1. Introduction
Terminal Ediacaran and Cambrian successions worldwide record the consequences of profound geo-biological changes in continental configuration, global climate, biological evolution, sea level and oxygen concentrations of the atmosphere at that time (e.g. Knoll, Reference Knoll1991; Zhu et al. Reference Zhu, Zhang, Steiner and Yang2003; Fike et al. Reference Fike, Grotzinger, Pratt and Summons2006; Zhu, Strauss & Shields, Reference Zhu, Strauss and Shields2007; Guo et al. Reference Guo, Strauss, Liu, Zhao, Yang, Peng and Yang2010a , Reference Guo, Strauss, Liu, Zhao, Yang, Peng and Yang b , Reference Guo, Strauss, Zhu, Zhang, Yang, Lu and Zhao2013; Maloof et al. Reference Maloof, Susannah, Moore, Dudás, Samuel, Higgins, Fike and Eddy2010; Shields-Zhou & Och, Reference Shields-Zhou and Och2011; Jiang et al. Reference Jiang, Wang and Shi2012; Schrag et al. Reference Schrag, Higgins, Macdonald and Johnston2013; Feng et al. Reference Feng, Li, Huang, Chang and Chu2014; Wang et al. Reference Wang, Struck, Ling, Guo, Shields-Zhou, Zhu and Yao2015; Och et al. Reference Och, Cremonese, Shields-Zhou, Poulton, Struck, Ling, Li, Chen, Manning, Thirlwall, Strauss and Zhu2016). Numerous studies revealed the great chemostratigraphic potential of high-resolution carbon isotope records during the Precambrian–Cambrian transition (Brasier et al. Reference Brasier, Rozanov, Zhuravlev, Corfield and Derry1994; Kaufman & Knoll, Reference Kaufman and Knoll1995; Brasier & Sukhov, Reference Brasier and Sukhov1998; Saltzman et al. Reference Saltzman, Runnegar and Lohmann1998, Reference Saltzman, Ripperdan, Brasier, Lohmann, Robison, Chang, Peng, Ergaliev and Runnegar2000, Reference Saltzman, Runkel, Cowan, Runnegar, Stewart and Palmer2004; Montañez et al. Reference Montañez, Osleger, Banner, Mack and Masgrove2000), including many studies from the Yangtze Platform (Li et al. Reference Li, Chen, Zhang, Lei, Shen and Chu1999, Reference Li, Love, Lyons, Fike, Sessions and Chu2010, Reference Li, Ling, Shields-Zhou, Chen, Cremonese, Och, Thirlwall, Christina and Mannin2013; Shen & Schidlowski, Reference Shen and Schidlowski2000; Zhu et al. Reference Zhu, Zhang, Steiner and Yang2003, Reference Zhu, Zhang, Li and Yang2004; Guo et al. Reference Guo, Strauss, Liu, Goldberg, Zhu, Heubeck, Pi, Vernhet, Yang and Fu2007, Reference Guo, Strauss, Liu, Zhao, Yang, Peng and Yang2010a , Reference Guo, Strauss, Liu, Zhao, Yang, Peng and Yang b , Reference Guo, Strauss, Zhu, Zhang, Yang, Lu and Zhao2013, Reference Guo, Strauss, Zhao, Yang, Peng, Yang and Deng2014; G. Jiang et al. Reference Jiang, Kaufman, Christie-Blick, Zhang and Wu2007, Reference Jiang, Wang and Shi2012; Zhou & Xiao, Reference Zhou and Xiao2007; Zhu, Strauss & Shields, Reference Zhu, Strauss and Shields2007; Sawaki et al. Reference Sawaki, Ohno and Fukushi2008; Zhao & Zheng, Reference Zhao and Zheng2010). A relevant archive of sedimentary rocks from this time interval is well exposed in the Tarim Basin, China, including the preservation of different palaeoenvironmental settings. Similar sedimentary sequences between the Yangtze Platform and the Keping area of the Tarim Basin have been observed (Chen et al. Reference Chen, Sun, Liu and Zheng2004, Reference Chen, Jiang, Zhou, Yang and Han2010; Sun et al. Reference Sun, Chen, Zheng and Liu2004; Feng et al. Reference Feng, Bao and Wu2006; He, Xu & Yuan, Reference He, Xu and Yuan2007; Yu et al. Reference Yu, Dong, Widom, Chen and Lin2009).
The entire sedimentary succession comprises (in ascending stratigraphic order): limestone, dolostone, chert, black shale and phosphorite of the Ediacaran Qigebulage Formation and the lower Cambrian Yuertusi, Xiaoerbulak and Wusonger formations and the middle Cambrian Shayilik and Awatage formations. This sedimentary succession in the Tarim Basin is therefore well suited to uncover the interactions between atmosphere, hydrosphere, biosphere and lithosphere during this critical interval of Earth history. However, only a few geochemical studies (Chen et al. Reference Chen, Sun, Liu and Zheng2004, Reference Chen, Jiang, Zhou, Yang and Han2010; Sun et al. Reference Sun, Chen, Zheng and Liu2004; Feng et al. Reference Feng, Bao and Wu2006; He, Xu & Yuan, Reference He, Xu and Yuan2007; Yu et al. Reference Yu, Dong, Widom, Chen and Lin2009) have been focused on the stratigraphy and the geological events in the Tarim Basin during the Ediacaran–Cambrian and Cambrian Series 2 – Cambrian Series 3 transitions. These are insufficient for comparison with the Yangtze Platform and other geological successions of the world, especially the division and the correlations of the stratigraphy between the Ediacaran–Cambrian and Cambrian Stage 4 – Stage 5 boundaries.
This study focuses on sedimentary rocks across the Ediacaran – Cambrian Series 3 transition in the Tarim Basin, NW China, considering the Penglaiba, the Dongergou and the Wushi phosphorite sections in western Xinjiang in particular (Fig. 1). One of the major aims was to investigate the link between high-resolution carbon isotope variations and the correlation with sections in other regions and referred to transgression. Moreover, the data can be used for supporting/refining the chemostratigraphic subdivision of the Ediacaran – Cambrian Series 3 boundary in China and elsewhere.

