1. Introduction
The Junction Bed (sensu stricto), deposited in the Wessex Basin (southern UK) and exposed in cliffs along the Dorset coast (Fig. 1), is so called because, together with the underlying Marlstone Rock Bed, it spans the contact between the Middle and Upper Lias (Pliensbachian–Toarcian boundary). It is stratigraphically extremely condensed, containing obvious hardgrounds, and records most ammonite zones and subzones of the Toarcian: it is typically less than a metre in thickness in coastal outcrops. Lithologically, it comprises grey to yellow, pink to red and brown nodular micritic limestone, fossil-rich and heavily mineralized with dark-coloured iron and manganese oxyhydroxides, locally in the form of centimetre-scale nodules, coatings and dendrites (Buckman, Reference Buckman1922; Jackson, Reference Jackson1922, Reference Jackson1926; Arkell, Reference Arkell1933; Howarth, Reference Howarth1957, Reference Howarth1992; Hallam, Reference Hallam1967; Sellwood et al. Reference Sellwood, Durkin and Kennedy1970; Cope et al. Reference Cope, Getty, Howarth, Morton and Torrens1980; Jenkyns & Senior, Reference Jenkyns and Senior1991; Hesselbo & Jenkyns, Reference Hesselbo, Jenkyns and Taylor1995).

Fig. 1. Map of the Dorset coast, showing the location of Doghouse Cliff, below which blocks of Marlstone and Junction Bed in so-called normal facies are common on the foreshore. Further east, close to where the Eype’s Mouth Fault appears in the cliff, so-called fissure facies are present as neptunian sills of graded ammonite-rich micritic limestones that penetrate the Marlstone/Junction Bed complex (Jenkyns & Senior, Reference Jenkyns and Senior1991). The tops of some of the fissure fills contain sparry ferroan calcite (Raven & Dickson, Reference Raven and Dickson1989). Modified from Hesselbo & Jenkyns (Reference Hesselbo, Jenkyns and Taylor1995).
Ammonites, whose shells may be partially eroded or corroded and/or serpulid-encrusted and present as internal moulds, are common in the Junction Bed (sensu stricto), as are belemnites. Bivalves, brachiopods, gastropods and nautiloids are accompanying faunal elements (Hallam, Reference Hallam1967; Boomer et al. Reference Boomer, Lord, Page, Bown, Lowry and Riding2009; King, Reference King2011); smaller-scale biota includes foraminifera and ostracods. Calcareous nannofossils are found locally in age-equivalent strata but are not abundant in the outcrops on the Dorset coast (Jenkyns & Senior, Reference Jenkyns and Senior1991; cf. Boomer et al. Reference Boomer, Copestake, Page, Huxtable, Loy, Bown, Jones, O’Callaghan, Hawkes, Halfacree, Reay, Reolid, Duarte, Mattioli and Ruebsam2021). Cupola-shaped stromatolitic structures are present at some levels (Sellwood et al. Reference Sellwood, Durkin and Kennedy1970). In overall appearance, the Junction Bed (sensu stricto) closely resembles some of the highly condensed fine-grained pelagic coccolith-rich red limestones of the Tethyan Jurassic (e.g. Rosso Ammonitico) that also commonly contain stromatolites, possibly formed from microbial organisms growing within the photic zone, and mineralized Fe–Mn oxyhydroxide nodules and hardgrounds and which formed on fault-bounded topographic highs or seamounts, typically related to extensional half-graben systems (e.g. Jenkyns, Reference Jenkyns1970, Reference Jenkyns1971; Jenkyns & Torrens, Reference Jenkyns, Torrens and Végh-Neubrandt1971; Bernoulli & Jenkyns, Reference Bernoulli, Jenkyns, Dott and Shaver1974; Prescott, Reference Prescott1988; Cronan et al. Reference Cronan, Galácz, Mindszenty, Moorby and Polgari1991; Vera & Martín-Algarra, Reference Vera, Martín-Algarra, Bertrand-Sarfati and Monty1994; Massari & Westphal, Reference Massari, Westphal, Tewari and Seckbach2011; Reolid, Reference Reolid2011; Föllmi, Reference Föllmi2016; Scopelliti & Russo, Reference Scopelliti and Russo2021).
The underlying Marlstone Rock Bed, formerly thought to be entirely of spinatum zone age, but now known to include the tenuicostatum zone, thereby crossing the Pliensbachian–Toarcian boundary (Arkell, Reference Arkell1933; Howarth, Reference Howarth1980), is a coarse-grained pink to brown conglomeratic crinoidal Fe-oolitic limestone, also relatively condensed being nowhere more than 0.6 m thick, whose biota is similar to that of the overlying Junction Bed (sensu stricto), from which it is clearly separable as a distinct lithological unit: the contact between the two units is sharp and marked by an obvious hardground. In the new stratigraphic scheme promulgated by the British Geological Survey, the two units are together referred to as the Beacon Limestone Formation, with the Junction Bed itself (sensu stricto) termed the Eype Mouth Limestone (Cox et al. Reference Cox, Sumbler and Ivimey-Cook1999). In this account, the original nomenclature, sanctioned by long use, is retained and all subsequent references to the Junction Bed refer only to the fine-grained reddish limestone and do not include the underlying conglomeratic Marlstone, even though it is this unit that is now known to record the stage boundary (Fig. 2).

Fig. 2. Litho- and biostratigraphy of the Marlstone and Junction Bed (Junction Bed sensu lato), grouped together as the Beacon Limestone by the British Geological Survey (Cox et al. Reference Cox, Sumbler and Ivimey-Cook1999). Modified from Hesselbo & Jenkyns (Reference Hesselbo, Jenkyns and Taylor1995). Stratigraphy follows Jenkyns & Senior (Reference Jenkyns and Senior1991) and Howarth (Reference Howarth1992). The contact between the Middle and Upper Lias (Pliensbachian–Toarcian) was originally thought to lie between the Marlstone and the Junction Bed (hence its name) until discovery of tenuicostatum zone ammonites in the highest parts of the stratigraphically lower unit (cf. Arkell, Reference Arkell1933; Howarth, Reference Howarth1980). PL – Pliensbachian.
Significantly, perhaps, given the Tethyan aspect of the Junction Bed, the underlying Marlstone, from which fossils are easier to extract, contains a brachiopod fauna dominated by rhynchonellids with southern European affinities that are otherwise rare in Britain (Ager, Reference Ager1956). Showing a similar pattern of distribution, and also contained within the Marlstone, are certain gastropods including Discohelix, which is locally abundant in exposures in the Alps, Apennines and Sicily (Jackson, Reference Jackson1922, Reference Jackson1926; Hallam, Reference Hallam1967; cf. Wendt, Reference Wendt1968; Conti & Monari, Reference Conti and Monari2003).
