical Potomac record (Doyle & Robbins, Reference Doyle and Robbins1977; Hochuli, Heimhofer & Weissert, Reference Hochuli, Heimhofer and Weissert2006), the Portuguese angiosperm pollen records from the Rodízio and Galé formations are regarded as a reference succession for the palynological changes observed in mid-latitude angiosperm palynofloras during Albian times (Field, Chatelet & Brodribb, Reference Feild, Chatelet and Brodribb2009).
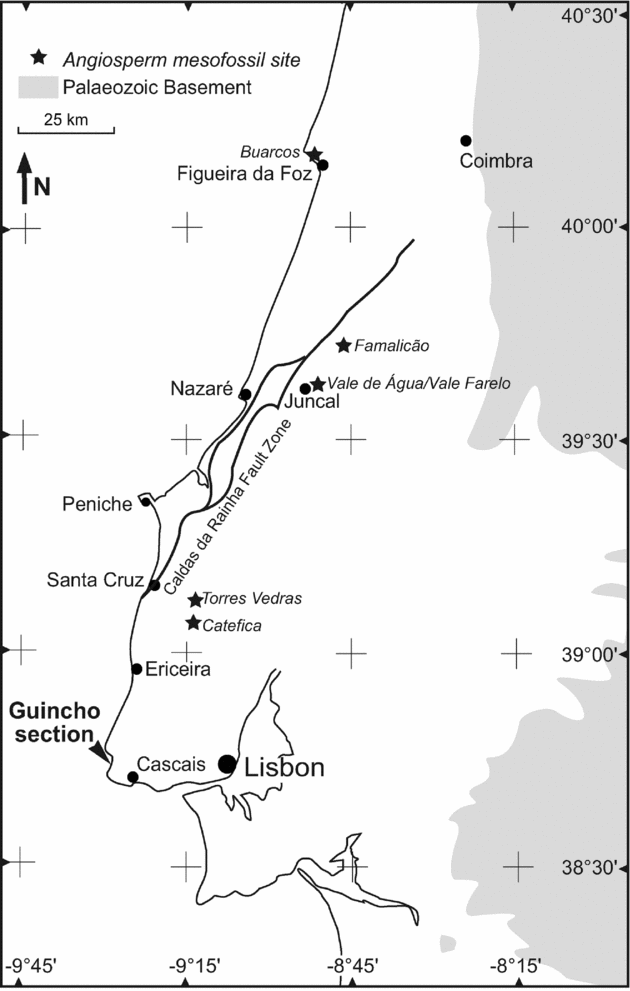
Figure 1. Map of western Portugal showing the location of the coastal outcrop of the Guincho section. Asterisks correspond to sites bearing Early Cretaceous angiosperm mesofossils. Map modified after Heimhofer et al. (Reference Heimhofer, Hochuli, Burla and Weissert2007) and Friis, Pedersen & Crane (Reference Friis, Pedersen and Crane2010).
The aim of this study is twofold: (1) Establish a refined stratigraphic framework using Sr-isotope stratigraphy based on low-Mg calcite oyster shells for the Albian deposits of the Guincho section; (2) Provide information on the floral community structure and on the climatic history of the area based on total spore-pollen associations and clay mineral data. The integration of the new stratigraphic and palaeoclimatic findings with existing results on the angiosperm palynoflora will provide a better understanding of the temporal and palaeoclimatic context under which the Albian diversification of angiosperms took place.
2. Geological setting
Lower Cretaceous sediments are well exposed in coastal outcrops of the Lusitanian Basin of Portugal. During Mesozoic times, the opening of the Atlantic Ocean resulted in the formation of a passive margin in western Iberia. In late Early Cretaceous times, thermally-induced basement uplift and isostatic adjustment led to the development of a basin-wide break-up unconformity in the Lusitanian Basin, followed by an episode of continental siliciclastic deposition directly overlying the unconformity (Dinis & Trincão, Reference Dinis and Trincão1995). The subsequent long-term eustatic rise allowed the re-establishment of near-shore marine conditions with deposition of mixed carbonate-siliciclastic sediments that are the focus of this study.
The sedimentary succession investigated covers more than 125 m of Albian strata and is accessible along the cliffs north of Cabo Raso, about 5 km northwest of the village of Cascais (Fig. 1). Here, an essentially continuous section (referred to as Guincho section) crops out along the beach of Praia de Água Doce between the Forte de Cresmina and the Ponta da Galé (Rey, Bilotte & Peybernes, Reference Rey, Bilotte and Peybernes1977; Rey, Reference Rey1992).
Following Rey, Bilotte & Peybernes (Reference Rey, Bilotte and Peybernes1977) and Rey (Reference Rey1992, Reference Rey2006) the section covers the Rodízio and the Galé formations with the latter being subdivided into two major lithological units, the Água Doce and Ponta da Galé members (Fig. 2). The Rodízio Formation covers the lowermost part of the section (0–29 m) and consists of thick-bedded and coarse-grained siliciclastic sediments with thin clayey and lignite-rich interbeds. At the studied locality, two fining-upward cycles can be differentiated, which consist of fluvial large-scale cross-bedded deposits, starting with conglomerates and passing into sandstones. The 18 to 22 m part of the section is currently not cropping out, being covered by beach sands.

Figure 2. Simplified lithological log and lithostratigraphy of the Guincho section plotted against sequence-stratigraphy, first- and last occurrences of age-diagnostic dinoflagellate cysts and Sr-isotope ratios derived from oyster shell calcite. Both the previous (left-hand column) and the revised age assignment (right-hand column) are shown. Sequence-stratigraphy based on Dinis, Rey & de Graciansky (Reference Dinis, Rey and de Graciansky2002) and Rey (Reference Rey2006); biostratigraphy based on Heimhofer et al. (Reference Heimhofer, Hochuli, Burla and Weissert2007). PdG – Ponta da Galé Member.
The upper part of the Rodízio Formation, making the transition to the Água Doce Member of the Galé Formation, is characterized by a succession (22–29 m) of reddish and grey sand- and siltstones alternating with purple to greenish claystones interpreted to reflect deposition in peritidal and/or coastal settings (Dinis, Rey & de Graciansky, Reference Dinis, Rey and de Graciansky2002). This siliciclastic-dominated interval is overlain by the Água Doce Member, formed between 29 m and 71 m by fossiliferous inner shelf marls and sandy limestones, which alternate with varicoloured mudstones and sparse silt- or sandstone beds. The marls and limestones are strongly bioturbated and characterized by a rich macrofossil assemblage including oysters, gastropods, serpulids, thick-shelled bivalves and orbitolinids. Within this interval, a unit composed of thin-bedded ochre-coloured sand- and siltstones can be distinguished (43.5 m to 45.5 m). Abundant ripple structures and a fine lamination pattern as well as the occurrence of highly oxidized wood fragments point to deposition in a shallow, high-energy environment.