Figure 1. Geological map of the Tarim Basin (modified from Feng et al. Reference Feng, Bao and Wu2006).
2. Geological setting and samples
The Tarim Basin is located in NW China, and contains sediments of the Ediacaran–Cambrian transition (Fig. 1). The Keping area in the northwestern part of the Tarim Basin provides good outcrop exposures from this time interval. During the Ediacaran–Cambrian transition, the Tarim Basin and the Yangtze platform were situated in a low-latitude position (Fig. 2) with similar depositional facies. This implies that the Tarim Basin is good for a regional stratigraphic division and correlation between both areas (Zhou Reference Zhou2001; Peng, Reference Peng2009; Peng, Babcock & Cooper, Reference Peng, Babcock, Cooper, Gradstein, Ogg, Schmitz and Ogg2012).

Figure 2. Palaeogeographic map during Ediacaran and Cambrian transition. (1) Lijiangtuo; (2) Wuliu-Zengjiayan; (3) Xiaotan; and (4) Penglaiba sections, China (modified from Scotese & McKerrow, Reference Scotese, McKerrow, McKerrow and Scotese1990; McKerrow, Scotese & Brasier, Reference McKerrow, Scotese and Brasier1992; Saltzman et al. Reference Saltzman, Ripperdan, Brasier, Lohmann, Robison, Chang, Peng, Ergaliev and Runnegar2000).
2.a. Penglaiba section
The Penglaiba section is located in Keping county, NW Xinjiang Uygur Autonomous Region. This section provides the most complete record including the Qigebulage, Yuertusi, Xiaoerbulak, Wusonger and Shayilik formations with no obvious depositional hiatus (Fig. 3). The Qigebulage Formation consists of carbonate and captures the transgressive Ediacaran–Cambrian boundary. The 20m thick sequence of the Yuertusi Formation consists of black phosphatic siliceous rocks, phosphorite, black shale and dolostone and contains benthic small shelly fossils (Chen et al. Reference Chen, Sun, Liu and Zheng2004, Reference Chen, Jiang, Zhou, Yang and Han2010; Sun et al. Reference Sun, Chen, Zheng and Liu2004; Feng et al. Reference Feng, Bao and Wu2006; He, Xu & Yuan, Reference He, Xu and Yuan2007; Yu et al. Reference Yu, Dong, Widom, Chen and Lin2009). The overlying 114m thick sequence of the Xiaoerbulak Formation consists of dolostone and intercalated limestone (Feng et al. Reference Feng, Bao and Wu2006). Remains of trilobites, brachiopods, ostracods and small shelly fossils are common. The overlying Wusonger Formation, an 87m thick sequence of dolostone and muddy dolostone (Feng et al. Reference Feng, Bao and Wu2006), is time-equivalent to the uppermost Stage 3 and Stage 4, Cambrian Series 2 (Fig. 3a; Zhou, Reference Zhou2001; Peng, Reference Peng2009). Trilobite species such as Paokannia sp. and Redlichia sp. from the Paokannia zone (Zhou, Reference Zhou2001) can be observed in the lower and middle part of the Wusonger Formation, which correlate to trilobite zones on the Yangtze Platform such as the Ovatoryctocara granulate–Bathynotus holopygus zone, the Arthricocephalus jiangkouensis, Arthricocephalus chauveaui and Arthricocephalites taijiangensis zones (Zhou, Reference Zhou2001; Peng, Reference Peng2009).

Figure 3. Comparison of temporal variations in δ13Ccarb, δ18Ocarb and TC for the (a) Penglaiba, (b) Wushi phosphrite and (c) Dongergou sections.
The 105m thick sequence of the Shayilik Formation captures the transgressive Stage 4 – Stage 5 boundary (Cambrian Series 2 – Series 3; Fig. 3a; Zhou, Reference Zhou2001; Peng, Reference Peng2009) and consists of dolostone, intercalated muddy dolostone and mudstone. The Kunmingaspis– Chittidilla trilobite zone (containing Chittidilla nanjiangensis Lu et Zhang, Kunmingaspis kalpingensis Zhang) of the Shayilik Formation in the Tarim Basin correlates with the Oryctocephalus indicus zone as well as the Peronopsis taijiangensis and Peychagnostus gibbus trilobite zones on the Yangtze Platform (Zhou Reference Zhou2001; Peng, Reference Peng2009).
Seventy-two (72) samples were collected from the Penglaiba section for geochemical work in this study.
2.b. Wushi phosphorite section
The Wushi phosphorite section is located in Wushi county, Xinjiang Uygur Autonomous Region, and comprises sedimentary rocks of (in ascending stratigraphic order) the Qigebulage, Yuertusi and Xiaoerbulak formations (Fig. 3b). The 165m thick Qigebulage Formation consists of dolostone and sandy dolostone, intercalated siltstone and sandstone, and there are abundant stromatolites, oncolites and micropalaeoflora in the formation. The overlying 24m thick sequence of the Yuertusi Formation consists of phosphatic siliceous rocks, phosphorite, black shale and limestone. The boundary of the Ediacaran and the Cambrian is located between the Qigebulage and Yuertusi formations. The 11m thick sequence of the Xiaoerbulak Formation consists of dolostone and siliceous dolostone. Fifty-nine (59) samples were collected from the Wushi phosphorite section for geochemical work in this study.