Although difficult to access in coastal outcrops, fallen blocks of the Marlstone and Junction Bed can be examined on the foreshore to the east of the hamlet of Seatown in Dorset below Doghouse Hill (Fig. 1) and two such blocks were investigated in this study. Original stratigraphic orientation of the blocks is facilitated by the presence of the characteristic coarse-grained conglomeratic Marlstone welded to the base of the Junction Bed. Detailed study of the Marlstone and Junction Bed by Jackson (Reference Jackson1922, Reference Jackson1926) enabled recognition of a number of distinct layers with characteristic lithology and fauna, labelled with various letters of the alphabet, as illustrated in Figure 2 (Arkell, Reference Arkell1933; Cope et al. Reference Cope, Getty, Howarth, Morton and Torrens1980; Hesselbo & Jenkyns, Reference Hesselbo, Jenkyns and Taylor1995). The suggested stratigraphy of the two investigated blocks is shown in Figures 3 and 4. It should be noted that age determinations are not constant across the outcrop, a difference that will necessarily be reflected in the sedimentary record of the fallen blocks on the beach.

Fig. 3. Field aspect of Block 1, showing the contact between the Marlstone and the overlying Junction Bed (sensu stricto). Biostratigraphy is interpretative. Ammonite zones in italics.

Fig. 4. Field aspect of Block 2 (post-drilling), showing the contact between the Marlstone and the overlying Junction Bed (sensu stricto). Biostratigraphy is interpretative. Key as in Figure 3. Ammonite zones in italics. variab – variabilis.
The Junction Bed contains faunas representing all the zones of the Toarcian from the serpentinum (falciferum) to the levesquei. Significantly, however, the presence of the lower part of the exaratum subzone (of the serpentinum zone) has never been proven (Howarth, Reference Howarth1992). The time interval represented by the Marlstone and Junction Bed would have been characterized by environmental events around the Pliensbachian–Toarcian boundary including the Toarcian Oceanic Anoxic Event (T-OAE) and associated hyperthermal episode, although the potential of the condensed and incomplete nature of the sequence to capture the signature of these major phenomena is obviously open to question. This matter is addressed in the following account.
It should be noted that the Junction Bed and Marlstone in this area are in so-called ‘normal facies’ with well-ordered stratigraphy, albeit highly condensed; further to the east, in the proximity of the Eype’s Mouth Fault (Fig. 1), the sequence is further complicated by the presence of so-called ‘fissure facies’ that are represented by out-of-sequence neptunian sills of pale-coloured fine-grained locally ammonite-rich micrite and later void-filling calcite penetrating older grey-green to reddish-brown calcareous/ferruginous/quartz-bearing sedimentary units of the Marlstone–Junction Bed complex (Jenkyns & Senior, Reference Jenkyns and Senior1977, Reference Jenkyns and Senior1991; Raven & Dickson, Reference Raven and Dickson1989). The geometry of the multi-generational fissure fills indicates that this lithological complex was moderately consolidated when syn-sedimentary faulting and sedimentary disruption took place, implying some degree of lithification of the host sediment on or just below the sea floor that pre-dated significant burial. The Eype’s Mouth Fault is but one of a number of active extensional Jurassic faults that characterized the Wessex Basin during a period of active subsidence and suggests that the Marlstone and Junction Bed exposed on the Dorset coast were deposited on the relatively non-subsident part of a tilted block that probably had only modest effect on sea-floor topography but may have extended into the photic zone (probably ~200 m or less), allowing local development of microbially mediated stromatolites (Sellwood & Jenkyns, Reference Sellwood and Jenkyns1975; Chadwick, Reference Chadwick1986; Karner et al. Reference Karner, Lake, Dewey, Coward, Dewey and Hancock1987; Jenkyns & Senior, Reference Jenkyns and Senior1991; cf. Jenkyns, Reference Jenkyns1971; Vera and Martin-Agarra, Reference Vera, Martín-Algarra, Bertrand-Sarfati and Monty1994; Massari and Westphal, Reference Massari, Westphal, Tewari and Seckbach2011; Reolid, Reference Reolid2011).
2. Methodology
The two fallen blocks investigated were oriented and described in the field with samples of bulk rock obtained using a cordless drill positioned every centimetre or less and the resultant powder put into sample bags. The tungsten carbide drill bit was cleaned with dilute HCl after each use to avoid cross-contamination. To obtain some primary skeletal calcite, all observed belemnites were collected from the sections using a hammer and chisel.
The powders thus obtained from bulk rock were analysed on a VG Isogas Series Prism Mass Spectrometer at Oxford University for carbon- and oxygen-isotope ratios using standard techniques, as described in Woodfine et al. (Reference Woodfine, Jenkyns, Sarti, Baroncini and Violante2008). Analytical reproducibility of replicate standards (Carrara Marble) on the instrument was typically less than 0.1 ‰ for both carbon- and oxygen-isotope ratios.
Belemnites were prepared as in the method of Jones et al. (Reference Jones, Jenkyns and Hesselbo1994) for strontium-isotope analyses, samples of 100–200 mg being first cleaned to remove matrix, crushed and repeatedly etched in 0.3M HCl to remove diagenetic calcite, followed by rinsing in distilled water. Subsequently, some fossil fragments were set aside for carbon- and oxygen-isotope analysis, the rest being dissolved in 6 % HNO3 (vol.–vol. %) before undergoing further dilution with 1 % HNO3 and addition of an indium internal standard. Solutions were analysed for a suite of trace and major elements (including Mg, Ca, Mn, Fe) on a Perkin Elmer Sciex Elan 6100 DRC Quadrupole inductively coupled plasma mass spectrometer (ICP-MS) at Oxford University following the technique of Rosenthal et al. (Reference Rosenthal, Boyle and Slowey1997). Typical analytical uncertainty for Mg/Ca on this instrument, obtained from the standard deviation of a synthetic standard, is ∼2.4 % (e.g. Ferguson et al. Reference Ferguson, Henderson, Kucera and Rickaby2008).
Strontium was separated from pre-prepared solutions using standard procedures (Jones et al. Reference Jones, Jenkyns and Hesselbo1994) and then loaded into a VG Isomass 54E solid-state thermal ionization mass spectrometer (TIMS). Results were normalized to an NIST SRM 987 standard value of 0.710250. The long-term (3 years) average of the standard over the period of analysis was 0.710248 with a 95 % confidence interval (2σ) of 2.6 × 10−6. All samples contained less than 150 ppm Fe, the cut-off used by Jones et al. (Reference Jones, Jenkyns and Hesselbo1994) to exclude potentially altered fossils likely to give elevated strontium-isotope ratios and plot above the reference curve. The only belemnite with an Fe value in excess of 100 ppm, which also has an anomalous Mn value of 965 ppm, is at the 20 cm level in Block 2.
The data generated (carbon- and oxygen-isotope ratios of bulk carbonate and belemnites, strontium-isotope ratios of belemnites, Mg:Ca ratios of belemnites) have been plotted against the lithological log of the Marlstone and Junction Bed (Figs 5, 6).

Fig. 5. Chemostratigraphy of Block 1. Note the close correspondence between the carbon- and oxygen-isotope profiles, compatible with a significant diagenetic overprint in the Marlstone and Junction Bed. The strontium-isotope ratios (87Sr/86Sr) of the belemnites conform to global trends; the oxygen-isotope patterns and Mg:Ca ratios indicate the onset of the Toarcian hyperthermal across the Pliensbachian–Toarcian boundary. Solid circles indicate samples of matrix; vertically oriented bullet-shaped symbols of variable length denote belemnites and the thickness of strata in which the fossils were found. Strontium-isotope error bars are 2σ. Ammonite zones in italics.