At ~ 71 m, the lithologically heterogeneous lower part of the Água Doce Member passes into inner shelf marls and limestones. The subsequent series (71–97.5 m) is dominated by thick-bedded and fossil-rich limestones, alternating with more marly or sand-rich interbeds. The limestones have a very distinct nodular appearance, most probably caused by intensive burrowing. This lithology is rich in large orbitolinids, gastropods and bivalves, whereas oysters, miliolinids and echinid remains are of subordinate importance. The limestone-dominated interval ends abruptly and is overlain by a succession of varicoloured peritidal mud- and siltstones with sandy and marly limestone intercalations (97.5–113 m). In this unit, the top of individual limestone beds is frequently marked by thick-shelled oyster colonies, often preserved in growth position. The greenish to dark-grey mudstones are characterized by millimetre-scale lamination, whereas the marly and sandy lithologies display intense bioturbation. This variegated interval passes again into thick-bedded limestones with thin clayey interbeds (113–131 m). The fossil content includes abundant oysters and other bivalves, echinoderm debris, gastropods, miliolinids and orbitolinids. The uppermost limestone bed (128 m) is characterized by abundant rudists, occurring reworked as well as in growth position, and serves as a distinct marker bed, namely as the lowermost bed of the Ponta da Galé Member.
The investigated succession has been studied in detail from a bio- and sequence-stratigraphic perspective by Rey, Bilotte & Peybernes (Reference Rey, Bilotte and Peybernes1977), Berthou & Schroeder (1979), Berthou & Leereveld (1990), Rey (Reference Rey1992, Reference Rey2006) and Dinis, Rey & de Graciansky (Reference Dinis, Rey and de Graciansky2002). According to a revised biostratigraphic framework based on dinoflagellate cyst assemblages (Heimhofer et al. Reference Heimhofer, Hochuli, Burla and Weissert2007), the upper part of the Rodízio Formation is characterized by an age not older than Early Albian, not excluding a Late Aptian age for its lower part. The overlying Água Doce Member is considered to be of Early and Middle Albian age. Biostratigraphic evidence derived from dinoflagellate cysts does not provide evidence for Upper Albian strata in the studied part of the succession.
Based on the sequence-stratigraphic interpretation of Dinis, Rey & de Graciansky (Reference Dinis, Rey and de Graciansky2002) and Rey, de Graciansky & Jacquin (Reference Rey, de Graciansky and Jacquin2003), the studied section covers at least two second-order transgressive–regressive cycles (Fig. 2). A first progradational–retrogradational sequence is covered by the lower part of continental siliciclastic sediments of the Rodízio Formation. A second sequence is characterized by an extensive transgressive (~ 15–97.5 m) and a short regressive part and covers the upper Rodízio Formation and most of the Água Doce Member. The uppermost part of the studied succession corresponds to the transgressive part of a third second-order cycle, including the top of the Água Doce and the lowermost levels of the Ponta da Galé Member.
3. Palaeogeographic and palaeoclimatic framework
During late Early Cretaceous times, the Iberian Peninsula was situated at a palaeolatitude between 25° and 35 °N (Sewall et al. Reference Sewall, van de Wal, van der Zwan, van Oosterhout, Dijkstra and Scotese2007) and during most of this period formed part of an archipelago, which resulted from the expansion of epicontinental shallow seas during times of high sea-level (Chumakov et al. Reference Chumakov, Zharkov, Herman, Doludenko, Kalandadze, Lebedev, Ponomarenko and Rautian1995; Hay et al. Reference Hay, DeConto, Wold, Wilson, Voigt, Schulz, Rossby Wold, Dullo, Ronov, Balukhovsky, Söding, Barrera and Johnson1999). The Lusitanian Basin formed part of the eastern passive margin of the evolving North Atlantic and was strongly influenced by tectonic movements resulting in several regional unconformities and transgressive–regressive cycles (Dinis et al. Reference Dinis, Rey, Cunha, Callapez and Pena dos Reis2008).
Generally, the late Early Cretaceous is considered a time characterized by exceptional global warmth as expressed in low equator–pole thermal gradients, high atmospheric CO2 levels and the absence of large continental ice sheets (e.g. Huber, Hodell & Hamilton, Reference Huber, Hodell and Hamilton1995; Price, Reference Price1999; Haworth et al. Reference Haworth, Hesselbo, McElwain, Robinson and Brunt2005; Forster et al. Reference Forster, Schouten, Baas and Sinninghe Damsté2007; Herman & Spicer, Reference Herman and Spicer2010). Following a phase of pronounced aridity during the Barremian to Aptian interval (Ruffell & Batten, Reference Ruffell and Batten1990), also demonstrated to occur on the western Iberian margin (Berthou, Blanc & Chamley, Reference Berthou, Blanc and Chamley1982), the southward migration of the equatorial humid belt during Late Aptian to Albian times reflects a significant change in the overall palaeoclimatic patterns (Chumakov et al. Reference Chumakov, Zharkov, Herman, Doludenko, Kalandadze, Lebedev, Ponomarenko and Rautian1995). According to the palaeoclimatic reconstructions of the latter authors, the southern part of Iberia was located within a broad zone of arid to semi-arid conditions (Northern Hot Arid belt, NHA), even with developed erg systems (Rodríguez-López et al. Reference Rodríguez-López, Meléndez, de Boer and Soria2008, Reference Rodríguez-López, Meléndez, de Boer and Soria2010), whereas the northern part was within the Northern Mid-latitude Warm humid belt (NMW) during Albian times.
Based on findings of plant macro-remains and on palynological assemblages from the Iberian Peninsula, different types of palaeovegetation and associated environments have been distinguished e.g. (Diéguez, Peyrot & Barrón, Reference Diéguez, Peyrot and Barrón2010). These include forest communities dominated by Taxodiaceae–Cupressaceae thriving in riparian or mangrove habitats as well as conifer forests composed of Cheirolepidaceae and tree ferns reflecting more stressed conditions (Peyrot et al. Reference Peyrot, Rodriguez-Lopez, Lassaletta, Meléndez and Barrón2007). On the other hand, freshwater-related environments like back swamps or riverbanks were populated by hygrophilous plant communities including Bryophyta, Lycophyta and various types of ferns as well as plants assigned to the Cycadales/Ginkoales/Bennettitales complex and early angiosperms (Coiffard, Gomez & Thevenard, Reference Coiffard, Gomez and Thevenard2007; Villanueva-Amadoz et al. Reference Villanueva-Amadoz, Pons, Diez, Ferrer and Sender2010). In summary, the vegetation was characteristic of a winter-wet biome under a semi-arid subtropical climate (Diéguez, Peyrot & Barrón, Reference Diéguez, Peyrot and Barrón2010).
4. Materials and methods
4.a. Strontium isotope stratigraphy
For the analysis of the strontium isotope composition, a total of 25 visually well-preserved oysters were collected from the Água Doce Member of the Galé Formation. Oysters are relatively abundant in the detritus-rich shoal-water deposits and inner shelf limestones of the Guincho section, often occurring in the form of regular pavements covering entire bedding planes. Oysters collected from the succession belong to the genus Exogyra and Ostrea (Rey & Cugny, Reference Rey and Cugny1978) and occur throughout the entire Galé Formation providing an essentially continuous coverage of the studied strata. Only the lowermost part of the Água Doce Member and the siliciclastic sediments of the Rodízio Formation were devoid of suitable shell material.