2.c. Dongergou section
The Dongergou section is located 60km SW of Akesu city, Xinjiang Uygur Autonomous Region, and comprises sedimentary rocks of the Yuertusi Formation (Fig. 3c). This 16.5m thick sequence consists of black phosphatic siliceous rocks, phosphorite, black shale and limestone with small shelly fossils (Anabarites–Protohertzina; Chen et al. Reference Chen, Sun, Liu and Zheng2004, Reference Chen, Jiang, Zhou, Yang and Han2010; Sun et al. Reference Sun, Chen, Zheng and Liu2004; Feng et al. Reference Feng, Bao and Wu2006; He, Xu & Yuan, Reference He, Xu and Yuan2007; Yu et al. Reference Yu, Dong, Widom, Chen and Lin2009). Fourteen (14) samples were collected from the Dongergou section for geochemical work in this study.
3. Analytical methods
Samples were chipped and pulverized (200 mesh). Total carbon (TC) abundances were measured at 1400°C using a high-frequency infrared carbon and sulphur analyser at the Institute of Geographic Sciences and Natural Resources Research, Chinese Academy of Sciences. CO2 for carbon and oxygen isotope analyses was liberated from whole-rock carbonates with phosphoric acid (McCrea, Reference McCrea1950; Wachter & Hayes, Reference Wachter and Hayes1985) at 50°C for 48 hours (dolostone) and 24 hours (limestone), respectively, and subsequent cryogenic distillation. All carbonate carbon and oxygen isotope analyses were carried out at the Institute of Geographic Sciences and Natural Resources Research, Chinese Academy of Sciences, using a ThermoFinnigan Delta Plus mass spectrometer. The analytical procedure was controlled by measuring the Beijing laboratory standard GBW 04406 for its δ18Ocarb (δ18Ocarb-standard: −12.40‰; Standard deviation: 0.20‰) and δ13Ccarb (δ13Ccarb-standard: −10.85‰; Standard deviation: 0.10‰) values. Results are reported in the standard delta notation as δ13C and δ18O v. VPDB. Standard deviations, as determined from replicate analyses, are generally better than 0.10 and 0.20‰ for carbon and oxygen isotopes, respectively.
In order to constrain carbonate diagenesis, samples were further studied for their elemental abundances of Mn, Sr, Fe, Ca and Mg (Veizer, Reference Veizer and Arthur1983; Popp et al. Reference Popp, Anderson and Sandberg1986; Kaufman et al. Reference Kaufman, Jacobsen and Knoll1993; Veizer et al. Reference Veizer, Bruckschen, Pawellek, Diener, Podlaha, Carden, Jasper, Korte, Strauss, Azmy and Ala1997, Reference Veizer, Ala, Azmy, Bruckschen, Buhl, Bruhn, Carden, Diener, Ebneth, Godderis, Jasper, Korte, Pawellek, Podlaha and Strauss1999). Samples were weighted and digested in 2N HCl and elemental concentrations were measured with atomic absorption spectroscopy at the Institute of Geographic Sciences and Natural Resources Research, Chinese Academy of Sciences. The precision is generally better than 5%. Results were corrected for the amount of insoluble residue (soluble (%) = (total weight – weight insoluble residue)/total weight).
4. Results
4.a. Penglaiba section
A total of 72 samples from the following lithologies were analysed on TC abundance and carbon isotopic composition (Fig. 3a; Table 1): carbonates from Qigebulage Formation, phosphorite and carbonates at the Yuertusi Formation, dolostones of the Xiaoerbulak and Wusonger formations and carbonates of the Shayilik Formation. TC abundances vary over the range 8.5–13wt% in the Qigebulage Formation, 0.4–13.2wt% in the Yuertusi Formation, 9.4–13.3wt% in the Xiaoerbulak Formation, 8.2–13.2wt% in the Wusonger Formation and 8.7–15.1wt% in the Shayilik Formation (Fig. 3a; Table 1).
Table 1. Analytical results for sediments from the Penglaiba section, Tarim Basin, NW China.
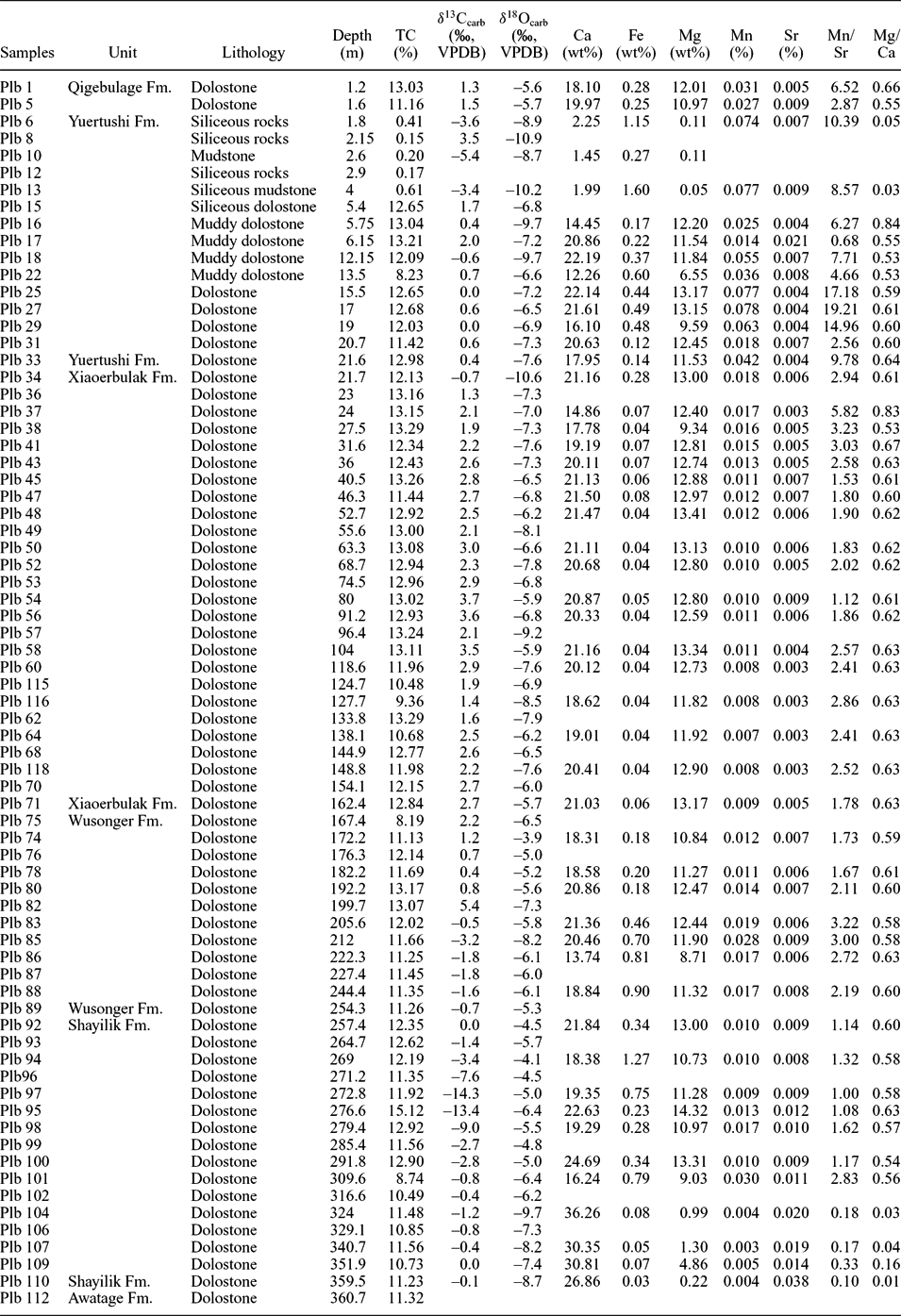
δ13Ccarb values for sediments range from –2.9‰ to 6.7‰ in the Qigebulage Formation, from –5.4‰ to 3.5‰ in the Yuertusi Formation, from –0.7‰ to 3.7‰ in the Xiaoerbulak Formation, from –3.2‰ to 5.4‰ in the Wusonger Formation and from 0‰ to –14.3‰ in the Shayilik Formation (Fig. 3a; Table 1).