Fig. 6. Chemostratigraphy of Block 2. Note the close correspondence between the carbon- and oxygen-isotope profiles, compatible with a significant diagenetic overprint in the Marlstone and Junction Bed. The strontium-isotope ratios of the belemnites generally conform to global trends although the two, stratigraphically highest samples could be reworked. The belemnite at the 20 cm level (coloured orange) contains elevated contents of Fe and Mn and is considered to be diagenetically compromised and not reliable as a chemostratigraphic index as regards 87Sr/86Sr and Mg:Ca. The oxygen-isotope patterns and Mg:Ca ratios indicate the onset of the Toarcian hyperthermal across the Pliensbachian–Toarcian boundary, particularly clearly in the case of the latter proxy, whose profile resembles that seen in Block 1. Solid circles indicate samples of matrix; vertically oriented bullet-shaped symbols of variable length denote belemnites and the thickness of strata in which the fossils were found. Strontium-isotope error bars are 2σ. Ammonite zones in italics.
3. Chemostratigraphic data
3.a. Carbon isotopes in bulk carbonate
In Block 1, the bulk carbon-isotope ratios in the Marlstone, of spinatum–tenuicostatum zone age, are relatively low, with most values falling between −4 ‰ and −3 ‰ (Fig. 5). There is an abrupt shift across the contact with the overlying finer grained Junction Bed where values are consistently higher, generally in the range 1–2 ‰. Such values persist up to the half-way level of the Junction Bed, of probable serpentinum zone age, where there is a pronounced negative excursion with values falling to ∼ −6 ‰ at around the 33–35 cm level. Following recovery from this negative shift, values return to background levels of 1–2 ‰. Close to the top of the block, values fall again with a minimum figure of −1.2 ‰ present at one level before recovering somewhat in the three highest samples.
A similar range of carbon-isotope values is present in Block 2 but the pattern is slightly different in that there is a negative excursion within the Marlstone interval from a background of ∼ −2 to ~ –3 ‰ down to −4.5 ‰ before a rise up to just below the contact with the Junction Bed with values in the range 1–1.5 ‰ (Fig. 6). Stratigraphically higher background values in the Junction Bed are similar to those in Block 1 but there are also two negative excursions down to ∼ −2 ‰ at around the 10–13 cm and 24–25 cm levels, both likely localized in the serpentinum zone: these values are considerably less negative than the lowest values seen in Block 1.
In a coeval section, a little more stratigraphically expanded than the sequences exposed on the Dorset coast (as exemplified by Blocks 1 and 2), and cropping out some 30 km to the northeast in Somerset (Preston Plunknett, near Yeovil), the carbon isotopes rise from a background value of between −0.5 ‰ and 0 ‰ in the Marlstone (spinatum–tenuicostatum zones) to a maximum of >3 ‰ in the lower part of the serpentinum zone of the Junction Bed before declining through the bifrons zone ultimately into the thouarsense zone (Jenkyns & Clayton, Reference Jenkyns and Clayton1997). Such isotopic values are in general a little higher than those found in Blocks 1 and 2.
3.b. Carbon isotopes in belemnites
Carbon isotopes of the belemnites in Block 1 show little variation, apart from a minor excursion at around the 15 cm level, with most values falling in the range 1 ‰ to 3 ‰: figures that in most, but not all cases are higher than values from the enclosing bulk carbonate (Fig. 5). Belemnite values in Block 2 are similar (Fig. 6).
3.c. Oxygen isotopes in bulk carbonate
In Block 1, bulk oxygen-isotope ratios are relatively constant in the Marlstone at around −3 ‰ (Fig. 5). Following this, apart from one anomalously low value, there is a step up across the contact with the Junction Bed to around −1 ‰ followed by a drop back to ∼ −2 ‰, and such values then generally increase in progressively higher levels towards −1 ‰, punctuated by a distinct negative excursion in the putative serpentinum zone where values drop to ∼ −4 ‰ at around the 33–35 cm level. These low values correlate with low values in the carbon-isotope profile and, in the higher stratigraphic values of the block, the oxygen and carbon isotopes also move in parallel, including the top four values that depart from the overall trend.
Across Block 2, the bulk oxygen-isotope profile also closely matches that of the carbon isotopes (Fig. 6). Overall, against a background of ∼ −2 ‰, there are three negative excursions with values falling to ∼ −3.5 ‰ in the Marlstone (spinatum–tenuicostatum zones) and to ∼ −3.8 ‰ and ∼ −2.5 ‰ at the 10–13 cm and 24–25 cm levels, respectively in the overlying putative serpentinum zone in the Junction Bed itself.
3.d. Oxygen isotopes in belemnites
The oxygen isotopes in the belemnites in Block I (Fig. 5) fall between 0 ‰ and −1 ‰ in the Marlstone, followed up-section by a dramatic fall of ∼2 ‰ crossing into the basal Junction Bed. Values become generally heavier thereafter in higher levels, albeit remaining relatively low in the putative serpentinum zone. The major drop in oxygen-isotope values of belemnites correlates with an increase in Mg/Ca ratios in the same fossils.
Oxygen-isotope values in the belemnites over the whole of Block 2 (Fig. 6) are mostly around −1 ‰ and are characterized by a ∼1 ‰ drop over the Marlstone–Junction Bed contact where the belemnite abundance tolerably well defines the trend. This interval correlates with an increase in belemnite Mg:Ca, as is the case for Block 1.
3.e. Strontium isotopes in belemnites
In Block 1 (Fig. 5), strontium-isotope ratios (87Sr/86Sr) in belemnites from the Marlstone (spinatum–tenuicostatum zones) show a subdued decline from 0.707097 to 0.707003 before rising abruptly in the base of the Junction Bed to 0.707198, and similar values persist to the top of the section with the highest figure obtained of 0.707237 in the putative bifrons–variabilis zones. Two separate belemnites at the 28 cm level give values of 0.707161 and 0.707189.
The pattern in Block 2 (Fig. 6) is not as clear-cut but, again, there are higher values within belemnites from the Junction Bed than those in the Marlstone. Values within the Marlstone fossils fall within the range 0.707072 to 0.707121 and two separate belemnites at the 9 cm mark have values of 0.707061 and 0.70711. At the level of its contact with the Junction Bed, belemnite values increase to the 0.707122–0.707156 range. Stratigraphically above, values rise to a maximum of 0.707218 with the sample at the 20 cm level, this being the belemnite with the highest concentrations of Fe and Mn, before illustrating a gentle decline.
3.f. Mg:Ca ratios in belemnites
In both Blocks 1 and 2, there is a dramatic increase in belemnite Mg:Ca ratios across the Marlstone–Junction Bed contact (Figs 5, 6). Values rise from a general background of ∼8–10 mmol/mol to mostly in the range of 18–20 mmol/mol. In Block 1, the highest value is at the top of the section (∼24 mmol/mol), lying in the putative bifrons–variabilis zones. In Block 2, the highest value (21.6 mmol/mol) is at the 20 cm mark in the putative serpentinum zone, but it should be noted that this sample has elevated levels of Fe and Mn. In Block 1, the major rise in Mg:Ca ratios of belemnites correlates with a dramatic decrease in oxygen-isotope ratios in the same fossils, a relationship also seen in Block 2. This correlation extends to the strontium-isotope profiles whose belemnites show an abrupt rise at the same level, most obviously displayed in Block 1 (Fig. 5). The apparent synchronous nature of these different geochemical signals is probably an artefact of the extremely condensed nature of the Junction Bed and presumably the underlying Marlstone.