Oysters precipitate a shell consisting of low-Mg calcite and composed of individual foliate and prismatic layers (Carter, Reference Carter and Carter1990). The specific mineralogy makes their calcitic shells relatively robust against subsequent diagenetic alteration processes which may affect the primary chemical composition of the shell material. Therefore, oyster shell calcite is considered a reliable carrier of the seawater Sr-isotope signal as demonstrated by previous studies (Jones et al. Reference Jones, Jenkyns, Coe and Hesselbo1994; Veizer et al. Reference Veizer, Ala, Azmy, Bruckschen, Buhl, Bruhn, Carden, Diener, Ebneth, Godderis, Jasper, Korte, Pawellek, Podlaha and Strauss1999; Denison et al. Reference Denison, Miller, Scott and Reaser2003; Burla et al. Reference Burla, Oberli, Heimhofer, Wiechert and Weissert2009; Schneider, Fürsich & Werner, Reference Schneider, Fürsich and Werner2009). However, conventional sampling of thin-shelled oysters can result in contamination by adjacent substrate and/or altered shell material or in insufficient sampling amounts. To circumvent this problem, a system combining laser ablation (LA) with multi-collector inductively coupled plasma mass spectrometry (MC-ICP-MS) was used for this study, which yields precise and accurate Sr-isotope data at a high spatial resolution (e.g. Christensen et al. Reference Christensen, Halliday, Lee and Hall1995; Woodhead et al. Reference Woodhead, Swearer, Hergt and Maas2005; Burla et al. Reference Burla, Oberli, Heimhofer, Wiechert and Weissert2009).
Following visual inspection, the selected oyster shells were carefully cleaned to remove matrix material. Cleaned shells were cut using a diamond saw, placed on a specimen slide and polished. Following conventional petrographic inspection, all shell samples were investigated using a cold cathode luminescence (CL) optical system (Technosyn CL8200 MK II) at ETH Zurich, Switzerland. Only non-luminescent shell portions of the inner, foliate shell layer where used for subsequent laser ablation sampling.
Sr-isotope compositions were measured on a Nu Plasma 1700 MC-ICP-MS coupled to an Excimer 193 nm ArF laser (see Burla et al. Reference Burla, Oberli, Heimhofer, Wiechert and Weissert2009 for details). Guided by prior CL mapping, typically 15 spots were located on each sample and ablated for 50 seconds each (120 μm spot size). The measurements were internally corrected for mass bias and interference by Rb, Kr and Ca argides, using equations given in Burla et al. (2009 and online supporting information). Groups of one to five sample measurements were bracketed by measurements on recent oyster shell material, the latter yielding a mean 87Sr/86Sr ratio of 0.709161 ± 0.000016 (external reproducibility at 1 standard deviation, n = 16). With the use of these bracketing standards, a renormalization to a value of 0.709175 was carried out on the samples in order to minimize ratio drift and to render the data compatible for comparison with the LOWESS model for seawater Sr evolution (Howarth & McArthur, Reference Howarth and McArthur1997; McArthur, Howarth & Bailey, Reference McArthur, Howarth and Bailey2001). Precision for runs on pristine samples ranges from ±0.000008 to ±0.000016 (1σ; Table 1) and includes an error component from the renormalization procedure. Average external reproducibility calculated from repeated measurements on nine different samples from a companion study (Burla et al. Reference Burla, Oberli, Heimhofer, Wiechert and Weissert2009) was ±0.000009 (1σ).
Table 1. Strontium isotopic results for the Água Doce Member of the Galé Formation

After internal correction for instrumental mass fractionation using 88Sr/86Sr, the data have been normalized to a value of 0.709175 based on standard-sample bracketing, using modern oyster shells as standard (for details see Burla et al. Reference Burla, Oberli, Heimhofer, Wiechert and Weissert2009 and online supporting information). The LOWESS ages (Howarth & McArthur, Reference Howarth and McArthur1997; McArthur, Howarth & Bailey, Reference McArthur, Howarth and Bailey2001) and their bracketing values are derived from Look-Up Table Version 4: 08/04, the latter by combining 2σ measurement uncertainties with error envelopes of the LOWESS curve. NA – no match of 87Sr/86Sr with the LOWESS curve.
4.b. Palynology
A total of 36 rock samples from the Guincho section were prepared for palynological analysis. Despite the selection of apparently well-suited samples (including fresh medium- to dark-grey silt- and claystones), 16 samples were barren of palynomorphs. Cleaned, crushed and weighed samples (20 to 80 g) were treated with HCl and HF following standard palynological preparation techniques (e.g. Traverse, Reference Traverse2007). All 20 productive samples were studied for their sporomorph content (spores and pollen). A minimum of 200 (average of 240) sporomorphs were determined and counted per slide. Light photomicrographs were taken using an Olympus BX 51 light microscope (LM) equipped with an Olympus DP 12 digital camera. The preservation of the studied palynomorphs is fairly good to excellent. Individual grains exhibit no obvious signs of post-depositional degradation. Thermally unaltered preservation of organic matter is indicated by the virtually unchanged colouring of the palynomorphs (TAI < 2 according to the thermal alteration index of Staplin, Reference Staplin and Staplin1982).
4.c. Clay mineralogy
A total of 25 samples from the Guincho section were analysed for their clay mineralogy. Clay mineral analyses were based on methods described by Adatte, Stinnesbeck & Keller (Reference Adatte, Stinnesbeck, Keller, Ryder, Fastovsky and Gartner1996). Ground chips were mixed with deionized water (pH 7–8) and agitated. The carbonate fraction was removed by addition of 10% HCl at room temperature for 20 minutes or longer until all the carbonate was dissolved. Ultrasonic disaggregation was accomplished during 3 minute intervals. The insoluble residue was washed and centrifuged (5–6 times) until a neutral suspension was obtained (pH 7–8). Separation of two different grain-size fractions (< 2 μm and 2–16 μm) was obtained by the timed settling method based on Stokes law. The selected fraction was then pipetted onto a glass plate and air-dried at room temperature. X-ray diffraction (XRD) analysis of oriented clay samples were carried out after air drying at room temperature and under ethylene-glycol solvated conditions. The intensities of selected XRD peaks characterizing each clay mineral present in the size fraction (e.g. chlorite, mica, kaolinite and illite/smectite mixed-layers) were measured for a semi-quantitative estimate of the proportion of clay minerals present in two size fractions (< 2 μm and 2–16 μm). Determination of the illite/smectite (I/S) mixed-layers and their content in smectitic expandable layers is based on the method of Moore & Reynolds (Reference Moore and Reynolds1997).
5. Results
5.a. Sr-isotope ratios of oyster shell calcite
The Sr-isotope data are graphically displayed in Figure 2 and summarized in Table 1. Measured Sr-isotope ratios of oyster calcite from the Água Doce Member of the Galé Formation at Guincho vary between 0.707373 ± 0.000023 and 0.707456 ± 0.000025 (2σ). The Sr-isotope record shows a distinct trend towards higher Sr ratios as a function of stratigraphic height. The lower part (22 m to 71 m) is characterized by 87Sr/86Sr ratios fluctuating between 0.707379 ± 0.000032 and 0.707400 ± 0.000021 with the exception of two outliers in its upper part (samples L-41/1 and L-43/1). On average the middle part of the Água Doce Member (72–90 m) shows slightly higher ratios ranging between 0.707386 ± 0.000025 and 0.707413 ± 0.000033. The strata of the upper Água Doce Member (91 m to 128 m) display the highest ratios with values between 0.707424 ± 0.000021 and 0.707456 ± 0.000025 with the highest Sr-isotope ratio occurring in the uppermost sample measured (L-95, 121.2 m).