Elemental abundances of Mn and Sr are highly variable (Mn 0.003–0.078%; Sr 0.003–0.039%). Analytical results are given in Table 1.
4.b. Wushi phosphorite section
The carbonate carbon isotope result is based on 59 samples (Fig. 3b; Table 2), including carbonates from the Qigebulage Formation (165m); across the Ediacaran–Cambrian transition; siliceous rocks, phosphorite and black shale at the base of Yuertusi Formation; and dolostones through the whole Yuertusi Formation.
Table 2. Analytical results for sediments from the Wushi phosphorite section, Tarim Basin, NW China.

Similar to the Penglaiba section, the base of the Yuertusi Formation in the Wushi phosphorite section consists of black phosphatic siliceous rocks, phosphorite and black shale (TC 0.3–1.8wt%), whereas the lithology of the rest of the section is carbonate (TC 7.6–13.2wt%).
δ13Ccarb values range between –2.9‰ and 6.7‰ in the Qigebulage Formation, and –9.8‰ and 1.1‰ in the Yuertusi Formation.
Elemental abundances of Mn and Sr are highly variable (Mn 0.003–0.141wt%; Sr 0.002–0.041wt%). Analytical results are given in Table 2.
4.c. Dongergou section
The Dongergou section comprises the Yuertusi Formation, which consists of black phosphatic siliceous rocks, phosphorite and black shale (TC 0.2–0.7wt%), whereas the lithology of the rest of the formation is carbonate (TC 5.3–13.2wt%).
Moreover, δ13Ccarb values vary from –7.7‰ to –0.1‰. Elemental abundances of Mn and Sr are highly variable (Mn 0.010–0.105%; Sr 0.002–0.319%). Analytical results are given in Table 3.
Table 3. Analytical results for sediments from the Dongergou section, Tarim Basin, NW China.

5. Carbonate diagenesis
Primary depositional trends from carbonate reflect seawater chemistry; however, carbonate diagenesis can change the elemental abundances and isotopic compositions of carbon and oxygen significantly (e.g. Veizer, Reference Veizer and Arthur1983; Marshall, Reference Marshall1992). Most frequently, an increase in the abundance of Mn, a decrease in the Sr concentration and a decrease in δ13Ccarb and δ18O (e.g. Veizer, Reference Veizer and Arthur1983; Marshall, Reference Marshall1992) reflect progressing diagenesis.
Usually, a Mn/Sr ratio <5 (even better is Mn/Sr ratio <2) and δ18O values more positive than –10‰ (even better is positive than –5‰) (Kaufman & Knoll, Reference Kaufman and Knoll1995) suggest that a carbonate has been retained near primary carbon isotope values and has archived past seawater chemistry.
No correlation can be observed between the carbonate carbon and oxygen isotopic compositions of the sedimentary rocks from the Yuertusi, Wusonger and Shayilik formations of the Penglaiba section, whereas a correlation is apparent between the carbonate carbon and oxygen isotopic compositions in the Xiaoerbulak Formation of the Penglaiba section (Fig. 4a). Moreover, a correlation is visible between the carbonate carbon and oxygen isotopic compositions of the sedimentary rocks from the Xiaoerbulak and Qigebulage formations of the Wushi phosphorite section (Fig. 4b), but no correlation could be observed between the carbonate carbon and oxygen isotopic compositions of the sedimentary rocks from the Yuertusi Formation of the Wushi phosphorite section.

Figure 4. Cross-plot of δ18Ocarb and δ13Ccarb for the (a) Penglaiba, (b) Wushi phosphrite and (c) Dongergou sections and (d) δ18Ocarb and Mn/Sr (weight ratio).
In contrast, an obvious correlation between the carbonate carbon and oxygen isotopic compositions of the carbonate from the Yuertusi Formation of the Dongergou section could be detected (Fig. 4c). Positive correlations between oxygen and carbon isotopes are probably a result of diagenesis or late alteration.