4. Discussion
4.a. Strontium-isotope stratigraphy
The chemostratigraphic significance of strontium-isotope ratios in belemnites can be prone to error. In their detailed study of Lower Jurassic condensed pelagic limestones from the Subbetic Zone of southern Spain, Nieto et al. (Reference Nieto, Ruiz-Ortiz, Rey and Benito2008) illustrated instances where the strontium-isotope age of the belemnites, as defined by a high-resolution reference curve (e.g. Jones et al. Reference Jones, Jenkyns and Hesselbo1994; McArthur et al. Reference McArthur, Donovan, Thirlwall, Fouke and Mattey2000, Reference McArthur, Howarth and Bailey2001; Jenkyns et al. Reference Jenkyns, Jones, Gröcke, Hesselbo and Parkinson2002), departs from the biostratigraphical age given by the associated ammonites. It seems that, in settings of particularly slow net deposition, relatively robust belemnites can undergo selective reworking up-section owing to winnowing of accompanying sediment and/or bioturbation. Such derived faunas (unaccompanied by coeval more fragile and easily dissolved ammonites) hence give a greater age than does the ultimately entombing sediment and contained younger ammonites. The reverse can also occur, in which case it may be the ammonites, commonly coated with a protective veneer of iron-manganese oxyhydroxides, that are reworked and give the greater age. It is also possible that belemnites can become cemented to the sea floor during hardground formation and, because there is no net deposition for some while, when ammonite-bearing sediment finally does accumulate it is younger than the belemnites that it encloses. The highly condensed nature of the Junction Bed makes it likely that some or all of these processes may have operated during the Toarcian, thereby compromising to some extent the use of strontium-isotope stratigraphy of the belemnites as a dating tool. Some level of diagenetic modification is possible for all belemnites sampled, a process that in general will tend to move the strontium-isotope ratios to more radiogenic values, thereby confounding their stratigraphic significance to some extent (Jones et al. Reference Jones, Jenkyns and Hesselbo1994).
These potential complications notwithstanding, it is apparent that the strontium-isotope ratios in both blocks record chemostratigraphically the boundary between the Pliensbachian and Toarcian and the higher levels of the latter stage (cf. Fig. 7). As illustrated by Jones et al. (Reference Jones, Jenkyns and Hesselbo1994) and in great detail by McArthur et al. (Reference McArthur, Donovan, Thirlwall, Fouke and Mattey2000), in both cases based on more stratigraphically expanded sections from Yorkshire, the overall trend is for strontium-isotope ratios to fall through the Pliensbachian stage to reach a minimum value of ∼0.707070 at the top of the spinatum/base of the tenuicostatum zone, a ratio that is regionally applicable across Europe, before rising through the whole of the following Toarcian stage to increasingly radiogenic values (cf. McArthur et al. Reference McArthur, Page, Duarte, Thirlwall, Li, Weis and Comas-Rengifo2019). An even lower figure (0.707003) is recorded from near the top of the Marlstone in Block 1 (Fig. 5), which must derive from an altered fossil given that this ratio is less than any Lower Jurassic value known from Europe (Fig. 7). Parenthetically, it may be noted that a diagenetic shift to lower than seawater values in belemnite calcite has been reported from Canada and New Zealand, where the interaction of the skeletal material with hydrothermal fluids has been postulated (Gröcke et al. Reference Gröcke, Hesselbo and Findlay2007; Ullmann et al. Reference Ullmann, Campbell, Frei, Hesselbo, von Strandmann and Korte2013 a). By contrast, the stratigraphically next highest belemnite on Block 1 has a value of 0.707198 (at 15–15.5 cm) that, at face value, places it around the serpentinum–bifrons zone boundary by comparison to reference curves. However, this seems unlikely to be a case of a stratigraphically adrift fossil (or matrix) and more probably the skeletal calcite has also been diagenetically modified somewhat, in this case to more radiogenic values, as the overlying three belemnites give relatively consistent values securely in the serpentinum zone. The succeeding next three belemnites, being the highest in the block, suggest the presence of the bifrons zone, possibly extending into the variabilis zone (Fig. 5).

Fig. 7. Strontium-isotope reference curve based on diagenetically screened belemnites from diverse localities except for the ‘England – O’ data points that indicate non-screened oysters as the source of skeletal calcite (after Jenkyns et al. Reference Jenkyns, Jones, Gröcke, Hesselbo and Parkinson2002). Stratigraphical extent of the Marlstone and Junction Bed, from the spinatum to the levesquei zone, derives from ammonite determinations (Cope et al. Reference Cope, Getty, Howarth, Morton and Torrens1980; Jenkyns & Senior, Reference Jenkyns and Senior1991; Howarth, Reference Howarth1992). Note the strontium-isotope minimum close to the Pliensbachian–Toarcian boundary, followed by the subsequent rise to progressively more radiogenic values up to the Aalenian, trends most clearly seen in Block 1 (Fig. 5). Together, belemnites from the Marlstone and Junction Bed record this major change in the balance between continental and mantle (mafic) sources of strontium to the world ocean, with increase in the former potentially linked to accelerated weathering of the land surface due to the high global temperatures of the Toarcian hyperthermal. All strontium-isotope data are normalized to a value of 0.710250 for the NBS 987 standard. Duration of stages is plotted according to the number of subzone units (Jones et al. Reference Jones, Jenkyns and Hesselbo1994).
The strontium-isotope pattern in Block 2 (Fig. 6) shows the same general pattern as in Block 1, with relatively low values (but no obvious trend) in the basal four belemnites in the Marlstone, compatible with a late Pliensbachian age, followed in the Junction Bed by a relatively abrupt rise to more radiogenic values typical of the Toarcian (Fig. 7). The two stratigraphically highest belemnites show values of 0.707188 and 0.707158, suggesting that the serpentinum zone possibly extends to this level. Accepted at face value, this is incompatible with a ratio of 0.707218, the highest recorded value in the section, in the underlying belemnite (at the 20 cm level) that would place it in the bifrons zone. Either some diagenetic increase in strontium-isotope ratios is the case for this belemnite and/or the two stratigraphically highest belemnites have been differentially reworked from a stratigraphically lower level, which could explain the up-section fall in values as opposed to the expected rise (cf. McArthur et al. Reference McArthur, Donovan, Thirlwall, Fouke and Mattey2000). Notably, the belemnite analysed in the section at the 20 cm level is unusual in containing relatively elevated Fe and Mn contents, and is consequently probably altered and not reliable as a chemostratigraphic marker. Hence, most of the Junction Bed sampled in Block 2, which is thinner and probably less stratigraphically extensive than Block 1, probably pertains to the serpentinum zone, although the presence of higher zones at the top of the section is well possible, as tentatively indicated in Figure 6.
Overall, the strontium-isotope pattern in the lower Toarcian is most simply interpreted as recording a relative increase in the impact of global continental weathering and consequent fluvial input to the oceans as a major source of strontium, thereby outcompeting its input from basalt–seawater interaction such as submarine volcanism and/or sea-floor spreading (Jenkyns, Reference Jenkyns2003, Reference Jenkyns2010; Ullmann et al. Reference Ullmann, Hesselbo and Korte2013 b).