Up to now, a number of similar late Early Cretaceous 87Sr/86Sr curves, both in terms of the trend and absolute values, have been published (Bralower et al. Reference Bralower, Arthur, Leckie, Sliter, Allard and Schlanger1994; Jones et al. Reference Jones, Jenkyns, Coe and Hesselbo1994; McArthur, Howarth & Bailey, Reference McArthur, Howarth and Bailey2001). These curves show a distinct minimum in the Late Aptian followed by a subsequent rise towards higher ratios during Albian times. Following a steep increase during latest Aptian and Early Albian times, the curve starts to flatten out during the Middle Albian with the Late Albian being characterized by comparatively stable values reflecting a plateau phase of the seawater strontium trend. Our results obtained on oyster calcite appear to conform to such a pattern both in terms of trend and absolute values.
Converted to numerical ages according to the LOWESS best-fit curve (SIS Look-Up Table Version 4: 08/04; Howarth & McArthur, Reference Howarth and McArthur1997; McArthur, Howarth & Bailey, Reference McArthur, Howarth and Bailey2001), 87Sr/86Sr ratios range from 109.4 +1.4/−4.7 Ma (L-11) to 108.3 +1.4/−4.7 Ma (L-23), disregarding samples L41–1 and L43–1. This would indicate an Early to Middle Albian age based on the timescale of Gradstein, Ogg & Smith (Reference Gradstein, Ogg and Smith2004) for the lower part of the Água Doce Member. The middle part of this member is assigned to the Middle Albian (109.1 +1.2/−4.2 Ma (L-32) to 107.0 +2.7/−13.2 Ma (L-62)), whereas its uppermost interval would be Late Albian (104.2 to 103.4 Ma with an error envelope ranging from c. 108.8 to 94.8 Ma) in age (Fig. 3). However, owing to the flat gradient of the seawater Sr-isotope curve from the Middle Albian onwards, the error derived from the combined uncertainties of the measurements and of the SIS look-up table is considerable and results in significant uncertainties regarding the chronostratigraphic position of this part of the section (Table 1).

Figure 3. Comparison of LOWESS best-fit curve (black line) and 95% confidence intervals (thin grey lines) versus Sr-isotope ratios derived from the Guincho section. To reduce the effect of changes in sedimentation rate, the Guincho Sr-isotope record has been subdivided into three stratigraphic intervals (following the revised age assignment to Early, Middle and Late Albian in Fig. 2). The corresponding sets of data points derived from those three stratigraphic intervals have been plotted against numerical age.
5.b. Spore-pollen assemblage
The sediments of the Guincho section contain a rich and diverse terrestrial palynoflora composed of various gymnosperm pollen, pteridophyte spores and angiosperm pollen taxa. Numerous spores and gymnosperm pollen were determined on the generic level; other forms were treated as groups (e.g. bisaccate pollen). In the studied record we differentiated 14 genera of gymnosperm pollen (including 7 species) and 20 genera of spores (including 14 species). Angiosperm pollen show a high diversity comprising 50 different forms, which have been previously described in detail by Heimhofer et al. (Reference Heimhofer, Hochuli, Burla and Weissert2007). To obtain information on changes in humidity, a xerophyte/hygrophyte ratio has been calculated following the concept of earlier studies (Visscher & van der Zwan, Reference Visscher and van der Zwan1981; Hochuli et al. Reference Hochuli, Os Vigran, Hermann and Bucher2010). In this study, all spores are regarded as hygrophytic plants whereas the xerophyte group is represented solely by Classopollis spp.
Based on quantitative distribution of the major pollen groups, four different local pollen zones (LPZ) are distinguished (Fig. 4) from the base to the top of the studied succession. The lowermost LPZ I (0–38.0 m) is characterized by an increase in Classopollis spp. increasing from less than 5% to up to 44%, high abundances of Inaperturopollenites spp. (up to 34%) and a decline in Perinopollenites spp. (from 28% to less than 10%) (Fig. 5k, m–q, s, t, x). Other gymnosperm pollen (including bisaccate pollen, Araucariacites spp., Exesipollenites spp.; Fig. 5a, b, d, j, v, w, y) occur in low numbers, whereas trilete spore abundances vary between 4% and 17%. In this interval, the majority of the spores belong to the spore taxa Cicatricosisporites spp., Deltoidospora spp. and Gleicheniidites spp. (Fig. 6a–c, e, f, r, w) and show a mean diversity of seven genera. Pollen assigned to Afropollis cf. jardinus are a rare but regular component of LPZ I where they account for up to 2% (Fig. 5aa, bb). Similarly, Sciadopityspollenites spp., Eucommiidites spp. and Callialasporites spp. occur as subordinate floral elements (Fig. 5c, e, f, h, i, r, z). In LPZ I, angiosperm pollen are dominated by monocolpate forms and account for up to 8% (average 4.1%). Tricolpate forms are restricted to rare occurrences of Tricolpites spp., Phimopollenites spp. and Retitricolpites spp.

Figure 4. Simplified lithological log, lithostratigraphy, relative abundance [%] of spores and pollen and clay mineral distribution through the Guincho section, Lusitanian Basin, Portugal. LPZ – local pollen zone.

Figure 5. Photomicrographs of selected gymnosperm pollen grains from the Guincho section. Scale bar is 10 μm if not indicated differently. (a) Araucariacites sp. (granulate form); L-72-5; 9.0/134.1; (b) Araucariacites cf. australis Cookson, Reference Cookson1947; L-72-5; 6.7/143.8; (c) Callialasporites sp.; L-91.5; 9.2/134.2; (d) Araucariacites australis Cookson, Reference Cookson1947; L-66-4; 12.1/145.5; (e) Callialasporites dampieri (Balme) Sukh-Dev, Reference Sukh-Dev1961; L-16-3; 8.4/141.0; (f) Callialasporites trilobatus (Balme) Sukh Dev, 1961; L-72-5; 11.3/132.5; (g) Ephedripites cf. barghoornii/staplinii formgroup (Pocock) sensu Herngreen, Reference Herngreen1973; L-16-4; 5.0/133.1; (h, i) Callialasporites aff. dampieri (Balme) Sukh-Dev, Reference Sukh-Dev1961; L-16-4; 9.2/121.7; (j) Araucariacites sp. (finely echinate form); L-55-5; 12.2/127.2; (k) Perinopollenites sp.; L-88.4; 8.0/135.0; (l) Steevesipollenites sp.; K-3.1.4; 19.7/142.0; (m) Classopollis sp. tetrade; L-55-5; 19.2/138.8; (n, o) Classopollis sp.; L-66-4; 7.0/134.3; (p) Classopollis sp.; L-55-5; 12.2/134.4; (q) Classopollis sp. (finely echinate form); L-55-5; 7.8/141.7; (r) Sciadopityspollenites sp.; L-16-4; 9.0/135.7; (s, t) Classopollis sp. (smooth form); L-66-4; 9.2/144.8; (u) Podocarpidites sp.; L-60-5; 10.3/127.4; (v) Exesipollenites sp.; L-66-4; 18.7/130.1; (w) Exesipollenites tumulus Balme, Reference Balme1957; L-66-4; 7.2/144.2; (x) Inaperturopollenites sp.; L-66-4; 7.0/137.0; (y) Pinuspollenites sp.; L-66-4; 18.7/140.0; (z) Eucommiidites sp.; L-66-4; 13.2/130.3; (aa, bb) Afropollis cf. jardinus; (Brenner) Doyle, Jardiné & Doerenkamp, Reference Doyle, Jardiné and Doerenkamp1982; L-16-4; 8.0/136.3.