The Xiaoerbulak Formation of the Penglaiba section and the Wushi phosphorite section consist of dolostone. Dolomitization is believed to affect the carbonate δ18O value (Vasconcelos et al. Reference Vasconcelos, McKenzie, Warthmann and Bernasconi2005). Lower δ18O values, reflecting a greater degree of alteration, becomes less useful in the case of dolomitization which can lead to higher δ18O due to equilibrium isotopic fractionation (Li et al. Reference Li, Shields-Zhou, Ling and Thirlwall2011, Reference Li, Ling, Shields-Zhou, Chen, Cremonese, Och, Thirlwall, Christina and Mannin2013). Fluid–rock interaction shows the reset of δ18O values without any significant effect on the δ13C values (cf. Jacobsen & Kaufman, Reference Jacobsen and Kaufman1999), indicating that the δ13Ccarb values in dolostone reflect its near-primary composition although the δ18O values have been affected by fluid–rock interactions.
Mn/Sr and Mg/Ca ratios, as well as Mg, Fe, Mn, Sr and Ca abundances, are variable (Tables 1–3). No obvious correlation exists between Mn/Sr and the respective δ18O values (Fig. 4d).
A few samples indicate that carbonates have been altered during diagenesis (e.g. DRG 9 shows a relatively low δ18O value of –19.7‰, reflecting diagenetic alteration).
In summary, the absence of sufficient indication of post-depositional alteration in all three sections suggests that the carbonate carbon isotope records reflect near-primary values. This is discussed in the following section.
6. Discussion
6.a. Sections in the study
6.a.1. The Penglaiba section: variation in δ13Ccarb
At Penglaiba, a sedimentary succession comprising the terminal Ediacaran and most of the Cambrian is exposed (Yu et al. Reference Yu, Chen, Li and Lin2004, Reference Yu, Dong, Widom, Chen and Lin2009). The section represents a carbonate platform setting and provides the most complete carbonate carbon isotopic record of this study (Figs 3a, 5).

Figure 5. Stratigraphic variations of δ13Ccarb for the Penglaiba, Wushi phosphrite and Dongergou sections plotted against lithologic columns (①ED1; ②CAM1; ③CAM2; ④CAM3).
A shift in δ13Ccarb from 1.5‰ to a more negative value of –5.4‰ (②CAM1, Fig. 5) across the boundary between the Qigebulage Formation and the Yuertusi Formation is followed by rather invariable δ13Ccarb values between –0.6‰ and 2‰ in the middle and upper Yuertusi Formation. These in turn are followed by somewhat more variable and generally positive δ13Ccarb values between 1.3‰ and 3.7‰ in the Xiaoerbulak Formation, as well as more fluctuating δ13Ccarb values including a maximum value of about 5.4‰ in dolostones of the Wusonger Formation (③CAM2, Fig. 5).
The strongly negative δ13Ccarb excursion to values at –14.3‰ were observed near the base to the middle Shayilik Formation across the Cambrian Series 2 – Series 3 boundary (④CAM3, Fig. 5), followed by δ13Ccarb values around 0‰.
6.a.2. The Wushi phosphorite section
A positive δ13Ccarb excursion from 0‰ to 6.7‰ from the base to the upper Qigebulage Formation were observed (①ED1, Fig. 5), followed by a δ13Ccarb shift from 2.6‰ to –2.9‰ at the top of the Qigebulage Formation. The most negative δ13Ccarb value (–9.8‰) in the section occurs at the base of the Yuertusi Formation (②CAM1, Fig. 5). Moreover, a δ13Ccarb shift from –9.8‰ to 1.9‰ from the middle Yuertusi Formation to the base of the Xiaoerbulak Formation (③CAM2, Fig. 5) is apparent.
6.a.3. The Dongergou section
The carbonate carbon isotope record is based on 14 samples (Fig. 3c; Table 3). Similar to the results for the Penglaiba and the Wushi phosphorite sections, the most negative δ13Ccarb value of the Dongergou section with –7.7‰ is recorded at the base of the Yuertusi Formation (②CAM1, Fig. 5) followed by a δ13Ccarb shift towards –0.2‰ between the middle Yuertusi Formation and the base of the Xiaoerbulak Formation.
6.b. Comparison of the stratigraphy in the Tarim Basin
An overall increase in δ13Ccarb from 3‰ to 6.7‰ at the base of Qigebulage Formation is followed by a slight negative shift to nearly invariable values at the middle to the upper Qigebulage Formation (①ED1, Fig. 5) and a negative shift at the upper part of Qigebulage Formation. Further upsection, δ13Ccarb records a shift to minimum values of –9.8‰ in the Wushi phosphorite section to –7.7‰ in the Dongergou section and to –5.4‰ in the Penglaiba section. A shift to positive carbonate carbon isotope values occurs across the Yuertusi Formation in the middle of Xiaoerbulak Formation, followed by nearly invariable positive carbonate carbon isotope values between 1.4‰ and 3.7‰ for dolostones in the middle and upper Xiaoerbulak Formation (③CAM2, Fig. 5). Variable carbonate carbon isotope values are recorded for the Wusonger Formation. The most negative δ13Ccarb value occurs at the base of the Shayilik Formation (–14.3‰, Penglaiba section) (④CAM3, Fig. 5). The observation is probably as a result of the upwelling of water, with dissolved inorganic carbon carrying a 13C depleted signature due to recycling of organic matter or enhanced weathering of organic-carbon-bearing rocks on the continents, and subsequent delivery of 13C depleted dissolved inorganic carbon into the ocean; alternatively, it may be related to the enrichment of organic matter, which could be responsible for the observed change in δ13C (Guo et al. Reference Guo, Strauss, Liu, Zhao, Yang, Peng and Yang2010a , Reference Guo, Strauss, Liu, Zhao, Yang, Peng and Yang b ). A positive carbonate carbon isotope excursion occurs in the middle–upper Shayilik Formation, indicating the recovery of the environment, increasing organic carbon burial and increasing carbon fixation.