4.b. Diagenesis
Examination of the carbon- and oxygen-isotope stratigraphic profiles of both Block 1 and Block 2 shows a high degree of parallelism, being particularly striking in the case of the negative excursions, of which there are several (Figs 5, 6). Such relationships are well illustrated in cross-plots (Fig. 8), in which additional end-member data are supplied from the belemnites constituting primary skeletal material and also diagenetic void-filling sparry calcite from the fissure facies of the Junction Bed/Marlstone complex sampled adjacent to the Eype’s Mouth Fault (Jenkyns & Senior, Reference Jenkyns and Senior1991). In Block 1, carbon-isotope values of most belemnites fall between 2 ‰ and 3 ‰ and most oxygen-isotope values between −2.5 ‰ and −0.4 ‰; in Block 2, carbon-isotope values of most belemnites fall between 1.7 ‰ and 3.0 ‰ and most oxygen-isotope values between −1.4 ‰ and −0.4 ‰. Carbon-isotope values of the void-filling calcite range between −2.71 ‰ and −10.23 ‰ with oxygen-isotope values ranging between −3.5 ‰ and −6.3 ‰. Given that the bulk carbonate isotopes of the Marlstone and Junction Bed lie in an intermediate position in terms of values, the data in the cross-plot can be interpreted as a two-component mixing ‘line’ representing different relative amounts of primary carbonate of biological origin and later diagenetic cements (cf. Marshall, Reference Marshall1992).
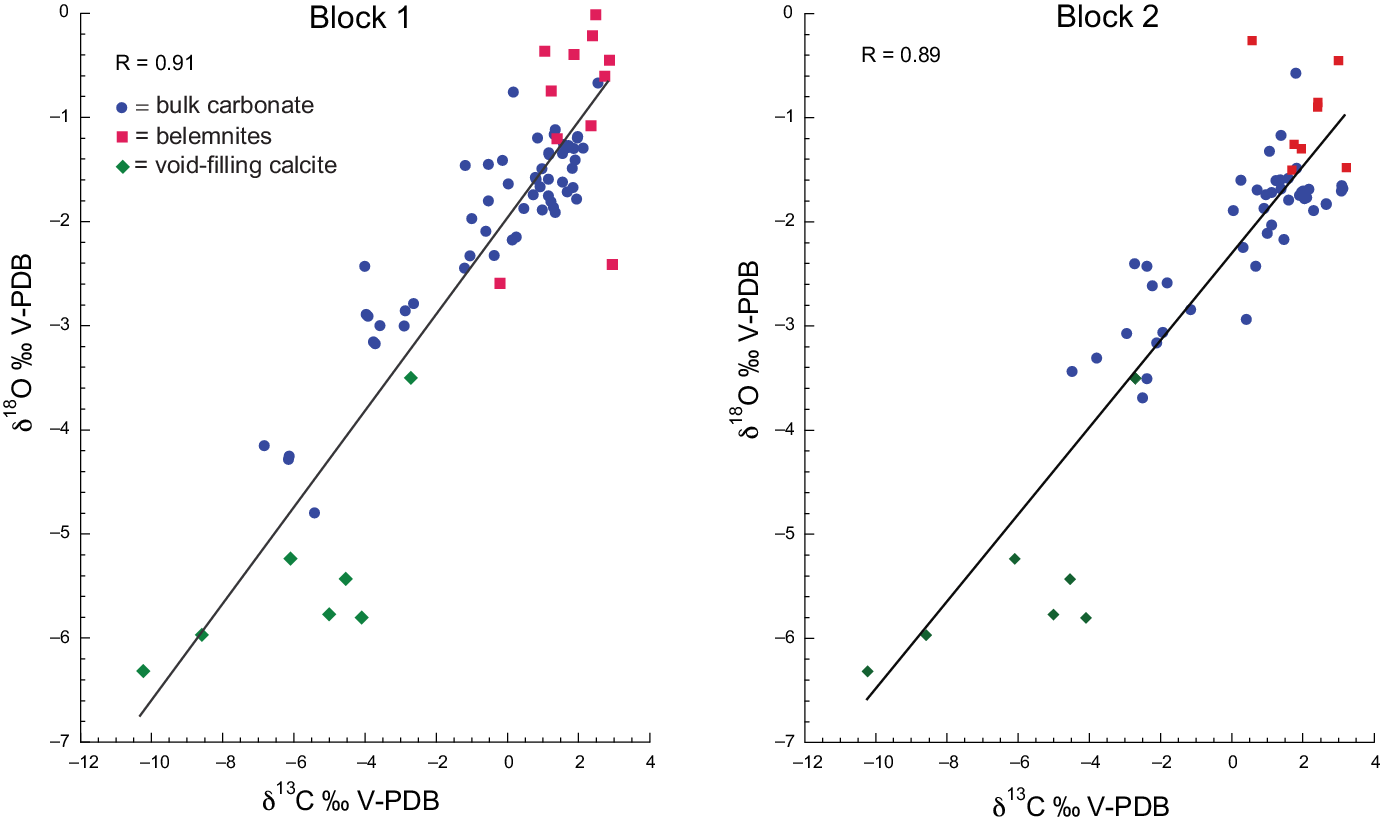
Fig. 8. Cross-plot of carbon and oxygen isotopes of bulk carbonate and belemnites from Blocks 1 and 2 and of diagenetic void-filling calcite from fissure facies sampled close to the Eype’s Mouth Fault. The high values of the correlation coefficient in both plots is compatible with the matrix of the Marlstone and Junction Bed being formed primarily from a mixture of biological carbonate and diagenetic cement, the latter probably formed both on the sea floor and in the burial environment. Solid circles are bulk carbonate; solid squares are belemnites; solid diamonds are samples of diagenetic void-filling calcite from neptunian sills from close to the Eype’s Mouth Fault.
At least some of this diagenetic cement probably formed at or just below the sea floor, as indicated by the relative consolidation of the Marlstone/Junction Bed matrix injected by the neptunian sills next to the Eype’s Mouth Fault. Additional evidence was cited by Jackson (Reference Jackson1922) and Arkell (Reference Arkell1933), who noted that vertically or sub-vertically planed-off ammonites in the Junction Bed, whose lower parts were held in micritic matrix, implied consolidation before syn-sedimentary erosion took place. Indeed, the overall slow net depositional rates of the Junction Bed would have been one of the prerequisites for open-marine submarine lithification (e.g., Milliman, Reference Milliman1966; Fischer & Garrison, Reference Fischer and Garrison1967). However, the relatively negative carbon- and oxygen-isotope values of the fissure-fill calcitic cements, typically ferroan, suggest a subsequent late-stage component, probably derived from fluids formed by compacting and dewatering of underlying organic-rich shales and transferred along the conduit of the Eype’s Mouth Fault (Raven & Dickson, Reference Raven and Dickson1989; Jenkyns & Senior, Reference Jenkyns and Senior1991). The relative importance of these possible processes as regards the lithification of the Junction Bed in normal facies, well separated from any fault is, however, not established. Notably, at Preston Plunknett (near Yeovil, Somerset), the carbon-isotope values of the serpentinum zone in the Junction Bed are ∼1 ‰ heavier than those recorded in Blocks 1 and 2 and described herein (cf. Jenkyns & Clayton, Reference Jenkyns and Clayton1997), suggesting a more minor role for late-stage diagenesis in at least some sections inland.