Figure 6. Photomicrographs of selected spores from the Guincho section. Scale bar is 20 μm on all photomicrographs. (a, b) Cicatricosisporites cf. hallei Delcourt & Sprumont, Reference Delcourt and Sprumont1955; L-55-5; 16.1/134.7; (c) Cicatricosisporites cf. venustus Deák, Reference Deák1963; L-66-4; 14.8/146.2; (d) Plicatella cf. cristata (Markova) Davies, Reference Davies1985; L-52.6; 10.4/129.0; (e) Cicatricosisporites cf. patapscoensis Brenner, Reference Brenner1963; L-16-4; 12.3/122.2; (f) Cicatricosisporites sp.; L-16-3; 16.7/124.8; (g, h) Plicatella cf. concentrica (Kemp) Davies, Reference Davies1985; L-16-3; 6.4/140.3; (i) Costatoperforosporites triangulatus Deák, Reference Deák1962; L-55.5; 13.5/128.0; (j, k) Appendicisporites potomacensis Brenner, Reference Brenner1963; L-16-3; 12.2/123.7; (l) Crybelosporites cf. pannuceus (Brenner) Srivastava, Reference Srivastava1977; L-16-3; 18.4/131.2; (m) Concavisporites sp.; L-16-4; 8.2/124.9; (n) Hamulatisporites sp.; L-55-5; 18.9/132.6; (o) Impardecispora sp.; L-16-4; 8.3/126.3; (p) Trilobosporites apibaculatus Brenner, Reference Brenner1963; L-16-3; 9.6/131.8; (q, v) Matonisporites excavatus Brenner, Reference Brenner1963; L-55-5; 7.9/141.5; (r) Gleicheniidites cf. senonicus Ross, Reference Ross1949; L-60-5; 17.8/136.3; (s, t) Taurocusporites cf. segmentatus Stover, Reference Stover1962; L-66-4; 16.2/146.3; (u) Cingutriletes cf. congruens Pierce, Reference Pierce1961; L-88.4; 8.8/131.8; (w) Gleicheniidites cf. circinidites (Cookson) Brenner, Reference Brenner1963; L-55-5; 14.8/137.0; (x, y) Klukisporites sp.; L-55-5; 9.0/133.0; (z) Leptolepidites sp.; L-55-5; 12.2/129.2.
LPZ II includes the interval between 38.0 m and 76.6 m and is characterized by a prominent peak in trilete spores reaching up to 54%. This increase in spore abundance is most pronounced in the Cicatricosisporites group, but can also be observed in spores assigned to Plicatella spp., Leptolepidites spp., Concavisporites spp., Cingutriletes spp. and Echinatisporites spp. (Fig. 6d, g, h, m, z). Peak abundance of trilete spores is paralleled by the highest spore diversity with 13 different genera. The increase in spore abundance is accompanied by a significant decline in Classopollis spp., Exesipollenites spp., Inaperturopollenites spp. and a further decrease in Perinopollenites spp., whereas Araucariacites spp. displays a slight increase, reaching up to 8% of the sporomorph count. Exesipollenites spp. shows an increase at the beginning of LPZ II to values of up to 11%, but decreases to less than 2% during peak spore abundance. Compared to LPZ I, bisaccate pollen show no significant variations in abundance and fluctuate between 2% and 11%. Noteworthy is the occurrence of Podocarpidites spp. (Fig. 5u) and Vitreisporites pallidus within LPZ II. Angiosperm pollen represent a relatively common element within this interval (up to 8%; average 4.9%), and the first occurrence of several new tricolpate taxa is observed (including Artiopollis spp., Hammenia spp. and Striatopollis spp.)
The palynoflora of LPZ III (76.6–95.0 m) is characterized by low abundances of Inaperturopollenites spp., Araucariacites spp. and trilete spores, with the latter group showing a reduced mean diversity (average of 5.8 genera). In contrast, Classopollis spp. and Exesipollenites spp. show comparatively high abundances, the count of the latter reaching up to 42%. Together with Sciadopityspollenites spp. and Eucommiidites spp., pollen assigned to Perinopollenites spp. are of subordinate importance and account for less than 2% of the assemblage. Ephedripites spp. (Fig. 5g) and Callialasporites spp are virtually absent. Compared to LPZ II the relative contribution of angiosperm pollen is slightly reduced within LPZ III and accounts for less than 5% (average 3.4%).
Following a palynologically barren interval between 95.0 m and 115.2 m, the assemblage characterizing the uppermost LPZ IV (115.2–127.8 m) shows an increase in Classopollis spp, which is paralleled by a decrease in Inaperturopollenites spp. In contrast to the previous LPZ, Exesipollenites spp. occurs in low abundances of less than 8%, whereas trilete spores show a return to values of up to 12% (with Cicatricosisporites spp. accounting for 5%). Perinopollenites spp. is virtually absent in this zone. Angiosperm pollen shows the highest relative abundance of the entire record reaching up to 12% (average 7.9%).
5.c. Clay mineral assemblage
The clay mineral assemblage of the Guincho section (fraction < 2 μm) is dominated to varying degrees by mica and kaolinite whereas chlorite and I/S mixed-layer clay minerals represent only a subordinate fraction (Fig. 4). The relative proportion of the different clay minerals shows distinct variations within the stratigraphic succession. Mica accounts for 30.3% on average and shows a continuous increase throughout the succession ranging from less than 2.4% in the coarse-grained siliciclastic sediments of the Rodízio Formation to reach > 40% at the base of the Ponta da Galé Member. The increasing trend in mica abundance is punctuated by two peaks of 49.5% (at 47.7 m) and 54.0% (at 95.0 m), respectively. Compared to mica, kaolinite shows an opposite pattern with high kaolinite abundances (> 70%) prevailing within the Rodízio Formation and lower part of the Água Doce Member and reduced abundances (~ 40%) above, in most of the Água Doce Member (mean of 56.2%). A distinct minimum of 18.0% is paralleled by peak mica abundances at 95.0 m. Chlorite shows a relatively stable abundance pattern, fluctuating between 2.6% and 14.0% (mean of 6.3%) in most samples (exception of 0% in the lowermost sample). In contrast, the I/S mixed-layers show a slow but distinct increase in abundance from 0.0% at the very base to 24.5% in the uppermost interval (mean of 7.6%). Following the criteria of Moore & Reynolds (Reference Moore and Reynolds1997), identified I/S are of R1–R3 type with 15–20% smectitic expandable layers.