6.c. Comparison between different continents
The Tarim Basin in NW China contains a sedimentary succession of Ediacaran and Cambrian rocks, including the deposition in different palaeoenvironmental settings (inner shelf, outer shelf, slope and basin). Three sections in the Keping area represent the carbonate platform environments. In the Tarim Basin, the Keping area exhibits a sedimentary succession and fossils comparable to the carbonate platform of the Yangtze Platform during the Ediacaran–Cambrian period (Chen et al. Reference Chen, Sun, Liu and Zheng2004, Reference Chen, Jiang, Zhou, Yang and Han2010; Sun et al. Reference Sun, Chen, Zheng and Liu2004; Feng et al. Reference Feng, Bao and Wu2006; He, Xu & Yuan, Reference He, Xu and Yuan2007; Yu et al. Reference Yu, Dong, Widom, Chen and Lin2009). The stratigraphic variation of the total carbon abundances shows a parallel evolution between the lower part of the Yuertusi Formation and the Niutitang Formation. All results from the study match previously published carbonate carbon isotope data from other sections on the Yangtze Platform (e.g. Zhang et al. Reference Zhang, Li, Zhou, Zhu and Yu1997; Zhu et al. Reference Zhu, Zhang, Li and Yang2004; Zhu, Babcock & Peng, Reference Zhu, Babcock and Peng2006; Guo et al. Reference Guo, Strauss, Liu, Goldberg, Zhu, Heubeck, Pi, Vernhet, Yang and Fu2007, Reference Guo, Strauss, Liu, Zhao, Yang, Peng and Yang2010a , Reference Guo, Strauss, Liu, Zhao, Yang, Peng and Yang b , Reference Guo, Strauss, Zhu, Zhang, Yang, Lu and Zhao2013; G. Jiang et al. Reference Jiang, Kaufman, Christie-Blick, Zhang and Wu2007, Reference Jiang, Wang and Shi2012; Yang et al. Reference Yang, Zhu, Guo and Zhao2007; Zhu, Strauss & Shields, Reference Zhu, Strauss and Shields2007; Li et al. Reference Li, Ling, Shields-Zhou, Chen, Cremonese, Och, Thirlwall, Christina and Mannin2013). The change in the carbon isotopic composition in the sequence of early Cambrian black rocks from the Yangtze Platform and the Tarim Basin is based on a large-scale transgressive event and interpreted as a change from anoxic to possibly dysoxic bottom-water conditions (S. Jiang et al. Reference Jiang, Yang, Ling, Chen, Feng, Zhao and Ni2007; Guo et al. Reference Guo, Strauss, Zhu, Zhang, Yang, Lu and Zhao2013).
Although defining the Ediacaran–Cambrian succession by δ13Ccarb data in these deeper water sections is problematic, respective δ13Corg and δ13Ccarb data allow stratigraphic comparison of some sections of the Yangtze Platform (Zhang et al. Reference Zhang, Li, Zhou, Zhu and Yu1997; Guo et al. Reference Guo, Strauss, Liu, Goldberg, Zhu, Heubeck, Pi, Vernhet, Yang and Fu2007, Reference Guo, Strauss, Zhu, Zhang, Yang, Lu and Zhao2013; S. Jiang et al. Reference Jiang, Yang, Ling, Chen, Feng, Zhao and Ni2007; Yang et al. Reference Yang, Zhu, Guo and Zhao2007; G. Jiang et al. Reference Jiang, Wang and Shi2012; Peng, Babcock & Cooper, Reference Peng, Babcock, Cooper, Gradstein, Ogg, Schmitz and Ogg2012; Li et al. Reference Li, Ling, Shields-Zhou, Chen, Cremonese, Och, Thirlwall, Christina and Mannin2013) and also the Tarim Basin (Fig. 6).

Figure 6. Comparison of temporal variations in δ13Ccarb from the different sections during the transition from the Ediacaran and Cambrian of (1) the Yangtze Platform, China: Shatan (Guo et al. Reference Guo, Strauss, Liu, Goldberg, Zhu, Heubeck, Pi, Vernhet, Yang and Fu2007), Songtao (Guo et al. Reference Guo, Strauss, Liu, Goldberg, Zhu, Heubeck, Pi, Vernhet, Yang and Fu2007), Yanwutan-Lijiatuo (Guo et al. Reference Guo, Strauss, Liu, Goldberg, Zhu, Heubeck, Pi, Vernhet, Yang and Fu2007), Longbizui (Guo et al. Reference Guo, Strauss, Zhu, Zhang, Yang, Lu and Zhao2013), Yuanjia (Guo et al. Reference Guo, Strauss, Zhu, Zhang, Yang, Lu and Zhao2013), Xiaotan (Li et al. Reference Li, Ling, Shields-Zhou, Chen, Cremonese, Och, Thirlwall, Christina and Mannin2013), Wuliu–Zengjiayan (Guo et al. 2010), Jianshan (Guo et al. Reference Guo, Strauss, Liu, Zhao, Yang, Peng and Yang2010a , b) and Wangcun sections (Zhu et al. Reference Zhu, Zhang, Li and Yang2004); (2) the Tarim Basin, China: Penglaiba (this study), Wushi phosphrite (this study) and Dongergou sections (this study); (3) USA: Rocky Mountain section (Montañez et al. Reference Montañez, Osleger, Banner, Mack and Masgrove2000); (4) Russia: Molodo section (Shabanov et al. Reference Shabanov, Korovnikov, Pereladov, Pak and Feflov2008); and (5) Canada: Sekwi Formation (Dilliard et al. Reference Dilliard, Pope, Coniglio, Hasiotis and Lieberman2007).