By contrast, the bulk carbon-isotope values in Toarcian coccolith-bearing pelagic red limestones from the Tethyan Jurassic of southern Europe (Italy, Greece) locally attain values of up to 4.5 ‰ in the serpentinum zone, and coeval belemnites from the organic-rich Jet Rock in Yorkshire attain values that exceed 6 ‰ in the upper exaratum subzone (Jenkyns & Clayton, Reference Jenkyns and Clayton1986, Reference Jenkyns and Clayton1997; Jenkyns et al. Reference Jenkyns, Jones, Gröcke, Hesselbo and Parkinson2002; Sabatino et al. Reference Sabatino, Neri, Bellanca, Jenkyns, Baudin, Parisi and Masetti2009; Kafousia et al. Reference Kafousia, Karakitsios, Mattioli, Kenjo and Jenkyns2014). These figures are considerably in excess of the 1–2 ‰ background values of the Junction Bed exposed on the Dorset coast, equally suggesting possession of a considerable diagenetic overprint. The unusually high values in the Yorkshire belemnites, however, may relate to their having lived in somewhat isolated stratified basins where deposition of organic matter resulted in unusually elevated carbon-isotope values of local watermasses not characteristic of the open ocean (McArthur et al. Reference McArthur, Algeo, van de Schootbrugge, Li and Howarth2008; Dickson et al. Reference Dickson, Gill, Ruhl, Jenkyns, Porcelli, Idiz, Lyons and van den Boorn2017; Remirez & Algeo, Reference Remírez and Algeo2020).
4.c. The Toarcian Oceanic Anoxic Event
Recognition of the signature of the early Toarcian Oceanic Anoxic Event (T-OAE) in any lithological sequence relies, in the first instance, on its exact definition. Using a combination of enhanced enrichment in organic carbon in globally distributed outcrops, and the accompanying carbonate and organic-matter carbon-isotope signature from a number of localities, suggests a duration from the mid-tenuicostatum zone (over which interval carbon-isotope values are generally rising) to the mid-serpentinum zone where values are typically at their highest, thereby taking in the intervening pronounced stepped negative carbon-isotope excursion that characterizes the T-OAE and interrupts the overarching positive trend (e.g. Jenkyns & Clayton, Reference Jenkyns and Clayton1997; Hesselbo et al. Reference Hesselbo, Gröcke, Jenkyns, Bjerrum, Farrimond, Morgans Bell and Green2000; Hermoso et al. Reference Hermoso, Le Callonnec, Minoletti, Renard and Hesselbo2009; Jenkyns, Reference Jenkyns2010; Kemp et al. Reference Kemp, Coe, Cohen and Weedon2011; Xu et al. Reference Xu, Ruhl, Jenkyns, Leng, Huggett, Minisini, Ullmann, Riding, Weijers, Storm and Percival2018; Storm et al. Reference Storm, Hesselbo, Jenkyns, Ruhl, Ullmann, Xu, Leng, Riding and Gorbanenko2020 for UK sequences). In northern and southern European outcrops, the most organic-rich level, typically developed as millimetre-laminated black shale, lies primarily in the lower part of the exaratum subzone of the serpentinum zone or its stratigraphic equivalents (Jenkyns et al. Reference Jenkyns, Jones, Gröcke, Hesselbo and Parkinson2002).
The Junction Bed, with its prevailing red, pink, brown and yellow colours, suggests oxic conditions prevailed from the time of deposition into the burial environment: evidence that could be taken to indicate that no organic-rich sediment was ever deposited in this part of the Wessex Basin. It is, of course, possible that the particular palaeogeographic position of the Marlstone and Junction Bed in coastal Dorset, on a relatively non-subsiding sector of a tilted fault-block, was critical in this regard. In such a case, the sea floor, being possibly somewhat shallower than surrounding areas, may have remained persistently current-swept and oxic even if fluxes of organic matter through the water column were relatively elevated at the time.
However, the apparent absence of the lower exaratum subzone in the Junction Bed (Howarth, Reference Howarth1992) indicates that the key interval is likely represented by a hiatus, compatible with an interpretation that organic-rich sediment was initially deposited but was subsequently removed by sea-floor erosion. A little to the east (∼40 km) of the outcrops on the Dorset coast, a somewhat more expanded section of the Junction Bed was encountered in the Winterborne Kingston Borehole where the T-OAE level is represented by shaly sediments containing up to 4 % total organic carbon (Jenkyns & Clayton, Reference Jenkyns and Clayton1997). Data from other wells in the region, including those in the offshore English Channel, include descriptions of lowermost Toarcian dark-coloured organic-rich ‘paper shales’ stratigraphically adjacent to the Junction Bed (Ainsworth et al. Reference Ainsworth, Braham, Gregory, Johnson, King and Underhill1998; Ainsworth & Riley, Reference Ainsworth and Riley2010). Together, these records indicate that the major palaeoceanographic phenomenon represented by the T-OAE impacted the waters of the Wessex Basin, although the lack of its characteristic sedimentary signature in the Junction Bed of the Dorset coast cannot be ascribed unambiguously to any particular process.
Given that a pronounced negative carbon-isotope excursion, typically interrupting an overarching positive trend, is a feature of the T-OAE worldwide and recorded in pelagic and shallow-water carbonate, marine and terrestrial organic matter (e.g. Jenkyns & Clayton, Reference Jenkyns and Clayton1997; Kemp et al. Reference Kemp, Coe, Cohen and Schwark2005; Hesselbo et al. Reference Hesselbo, Jenkyns, Duarte and Oliveira2007; Woodfine et al. Reference Woodfine, Jenkyns, Sarti, Baroncini and Violante2008; Hermoso et al. Reference Hermoso, Le Callonnec, Minoletti, Renard and Hesselbo2009; Al-Suwaidi et al. Reference Al-Suwaidi, Angelozzi, Baudin, Damborenea, Hesselbo, Jenkyns, Manceñido and Riccardi2010; Caruthers et al. Reference Caruthers, Gröcke and Smith2011; Sabatino et al. Reference Sabatino, Vlahović, Jenkyns, Scopelliti, Neri, Prtoljan and Velić2013; Kemp & Izumi, Reference Kemp and Izumi2014; Bodin et al. Reference Bodin, Krencker, Kothe, Hoffmann, Mattioli, Heimhofer and Kabiri2016; da Rocha et al. Reference da Rocha, Mattioli, Duarte, Pittet, Elmi, Mouterde, Cabral, Comas-Rengifo, Gómez, Goy, Hesselbo, Jenkyns, Littler, Mailliot, de Oliveira, Osete, Perilli, Pinto, Ruget and Suan2016; Them et al. Reference Them, Gill, Caruthers, Gröcke, Tulsky, Martindale, Poulton and Smith2017; Xu et al. Reference Xu, Ruhl, Jenkyns, Hesselbo, Riding, Selby, Naafs, Weijers, Pancost, Tegelaar and Idiz2017, Reference Xu, Ruhl, Jenkyns, Leng, Huggett, Minisini, Ullmann, Riding, Weijers, Storm and Percival2018; Han et al. Reference Han, Hu, Kemp and Li2018; Ikeda et al. Reference Ikeda, Hori, Ikehara, Miyashita, Chino and Yamada2018; Kemp et al. Reference Kemp, Selby and Izumi2020), it is possible that this signal is recorded in the Junction Bed. Where well dated, the core of the negative excursion lies in the basal exaratum subzone or equivalent (basal serpentinum zone), which is the interval that appears to be unrecorded in the Junction Bed and is likely lost to a hiatus (Howarth, Reference Howarth1992). It is, however, possible that some elements of the negative carbon-isotope excursion (e.g. a rising and/or falling limb) are recorded in the Junction Bed and the pronounced shift at the 30–35 cm in Block 1 would be a possible candidate, given its likely position somewhere in the serpentinum zone, although this appears to be rather too high in the stratigraphy and could equally well be a diagenetic artefact. Of the negative excursions displayed in Block 2, all of which could also be entirely diagenetic in origin, that centred close to the boundary between the Marlstone and Junction Bed, probably extending into the basal serpentinum zone, is perhaps most likely to be a partial record of the T-OAE negative excursion. Correlation with lowered oxygen-isotope values and increased Mg:Ca ratios (Figs 5, 6) in this case could signify increased palaeotemperatures known to characterize this interval, as discussed below.