The obtained results agree well with other studies on the clay assemblages of the Upper Aptian and Albian deposits of the region, showing a change from kaolinite to a predominance of illite approximately in Middle Albian times (Berthou, Blanc & Chamley, Reference Berthou, Blanc and Chamley1982). Around 100 to 150 km further north in the basin, kaolinite is much more important all over the Figueira da Foz Formation (Upper Aptian to lower Cenomanian) (e.g. Grade & Moura, Reference Grade and Moura1992), with an increase in smectite and I/S mixed-layers in the presumably Upper Albian to lower Cenomanian levels (Rocha & Gomes, Reference Rocha and Gomes1995).
6. Discussion
6.a. Stratigraphic assignment
Generally the 87Sr/86Sr ratios derived from low-Mg oyster calcite of the studied Guincho section match the global marine Sr-isotope trend for the Albian Stage, both in terms of the general rise in the ratio and the absolute values (McArthur, Howarth & Bailey, Reference McArthur, Howarth and Bailey2001). Correlation with existing Sr-isotope curves from open marine environments (Bralower et al. Reference Bralower, Fullagar, Paull, Dwyer and Leckie1997) allows a tentative distinction between Lower, Middle and Upper Albian strata. Comparison with oyster-derived Sr-isotope results from the Portuguese Algarve region generated by the same analytical technique (Burla et al. Reference Burla, Oberli, Heimhofer, Wiechert and Weissert2009) shows a good match between the two records for the Early to Middle Albian interval, enabling correlation between the two successions. On the other hand, certain discrepancies are observed between the Guincho results and an oyster-derived Sr-isotope record from the Albian of northern Texas, USA (Denison et al. Reference Denison, Miller, Scott and Reaser2003). Both records show a distinct increase in 87Sr/86Sr ratios for the Early to Middle Albian interval, but both amplitudes and absolute values differ significantly. The steeper gradient and the significantly higher 87Sr/86Sr ratios (reaching up to 0.70750) of the Texan oyster record may reflect freshwater influx, which could have shifted the original seawater Sr-isotope composition to more radiogenic ratios (Ingram & Sloan, Reference Ingram and Sloan1992; Korte et al. Reference Korte, Kozur, Bruckschen and Veizer2003). A similar mechanism may be invoked to explain the small-scale shifts observed in the Guincho Sr-isotope record, in particular in its lower part. Here, the observed gradual low-amplitude fluctuations may reflect variations in the riverine input of radiogenic Sr derived from continental silicate weathering.
Generally the age assignment based on the isotope record of the Guincho section is in good agreement with existing age interpretations, thus confirming the accuracy of previous stratigraphic studies based on macrofauna and orbitolinids (Rey, Bilotte & Peybernes, Reference Rey, Bilotte and Peybernes1977; Berthou & Schroeder, Reference Berthou and Schroeder1979), dinoflagellate cysts (Berthou & Leereveld, Reference Berthou and Leereveld1990; Heimhofer et al. Reference Heimhofer, Hochuli, Burla and Weissert2007) and sequence-stratigraphic considerations (Rey, Reference Rey1992; Dinis, Rey & de Graciansky, Reference Dinis, Rey and de Graciansky2002; Rey, de Graciansky & Jacquin, Reference Rey, de Graciansky and Jacquin2003). A late Early Albian age for the lower part of the Água Doce Member is corroborated by the consistent occurrence of Dinopterygium cladoides.
A distinct offset in the Sr-isotope record in the middle part of the Água Doce Member at ~ 95 m (between samples L-66 and L-69/1) may reflect a sedimentary hiatus. Evidence is provided by the occurrence of a distinct hardground (discontinuités sedimentaires in Rey, Reference Rey1992), which is considered to reflect the maximum flooding surface of a second-order cycle (Dinis, Rey & de Graciansky, Reference Dinis, Rey and de Graciansky2002; Rey, de Graciansky & Jacquin, Reference Rey, de Graciansky and Jacquin2003).
Stratigraphic discrepancies exist with regard to the position of the upper part of the Água Doce Member and the lowermost part of the Ponta da Galé Member (~ 100–131 m; Fig. 2). According to the numerical age assignment derived from the Sr-isotope data, this interval belongs to the early Late Albian (c. 104.2 to 103.4 Ma nominal; however, note the error envelope allows for a range of c. 108.8 to 94.8 Ma). Similarly, Rey, Bilotte & Peybernes (Reference Rey, Bilotte and Peybernes1977) considered the interval above the bed including the ammonite Knemiceras uhligi (~ 95 m) to be Late Albian in age, and Berthou & Schroeder (1979) indicate an Early to Middle Albian age for the carbonates below ~ 85 m based on the occurrence of the orbitolinid Simplorbitolina manasi-conulus. In contrast, a Middle Albian age has been proposed for the interval above ~ 92 m based on the absence of a characteristic Late Albian dinoflagellate cyst assemblage (Heimhofer et al. Reference Heimhofer, Hochuli, Burla and Weissert2007). However, given the near-shore character of the deposits in this part of the succession, the lack of typical Late Albian dinoflagellate taxa may result from the proximity and restricted character of the depositional setting.
In summary, considering the biostratigraphic constraints and the 87Sr/86Sr chronostratigraphic best-fit, the boundary between the Middle and Late Albian is tentatively located in the studied section between 89.9 m (sample L-66) and 95.0 m (sample L-69). The Sr-isotope results of the Guincho section further support the suitability of oyster-derived low-Mg calcite for stratigraphic applications and corroborate earlier biostratigraphic studies.
6.b. Significance of near-shore marine palynomorph records
When dealing with sporomorph assemblages obtained from near-shore marine sedimentary deposits, potential effects of hydrodynamic sorting during transportation and deposition have to be considered. Differential sorting due to varying buoyancy or sporomorph size and density can results in significant bias of the studied association (Tyson, Reference Tyson1995; Traverse, Reference Traverse2007). This applies in particular to bisaccate conifer pollen grains, which can remain afloat for weeks explaining the observed relative off-shore increase in bisaccate pollen in modern continental margin sediments (Heusser & Balsam, Reference Heusser and Balsam1977). In the studied succession the relative abundance of bisaccate pollen varies between 2% and 12%, but does not show significant fluctuations with stratigraphic height. In fact, during Albian times sedimentation in the Lusitanian Basin was affected by distinct sea-level variations (Dinis, Rey & de Graciansky, Reference Dinis, Rey and de Graciansky2002; Rey, de Graciansky & Jacquin, Reference Rey, de Graciansky and Jacquin2003) as indicated by changes from peritidal (estuary to upper delta) to inner and shallow platform depositional settings (Rey & Cugny, Reference Rey and Cugny1978). However, the relatively stable distribution of bisaccates does not show a clear sporomorph sorting due to varying distance from the shoreline. Bias of the quantitative composition of sporomorph assemblages due to differential sorting has also been observed between thin-walled, non-saccate pollen grains and dense, ornamented trilete spores, with the latter being preferentially deposited more proximally, often in the vicinity of a river mouth (Heusser & Balsam, Reference Heusser and Balsam1977; Tyson, Reference Tyson1995). Again, the Guincho record does not display a strong correspondence between trilete spore abundance and sea-level variations since the exceptionally high spore abundances in LPZ II occur during a long-lasting transgressive phase, even if the minimum in LPZ III coincides with the second-order highest sea-level. The deposits in LPZ II show no sign of enhanced riverine detrital input or sea-level lowering, which could explain the pronounced peak in spore percentages. Hence the effects of hydrodynamic sorting processes are considered to be of minor importance for most of the observed sporomorph distribution patterns. This assumption is in agreement with the view that sporomorph assemblages obtained from marginal marine settings reflect changes in vegetation patterns on the adjacent hinterland rather than sorting during transport and deposition (Tyson, Reference Tyson1995; Abbink, Reference Abbink1998; Traverse, Reference Traverse2007).