A negative shift in δ13Ccarb (negative excursion at the base of the Cambrian System (BASE); Zhu, Babcock & Peng, Reference Zhu, Babcock and Peng2006) from the base to the top of Qigebulage Formation and across the Ediacaran–Cambrian transition into the Yuertusi Formation can be observed. Moreover, more negative δ13Ccarb values can be observed at the base of the Yuertusi Formation in the Keping area, which can be compared with carbon isotope values from the transition of the Dengying Formation (Liuchapo Formation) and Niutitang Formation on the Yangtze Platform (Zhang et al. Reference Zhang, Li, Zhou, Zhu and Yu1997; Zhu et al. Reference Zhu, Zhang, Li and Yang2004; Zhu, Babcock & Peng, Reference Zhu, Babcock and Peng2006; Zhu, Strauss & Shields, Reference Zhu, Strauss and Shields2007; Guo et al. Reference Guo, Strauss, Liu, Goldberg, Zhu, Heubeck, Pi, Vernhet, Yang and Fu2007, Reference Guo, Strauss, Zhu, Zhang, Yang, Lu and Zhao2013; G. Jiang et al. Reference Jiang, Kaufman, Christie-Blick, Zhang and Wu2007, Reference Jiang, Wang and Shi2012; Yang et al. Reference Yang, Zhu, Guo and Zhao2007; Li et al. Reference Li, Ling, Shields-Zhou, Chen, Cremonese, Och, Thirlwall, Christina and Mannin2013; Fig. 6). The δ13C minimum reflects a global decrease in organic carbon burial and/or a decrease in carbon fixation, probably caused by a transgressive event (e.g. S. Jiang et al. Reference Jiang, Yang, Ling, Chen, Feng, Zhao and Ni2007; Guo et al. Reference Guo, Strauss, Zhu, Zhang, Yang, Lu and Zhao2013), flooding the shelf area with 13C depleted basinal anoxic bottom water. The Ediacaran–Cambrian boundary in India (Kaufman et al. Reference Kaufman, Jiang, Christie-Blick, Banerjee and Rai2006), Iran (Brasier et al. Reference Brasier, Magaritz and Corfeld1990), Siberia (e.g. Magaritz et al. Reference Magaritz, Kirschvink, Latham, Zhuravlev and Rozanov1991; Brasier, Khomentovsky & Corfield, Reference Brasier, Khomentovsky and Corfield1993; Brasier et al. Reference Brasier, Rozanov, Zhuravlev, Corfield and Derry1994, Reference Brasier and Sukhov1998; Knoll et al. Reference Knoll, Grotzinger, Kaufman and Kolosov1995a , Reference Knoll, Kaufman, Semikhatov, Grotzinger and Adams b ; Kouchinsky et al. Reference Kouchinsky, Bengtson, Pavlov, Runnegar, Torssander, Young and Ziegler2007), Mongolia (Brasier et al. Reference Brasier, Shields, Kuleshov and Zhegallo1996; Khomentovsky & Gibsher, Reference Khomentovsky and Gibsher1996; Maloof et al. Reference Maloof, Susannah, Moore, Dudás, Samuel, Higgins, Fike and Eddy2010), Morocco (Maloof et al. Reference Maloof, Schrag, Crowley and Bowring2005, Reference Maloof, Susannah, Moore, Dudás, Samuel, Higgins, Fike and Eddy2010), Oman (Fike et al. Reference Fike, Grotzinger, Pratt and Summons2006; Schröder & Grotzinger, Reference Schröder and Grotzinger2007), the Yangtze Platform (e.g. Shen & Schidlowski, Reference Shen and Schidlowski2000; Zhu, Strauss & Shields, Reference Zhu, Strauss and Shields2007; Guo et al. Reference Guo, Strauss, Liu, Goldberg, Zhu, Heubeck, Pi, Vernhet, Yang and Fu2007, Reference Guo, Strauss, Zhu, Zhang, Yang, Lu and Zhao2013; S. Jiang et al. Reference Jiang, Yang, Ling, Chen, Feng, Zhao and Ni2007; G. Jiang et al. Reference Jiang, Wang and Shi2012; Peng, Babcock & Cooper, Reference Peng, Babcock, Cooper, Gradstein, Ogg, Schmitz and Ogg2012; Li et al. Reference Li, Ling, Shields-Zhou, Chen, Cremonese, Och, Thirlwall, Christina and Mannin2013) and the Tarim Basin (this study) can be correlated with each other using the negative carbon isotope anomaly followed by a transition to less negative δ13C values in lower Cambrian stratigraphy. A widespread transgressive event can be detected for lower Cambrian rocks on different continents (e.g. S. Jiang et al. Reference Jiang, Yang, Ling, Chen, Feng, Zhao and Ni2007; Guo et al. Reference Guo, Strauss, Zhu, Zhang, Yang, Lu and Zhao2013).
A distinct positive δ13Ccarb excursion from the Yuertusi Formation to the Xiaoerbulak Formation of the Penglaiba section reflects the enhanced fractional burial of organic matter and the release of oxygen. This excursion can be compared with the positive δ13Ccarb shift in the lower part of Cambrian Stage 2 (positive excursion in the lower part of Stage 2 (ZHUCE); Zhu, Babcock & Peng, Reference Zhu, Babcock and Peng2006), which is the equivalent to the Dahai Member of the Xiaotan section in the shelf area of NE Yunnan, at 62m of the Longbizui section and at 33m at the Yuanjia section of the slope to basin area in the Yangtze Platform (Fig. 6; Zhu, Babcock & Peng, Reference Zhu, Babcock and Peng2006; Li et al. Reference Li, Ling, Shields-Zhou, Chen, Cremonese, Och, Thirlwall, Christina and Mannin2013; Guo et al. Reference Guo, Strauss, Zhu, Zhang, Yang, Lu and Zhao2013). Such a stratigraphic correlation is strongly supported by similar phosphorus-rich sediments between the ZHUCE-equivalent interval of the Penglaiba section, the Longbizui/Yuanjia sections and the Xiaotan section (Figs 2, 6).