The general relatively low values of carbon isotopes in the underlying Marlstone seen in Blocks 1 and 2 (Figs 5, 6) could possibly be recording the negative excursion recently recognized in the spinatum zone (Storm et al. Reference Storm, Hesselbo, Jenkyns, Ruhl, Ullmann, Xu, Leng, Riding and Gorbanenko2020), although the parallel shift in oxygen isotopes is equally compatible with a diagenetic interpretation. Other potential relatively minor carbon-isotope disturbances, such as those recorded from around the Pliensbachian–Toarcian boundary (Littler et al. Reference Littler, Hesselbo and Jenkyns2010), cannot be positively identified in either block.
4.d. Palaeotemperatures: the Toarcian hyperthermal
The oxygen-isotope and Mg:Ca records of the belemnites potentially contain climatic information and point to a major increase in palaeotemperature across the Pliensbachian–Toarcian boundary or a little later. The signal is clearest in Block 1 (Fig. 5), which illustrates a dramatic drop in oxygen-isotope values of ∼2.4 ‰ with an accompanying jump in Mg:Ca ratios of ∼10 mmol/mol in the lower serpentinum zone. The oxygen-isotope record, following the palaeotemperature equation of Anderson & Arthur (Reference Anderson, Arthur, Arthur, Anderson, Kaplan, Veizer and Land1983), translates into a rise of ∼10 °C from a background of ∼14 °C (Fig. 9). The incorporation of Mg into skeletal calcite is both temperature- and taxon-dependent and is hence unknown for extinct organisms such as belemnites, but application of the analysis of Bailey et al. (Reference Bailey, Rosenthal, McArthur, van de Schootbrugge and Thirlwall2003), who studied a large collection of these fossils from Yorkshire and Germany for Mg:Ca and oxygen-isotope ratios and found similar values to those given here, suggested that a temperature rise of 10–11 °C was plausible for the Wessex Basin. Recourse to the bivalve-derived Mg:Ca palaeotemperature equation of Klein et al. (Reference Klein, Lohmann and Thayer1996) as applied to the belemnite data, given that both organisms belong to the same phylum, suggests a slightly greater rise (∼15 °C) from a background of ∼12 °C for Block 1 (Fig. 9). Higher in the section in Block 1, the signals diverge, with oxygen isotopes showing a general rise in values, potentially indicating cooler temperatures, whereas Mg:Ca ratios remain relatively high. The stratigraphically highest belemnite, if the proxy data are accepted at face value, suggests another increase in temperature in the bifrons zone or stratigraphically above, with the higher values indicated by the Mg:Ca ratios (Fig. 9).

Fig. 9. Palaeotemperature reconstructions from oxygen-isotope values and Mg:Ca ratios from belemnites in Block 1. Oxygen-isotope-derived palaeotemperatures calculated using the formula of Anderson & Arthur (Reference Anderson, Arthur, Arthur, Anderson, Kaplan, Veizer and Land1983) applying a value of −1 ‰smow for Mesozoic seawater; palaeotemperatures from Mg:Ca ratios in belemnites derived using the bivalve-based equation of Klein et al. (Reference Klein, Lohmann and Thayer1996). Ammonite zones in italics.
The Mg:Ca pattern in Block 2 (Fig. 6) is rather similar to Block 1, although the oxygen-isotope shift is less pronounced. The increase in Mg:Ca ratios in the basal Toarcian is ∼10 mmol/mol; the fall in oxygen-isotope ratios is ∼1 ‰. Taken at face value, these figures suggests a more modest rise in temperature than do the data from Block 1 (Fig. 10), but are most likely a manifestation of the innate variability of the two proxies in question, particularly as changes in salinity operating on a variety of time scales could have been associated with both of them (Bailey et al. Reference Bailey, Rosenthal, McArthur, van de Schootbrugge and Thirlwall2003; Rosales et al. Reference Rosales, Robles and Quesada2004 b; Ferguson et al. Reference Ferguson, Henderson, Kucera and Rickaby2008).

Fig. 10. Palaeotemperature reconstructions from oxygen-isotope values and Mg:Ca ratios from belemnites in Block 2. Oxygen-isotope-derived palaeotemperatures calculated using the formula of Anderson & Arthur (Reference Anderson, Arthur, Arthur, Anderson, Kaplan, Veizer and Land1983) applying a value of −1 ‰smow for Mesozoic seawater; palaeotemperatures from Mg:Ca ratios in belemnites derived using the bivalve-based equation of Klein et al. (Reference Klein, Lohmann and Thayer1996). Ammonite zones in italics.
Other potentially confounding factors implicated as controls on Mg:Ca ratios in belemnites include species-specific differences in the molluscs themselves, metabolic effects on trace-element incorporation, growth rates and diagenesis (McArthur et al. Reference McArthur, Doyle, Leng, Reeves, Williams, Garcia-Sanchez and Howarth2007; Li et al. Reference Li, McArthur and Atkinson2012; Immenhauser et al. Reference Immenhauser, Schoene, Hoffmann and Niedermayr2016; Ullmann & Pogge von Strandmann, Reference Ullmann and Pogge von Strandmann2017). The effects of diagenesis are most obviously seen in the belemnite from the 20 cm level in Block 2, whose Fe and Mn contents are also relatively elevated and whose strontium-isotope values appear anomalous (Fig. 6).