6.c. Evolution of vegetation patterns and climate
The composition of the sporomorph assemblages obtained from the Albian deposits of the Guincho section is in good accordance with existing sporomorph records from other parts of Iberia (Groot & Groot, Reference Groot and Groot1962; Medus & Berthou, Reference Medus and Berthou1980; Medus, Reference Medus1982; Taugourdeau-Lantz et al. Reference Taugourdeau-Lantz, Azéma, Hasenboehler, Masure and Moron1982; Solé da Porta et al. Reference Solé da Porta, Querol, Cabanes and Salas1994; Peyrot et al. Reference Peyrot, Rodriguez-Lopez, Lassaletta, Meléndez and Barrón2007; Diéguez, Peyrot & Barrón, Reference Diéguez, Peyrot and Barrón2010; Villanueva-Amadoz et al. Reference Villanueva-Amadoz, Pons, Diez, Ferrer and Sender2010). The composition fits well into the palaeophytogeographic scheme of Brenner (Reference Brenner and Beck1976) in which the occurrence of various types of conifer-derived gymnosperm pollen (e.g. Classopollis spp., Araucariacites spp., Inaperturopollenites spp. and of bisaccate pollen grains of pinacean affinity) and the occurrence of diverse pteridophyte spore associations (including numerous representatives of the Schizaeaceae and Gleicheniaceae) place the Guincho record into the Southern Laurasian floral province. However, minor influence of the Northern Gondwana province adjacent to the south is indicated by the continuous occurrence of typical Gondwanan floral elements including Afropollis spp. as well as by the regular occurrence of pollen grains of the Ephedripites group. A position at the southernmost margin of the Southern Laurasian floral province is in good agreement with the concept of a transitional zone between the two major floral provinces, which incorporates floral elements from both provinces to varying extent (Hochuli, Reference Hochuli1981).
Based on the presumed ecological preferences and habitat requirements of the parent plants of the observed sporomorph groups, the distribution patterns can be interpreted in terms of changes in palaeovegetation and corresponding environmental conditions. Abbink (Reference Abbink1998) and Abbink, Van Konijnenburg-Van Cittert & Visscher (Reference Abbink, Van Konijnenburg-Van Cittert and Visscher2004) provide a comprehensive compilation of presumed ecologies of the parent plants of Late Jurassic–earliest Cretaceous sporomorph groups, which serves as a basis for the interpretation of the Guincho record. A distinct shift from forest communities dominated by cupressacean and taxodiacean conifers to the dominance of Classopollis–(Cheirolepidaceae) is observed in LPZ I (late Early Albian). Conifers of the family Cheirolepidaceae are considered thermophilous and drought-resistant and have thrived in a wide range of habitats, ranging from well-drained upland to coastal lowlands to even saline habitats (Vakhrameyev, Reference Vakhrameyev1982; Watson, Reference Watson and Beck1988; Heimhofer et al. Reference Heimhofer, Adatte, Hochuli, Burla and Weissert2008; Mendes et al. Reference Mendes, Dinis, Gomez and Pais2010). In contrast, Taxodiacean conifers (as indicated by Perinopollenites) are interpreted to form part of lush vegetation thriving predominantly in wet lowland environments (Pelzer, Riegel & Wilde, Reference Pelzer, Riegel, Wilde, McCabe and Parrish1992). Hence, the marked increase in cheirolepidacean at the expense of taxodiacean conifers during LPZ I most probably reflects a shift towards more arid conditions in the hinterland of the Lusitanian Basin. This climatic trend is supported by the clay mineral assemblage, where a decline in kaolinite abundance can be observed. Formation of kaolinite is usually associated with intense chemical weathering under humid tropical to subtropical conditions (Gaucher, Reference Gaucher1981; Chamley, Reference Chamley1989). An inverse trend back towards a more humid climate characterizes the late Early Albian to early Middle Albian interval of LPZ II. Here, a pronounced peak in pteridophytes, particularly of ferns of the families Schizaeaceae and Matoniaceae as well as lycopsids, is accompanied by a marked decrease in cheirolepidacean conifers and points to the establishment of more humid conditions. High abundances of pteridophytes are characteristic of relatively moist and lush vegetation occurring, for example, along riversides and/or in costal lowlands (Van Konijnenburg-Van Cittert & Van der Burgh, Reference van Konijnenburg-van Cittert and van der Burgh1989; Pelzer, Riegel & Wilde, Reference Pelzer, Riegel, Wilde, McCabe and Parrish1992; Abbink, Reference Abbink1998). Peak abundances in pteridophytes are accompanied by Vitreisporites-producing pteridosperms, which are typically associated with warm and rather wet habitats (Pelzer, Reference Pelzer1984; Van Konijnenburg-Van Cittert & Van der Burgh, Reference van Konijnenburg-van Cittert and van der Burgh1989). A trend towards a more humid climate may be interpreted from a subtle increase in kaolinite content and decreasing mica abundances, reflecting a shift from physical to predominantly chemical weathering processes. Following this period of comparatively humid conditions, a reverse trend towards a more arid climate is indicated in LPZ III by a pronounced increase of Exesipollenites spp., most probably produced by bennettitalean or cupressacean plants. According to Watson & Sincock (Reference Watson and Sincock1992) and Hotton & Baghai-Riding (Reference Hotton, Baghai-Riding and Gee2010) the parent plants of Exesipollenites preferred a dry microclimate and were able to withstand a certain saline influence. This climatic interpretation is supported by sedimentological evidence. Reduced riverine siliciclastic input to the basin is indicated by the predominance of carbonate-rich deposits during LPZ III. Similarly, decreasing percentages of kaolinite point to a reduction in chemical weathering rates, most probably owing to limited water availability. A slightly modified picture emerges from the Late Albian interval of LPZ IV, where moderate abundances of pteridophytes occur in association with cupressacean, araucariacean and taxodiacean conifers and angiosperms. A rise in Classopollis pollen may indicate increasing dominance of drought-adapted cheirolepidaceans. Increased abundances of I/S mixed-layer minerals point to the erosion of poorly drained soils in the hinterland (Deconinck & Chamley, Reference Deconinck and Chamley1995). The climatic condition prevailing during LPZ IV are probably best described as semi-arid, reflecting conditions in between the enhanced aridity of LPZ III and the humid phase of LPZ II.
6.d. Palaeoenvironmental implications
Evaluation of the palynological data indicates that during Early to Middle Albian times the hinterland of the southern Lusitanian Basin was covered by evergreen vegetation, adapted to semi-humid conditions. Mixed conifer forests, essentially composed of Cheirolepidaceae, Taxodiaceae–Cupressaceae and Araucariaceae, sometimes accompanied by different types of Podocarpaceae and Pinaceae, prevailed. The forest understorey was composed of ferns of the groups Schizaeaceae, Gleicheniaceae and Cyatheaceae/Dicksoniaceae/Dipteridaceae and as well as of lycopsids and early angiosperms. Similar floral assemblages have been described from the Albian of Iberia, from the Basque-Cantabrian basin (Barrón, Comas-Rengifo & Elorza, Reference Barrón, Comas-Rengifo and Elorza2001) and from the Oliete Sub-basin (Peyrot et al. Reference Peyrot, Rodriguez-Lopez, Lassaletta, Meléndez and Barrón2007).