A linear evolution towards more 13C-depleted carbonate carbon isotope values can be observed across the boundary of the Wusonger Formation and the Shayilik Formation at the Penglaiba section, with the most negative δ13Ccarb value of –14.3‰. This can be compared with the evolution of the carbonate carbon isotopes on the Yangtze Platform. The Cambrian Series 2 – Series 3 transition is characterized worldwide by a negative carbon isotope excursion caused by a transgressive event and biological radiation (Redlichiid–Olenellid extinction carbon isotope excursion or ROECE; Zhu, Babcock & Peng, Reference Zhu, Babcock and Peng2006). The samples from the Penglaiba section show the most 13C-depleted signature among Cambrian Series 2 and Cambrian Series 3, which could reflect a closer proximity to the source of 13C-depleted water during upwelling (Guo et al. Reference Guo, Strauss, Liu, Zhao, Yang, Peng and Yang2010a , Reference Guo, Strauss, Liu, Zhao, Yang, Peng and Yang b ). Above the deposits of the transgressive event, the δ13Ccarb values increase again to values of c. 0‰, indicating the recovery in the marine environment, which is in accordance with values recorded for the Wuliu–Zengjiayan section and the Wangcun section on the Yangtze Platform (Zhu et al. Reference Zhu, Zhang, Li and Yang2004; Guo et al. Reference Guo, Strauss, Liu, Zhao, Yang, Peng and Yang2010a , Reference Guo, Strauss, Liu, Zhao, Yang, Peng and Yang b ). More evidence (Guo et al. Reference Guo, Strauss, Liu, Zhao, Yang, Peng and Yang2010a , Reference Guo, Strauss, Liu, Zhao, Yang, Peng and Yang b ) for this development is provided by the fact that the position of the observed negative shift in δ13C can be correlated with a similar chemostratigraphic evolution in the sections of the Tarim Basin (this study) by a composite profile across the boundary from the Yangtze Platform (Zhu et al. Reference Zhu, Zhang, Li and Yang2004; Guo et al. Reference Guo, Strauss, Liu, Zhao, Yang, Peng and Yang2010a , Reference Guo, Strauss, Liu, Zhao, Yang, Peng and Yang b ), Canada (Dilliard et al. Reference Dilliard, Pope, Coniglio, Hasiotis and Lieberman2007), the USA (Montañez et al. Reference Montañez, Osleger, Banner, Mack and Masgrove2000) and in respective successions in Siberia (Shabanov et al. Reference Shabanov, Korovnikov, Pereladov, Pak and Feflov2008) (Fig. 6). The development of inhospitable bottom-water conditions due to the introduction of anoxic waters would undoubtedly result in faunal extinction (Guo et al. Reference Guo, Strauss, Liu, Zhao, Yang, Peng and Yang2010a , Reference Guo, Strauss, Liu, Zhao, Yang, Peng and Yang b ). The negative carbon isotope excursion and the trilobite mass extinction (e.g. Montañez et al. Reference Montañez, Osleger, Banner, Mack and Masgrove2000) at the ROECE event in the uppermost part of Cambrian Series 2 and the lowermost part of Cambrian Series 3 were caused by major palaeogeographical changes coupled to the transgressive event (Guo et al. Reference Guo, Strauss, Liu, Zhao, Yang, Peng and Yang2010a , Reference Guo, Strauss, Liu, Zhao, Yang, Peng and Yang b ), which resulted in the deposition of transgressive successions visible in the Gondwana and Laurentia sections (e.g. Landing & Bartowski, Reference Landing and Bartowski1996; Brasier & Sukhov, Reference Brasier and Sukhov1998; Montañez et al. Reference Montañez, Osleger, Banner, Mack and Masgrove2000; Zhu et al. Reference Zhu, Zhang, Li and Yang2004; Wotte et al. Reference Wotte, Álvaro, Shields, Brown, Brasier and Veizer2007; Guo et al. Reference Guo, Strauss, Liu, Zhao, Yang, Peng and Yang2010a , Reference Guo, Strauss, Liu, Zhao, Yang, Peng and Yang b ; Figs 2, 6).
The carbon isotopic evolution recorded for the sections in the Tarim Basin is in good agreement with existing δ13C records obtained from other sections on the Yangtze Platform that define the ZHUCE and the ROECE events (Zhu et al. Reference Zhu, Zhang, Li and Yang2004; Zhu, Babcock & Peng, Reference Zhu, Babcock and Peng2006; Zhu, Strauss & Shields, Reference Zhu, Strauss and Shields2007).
7. Conclusion
Complete high-resolution carbonate carbon isotope profiles across the Ediacaran–Cambrian and Cambrian Series 2 – Series 3 transitions in the Keping area of the Tarim Basin in NW China display clear stratigraphic variations. These can be correlated with the carbonate carbon isotope records for sedimentary successions on the Yangtze Platform. Isotope excursions reflect changes in the fractional burial of organic carbon, biological evolution and geological events. δ13Ccarb variations in the successions of the Tarim Basin and the Yangtze Platform correlate with each other and define the BASE, ZHUCE and the ROECE events. However, the global signature has been enhanced by a regional signal, likely as a consequence of differences in the palaeogeographical setting. These results reflect the perturbation of the carbon cycle in the Tarim Basin during the Ediacaran–Cambrian and the Cambrian Series 2 – Series 3 transitions.
Acknowledgements
The authors appreciate the assistance and expertise in the field as well as stimulating discussions with Professor Harald Strauss, Dr Marc Peters, Professor Bingsong Yu, Professor Xianhua Li, Professor Maoyan Zhu, Dr Chukwunonso Peter Okoli and Mr Yue Chen. GQ acknowledges financial support from 973 Program (no. 2013CB835004) and the National Natural Science Foundation of China (NSFC nos 41473018, 41625006 and 41173008).