There is no doubt, however, that the vast range of data from palynology, particularly from the former Soviet Union, oxygen-isotope data from skeletal calcite (European belemnites and brachiopods), locally elevated levels of kaolinite in clay-mineral assemblages in north European sections, as well as novel TEX86 data (a rise of ∼10 °C from a background of ∼22 °C, based on sections in Italy and Spain) and novel clumped isotope measurements (with a model increase of 13 ± 4 °C from the spinatum to serpentinum zones in belemnites from SW Germany), points to considerable warmth being a feature of the early Toarcian climate, thereby qualifying it as one of the major Mesozoic hyperthermals, as well as recording increased continental weathering, an oceanic anoxic event and an episode of ocean acidification (e.g. Vakhrameyev, Reference Vakhrameyev1982; Jenkyns & Clayton, Reference Jenkyns and Clayton1997; McArthur et al. Reference McArthur, Donovan, Thirlwall, Fouke and Mattey2000; Jenkyns, Reference Jenkyns2003, Reference Jenkyns2010; Rosales et al. Reference Rosales, Quesada and Robles2004 a; Suan et al. Reference Suan, Mattioli, Pittet, Lécuyer, Suchéras-Marx, Duarte, Philippe, Reggiani and Martineau2010, Reference Suan, Nikitenko, Rogov, Baudin, Spangenberg, Knyazev, Glinskikh, Goryacheva, Adatte, Riding and Föllmi2011; Gomez & Goy, Reference Gómez and Goy2011; Dera et al. Reference Dera, Brigaud, Monna, Laffont, Pucéat, Deconinck, Pellenard, Joachimski and Durlet2011 a; Hermoso et al. Reference Hermoso, Minoletti, Rickaby, Hesselbo, Baudin and Jenkyns2012; Hönisch et al. Reference Hönisch, Ridgwell, Schmidt, Thomas, Gibbs, Sluijs, Zeebe, Kump, Martindale, Greene, Kiessling, Ries, Zachos, Royer, Barker, Marchitto, Moyer, Pelejero, Ziveri, Foster and Williams2012; Trecalli et al. Reference Trecalli, Spangenberg, Adatte, Föllmi and Parente2012; Harazim et al. Reference Harazim, van de Schootbrugge, Sorichter, Fiebig, Weug, Suan and Oschmann2013; Hermoso & Pellenard, Reference Hermoso and Pellenard2014; Fantasia et al. Reference Fantasia, Föllmi, Adatte, Spangenberg and Montero-Serrano2018; Müller et al. Reference Müller, Jurikova, Gutjahr, Tomašových, Schlögl, Liebetrau, Duarte, Milovský, Mattioli and Pittet2020; Ruebsam et al. Reference Ruebsam, Reolid, Sabatino, Masetti and Schwark2020; Ullmann et al. Reference Ullmann, Boyle, Duarte, Hesselbo, Kasemann, Klein, Lenton, Piazza and Aberhan2020; Ettinger et al. Reference Ettinger, Larson, Kerans, Thibodeau, Hattori, Kacur and Martindale2021; Fernandez et al. Reference Fernandez, Korte, Ullmann, Looser, Wohlwend and Bernasconi2021). Warming may have been primarily responsible for the northward spread of Mediterranean ammonites during the tenuicostatum and serpentinum zones (e.g. Dera et al. Reference Dera, Neige, Dommergues and Brayard2011 b), also reflected by the presence of Tethyan gastropods and brachiopods in the Marlstone.
The coincident dramatic shift in oxygen isotopes, Mg:Ca ratios and 87Sr/86Sr values, most clearly seen in Block 1 and somewhat less obviously in Block 2 (Figs 5, 6), albeit likely being a function of condensation in the Marlstone and Junction Bed, is compatible with the view that the onset of hyperthermal conditions fostered an acceleration in continental weathering, thereby increasing the flux of radiogenic strontium to the oceans (Jenkyns, Reference Jenkyns2003, Reference Jenkyns2010; Ullmann et al. Reference Ullmann, Hesselbo and Korte2013 b). The initial warming itself is conventionally attributed to supply of carbon dioxide during eruption of the Karoo–Ferrar Large Igneous Province, an interpretation supported by the presence, in a number of sections, of mercury assumed to be of volcanic and/or thermogenic origin and transmitted through the atmosphere to be ultimately fixed in organic-rich sediments (Pálfy & Smith, Reference Pálfy and Smith2000; Svensen et al. Reference Svensen, Planke, Chevallier, Malthe-Sørenssen, Corfu and Jamtveit2007; Percival et al. Reference Percival, Witt, Mather, Hermoso, Jenkyns, Hesselbo, Al-Suwaidi, Storm, Xu and Ruhl2015, Reference Percival, Cohen, Davies, Dickson, Hesselbo, Jenkyns, Leng, Mather, Storm and Xu2016). Temperature-dependent dissociation of gas hydrates and/or metamorphism of organic-rich material, processes that would supply isotopically light carbon to the ocean–atmosphere system, have both been invoked as a prime cause of the negative carbon-isotope excursion that is one of the hallmarks of the T-OAE (Hesselbo et al. Reference Hesselbo, Gröcke, Jenkyns, Bjerrum, Farrimond, Morgans Bell and Green2000; McElwain et al. Reference McElwain, Wade-Murphy and Hesselbo2005).
Finally, it should be noted that belemnites are thought to have been nektobenthonic and likely record watermass characteristics below the thermocline that might have been ∼14 °C cooler than sea-surface temperatures, based on comparative oxygen-isotope and TEX86 data from Jurassic and Cretaceous sediments and their contained skeletal fossils (Mutterlose et al. Reference Mutterlose, Malkoc, Schouten, Sinninghe Damsté and Forster2010; Jenkyns et al. Reference Jenkyns, Schouten-Huibers, Schouten and Sinninghe Damsté2012). An exception to this rule may have been during the T-OAE when regionally developed expanded anoxic/euxinic bottom waters may have driven belemnites to occupy warmer nearer surface levels of the ocean (Ullmann et al. Reference Ullmann, Thibault, Ruhl, Hesselbo and Korte2014).
5. Conclusions
The stratigraphically condensed Marlstone and overlying Junction Bed (Pliensbachian–Toarcian) in coastal Dorset, UK (Wessex Basin) have yielded a range of chemostratigraphic data: 87Sr/86Sr, δ13C, δ18O and Mg:Ca of belemnites and δ13C and δ18O of bulk carbonate. Although the fossil-rich matrix of the Marlstone and Junction Bed shows a significant diagenetic overprint, the isotopic signals from the contained belemnites reflect global trends, such as increasing radiogenic 87Sr/86Sr values from a relative minimum around the Pliensbachian–Toarcian boundary, at which level there is evidence for a dramatic rise in temperature illustrated by δ18O and Mg:Ca of the fossil skeletal calcite. With regard to the T-OAE, there is no trace of organic-rich sediment anywhere in the Junction Bed, which could be due in part to the apparent absence of the lower exaratum subzone in the unit, which elsewhere in the UK is developed as laminated black shale. Alternatively, oxic conditions may have prevailed on the sea floor throughout the deposition of the Junction Bed. Possible signatures of the negative δ13C excursion that accompanies the T-OAE in sequences worldwide are present in the bulk carbon-isotope curve in the putative serpentinum zone, although they could also be interpreted as diagenetic artefacts. With its striking pink, yellow, red and brown colours, stromatolitic layers and enrichment in Fe–Mn oxyhydroxides, the Junction Bed and to a lesser extent the Marlstone recall certain highly condensed pelagic facies of the Tethyan Jurassic such as the Rosso Ammonitico and a similar depositional context is likely, namely on the relatively non-subsident sector of a complex half-graben system.
Supplementary material
To view supplementary material for this article, please visit https://doi.org/10.1017/S0016756821000972
Acknowledgements
Thanks are due to John Arden, Julie Cartlidge, Stephen Hesselbo, Stuart Robinson, Richard Woodfine, Steve Wyatt and two anonymous referees for their help with this paper. The study was supported by the Department of Earth Sciences, University of Oxford. Numerical data are given in the online Supplementary Material.