However, the stratigraphic succession of the palynofloras reflect some marked changes in the plant communities, including the demise of taxodiacean conifers (LPZ I), a pronounced increase in pteridophyte spores, particularly of Schizaeaceae (LPZ II) and a rise in Cupressaceae (LPZ III) as well as an overall increase in angiosperm pollen throughout the studied section. Whereas the increase in the abundance of angiosperm pollen is interpreted to reflect the incipient radiation of this group during Albian times (Medus & Berthou, Reference Medus and Berthou1980; Heimhofer et al. Reference Heimhofer, Hochuli, Burla, Dinis and Weissert2005, Reference Heimhofer, Hochuli, Burla and Weissert2007) observed variations in the distribution of gymnosperm pollen and pteridophyte spores are best explained as a response of vegetation to changes in regional climatic conditions, in particular to moisture availability. Changes in humidity during the studied time interval are well expressed in the xerophyte/hygrophyte ratio (Fig. 7). The dataset indicates significant variations in the temporal distribution of moisture availability, with an increase in regional humidity during late Early Albian times. Coeval deposits from the Algarve Basin, located ~ 200 km towards the south, show no evidence for increased humidity, neither in the palynoflora nor in the clay mineral composition (Heimhofer et al. Reference Heimhofer, Adatte, Hochuli, Burla and Weissert2008). Here, the palynological assemblages are strongly dominated by Classopollis pollen, resulting in a constantly high xerophyte/hygrophyte ratio throughout the Early Albian interval (Fig. 7).

Figure 7. Comparison of the xerophyte/hygrophyte ratios (dashed line) and angiosperm pollen relative abundances (solid line) between the Early Albian records of Guincho (Lusitanian Basin, W Portugal) and the Luz (Algarve Basin, S Portugal). Xerophyte/hygrophyte ratio of the Luz record is based on Heimhofer et al. (Reference Heimhofer, Adatte, Hochuli, Burla and Weissert2008), angiosperm pollen abundance is based on Heimhofer et al. (Reference Heimhofer, Hochuli, Burla and Weissert2007), and stratigraphic assignment follows Burla et al. (Reference Burla, Oberli, Heimhofer, Wiechert and Weissert2009).
Late Aptian to early Cenomanian deposits in the northern part of the western Iberian margin include very high proportions of kaolinite and are interpreted as a braided fluvial system (Dinis et al. Reference Dinis, Rey, Cunha, Callapez and Pena dos Reis2008), pointing to a climate that was, at least seasonally, wet. In contrast, sedimentological evidence for relatively arid conditions prevailing in eastern Iberia during Albian times is derived from the presence of a subtropical erg-margin system (Rodríguez-López et al. Reference Rodríguez-López, Meléndez, de Boer and Soria2008, Reference Rodríguez-López, Meléndez, de Boer and Soria2010). Sand sea development resulted from a northward shift of the northern subtropical high-pressure system, probably linked to the opening of the equatorial Atlantic gateway (Poulsen, Gendaszek & Jacob, Reference Poulsen, Gendaszek and Jacob2003). Arid palaeoclimatic conditions prevailing in southern and eastern Iberia during latest Early Cretaceous times are in line with the palaeogeographic reconstructions of Chumakov et al. (Reference Chumakov, Zharkov, Herman, Doludenko, Kalandadze, Lebedev, Ponomarenko and Rautian1995). According to these authors, the boundary between the so-called Northern Hot Arid belt and the Northern Mid-latitude Warm humid belt divides the Iberian Peninsula in an E–W direction. The position of the studied Guincho section close to the boundary of the two belts made this area sensitive to climatic changes resulting in expansion or contraction of the latitudinally arranged climatic zones. Hence, the occurrence of a more hygrophilous vegetation during late Early Albian times may reflect a transient expansion of the Northern Mid-latitude Warm humid belt towards the south, probably driven by the rearrangement of atmospheric or oceanic heat transport.
7. Conclusions
(1) The near-shore marine deposits of the Guincho section of the Lusitanian Basin, W Portugal were deposited in Early to early Late Albian times as indicated by Sr-isotope data derived from low-Mg oyster calcite. The new Sr-isotope record corroborates the existing biostratigraphic assignment of the lower Água Doce Member as being Early to Middle Albian in age. However, high 87Sr/86Sr values between 0.707424 ± 0.00002 and 0.707456 ± 0.00003 characterizing the uppermost Água Doce Member, point to an age not older than early Late Albian. The Sr-isotope data obtained using laser-ablation multi-collector ICP-MS highlight the suitability of low-Mg oyster shell calcite for the age assignment of Cretaceous near-shore marine deposits.
(2) The sedimentary deposits are characterized by a rich and diverse palynoflora essentially composed of conifer-derived gymnosperm pollen, trilete pteridophyte spores and angiosperm pollen. According to the spore-pollen data, the hinterland of the Lusitanian Basin was covered by an evergreen vegetation composed of mixed conifer forests (including Cheirolepidaceae, Taxodiaceae–Cupressaceae, Araucariaceae and subordinately Podocarpaceae, Pinaceae) with the understorey being composed of various ferns (Schizaeaceae, Gleicheniaceae, Cyatheaceae, Dicksoniaceae, Dipteridaceae), and a relatively diverse angiosperm assemblage. This vegetation is interpreted to correspond to semi-humid conditions.
(3) Across the stratigraphic succession, the spore-pollen record shows some distinct fluctuations in assemblage composition. Similarly, the clay mineral assemblages vary with stratigraphic height. Important changes in the plant community structure include the demise of taxodiacean conifers (LPZ I), a pronounced increase in pteridophyte spores (LPZ II) and a rise in bennettitalean or cupressacean-derived pollen (Exesipollenites spp.; LPZ III). In concert with the clay mineral data, these changes in the distribution patterns of gymnosperm pollen and pteridophyte spores are interpreted as reflecting changes in regional climate patterns, particularly in moisture availability. Distinct differences in the xerophyte/hygrophyte ratio between the Guincho record and data from the southern Algarve Basin are interpreted as reflecting the position of gross climatic belts, with southern and southeastern Iberia being under the influence of the northern subtropical high-pressure system during Early Albian times.
Acknowledgements
We thank P. W. Skelton (Open University Milton Keynes) for field assistance, U. Wiechert (FU Berlin) and the technical staff of the Institute of Isotope Geochemistry and Mineral Resources for support with the Sr-isotope measurements. The thorough comments of the editor and two anonymous reviewers are gratefully acknowledged. Financial support from ETH-Project TH-34/99–4 and by the Nordrhein-Westfälische Akademie der Wissenschaften und Künste to UH is gratefully acknowledged. JD's participation is supported by FCT (Fundação para a Ciência e a Tecnologia) through projects PTDC/CTE-GIX/104999/2008 and PTDC/CTE-GIX/113983/2009, with co-participation of the COMPETE Program/FEDER European Fund.