1. Introduction
The Canary Islands are a group of ocean island volcanoes situated near the continental margin of northwest Africa. Tenerife is the largest and topographically highest island in the Canaries, with a base area of > 2000 km2 and a summit elevation of 3718 m (Pico de Teide). The oldest volcanic rocks exposed on Tenerife are the remnants of the basalt shield massifs of Roque del Conde (S Tenerife), Anaga (NE Tenerife), and Teno (NW Tenerife; Fig. 1a), with a constructional age between 11.9 Ma and 3.9 Ma (Guillou et al. Reference Guillou, Carracedo, Paris and Pérèz Torrado2004a). Fuster et al. (Reference Fuster, Araña, Brandle, Navarro, Alonso and Aparicio1968) combined the volcanic rocks of these massifs into the Old Basaltic Series.
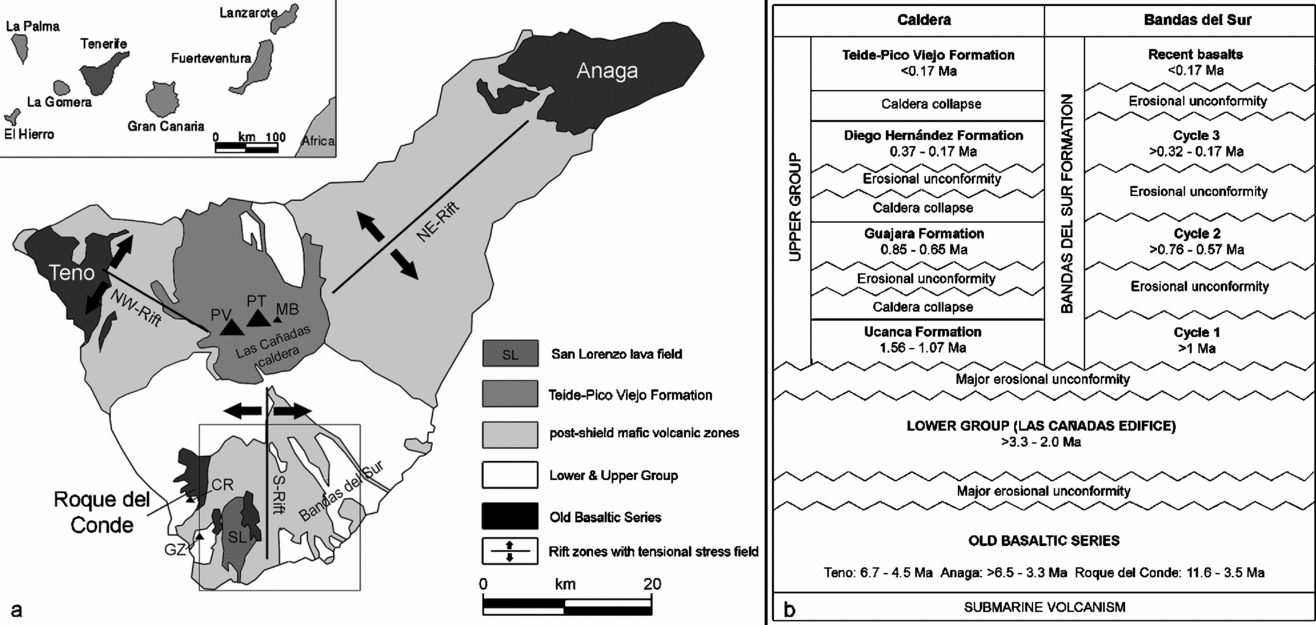
Figure 1. (a) Simplified geological map of Tenerife island, showing the main volcanic features; modified after Ablay & Martí (Reference Ablay and Martí2000), Carracedo (Reference Carracedo1994) and Carracedo et al. (Reference Carracedo, Rodríguez Badiola, Guillou, Paterne, Scaillet, Perez Torrado, Paris, Fra-Paleo and Hansen2007). Frame marks the study area (presented in detail in Fig. 2). CR – Caldera del Rey; GZ – Montaña Guaza; MB – Montaña Blanca; PT – Pico de Teide; PV – Pico Viejo; SL – San Lorenzo lava field. (b) Volcanic stratigraphy of Tenerife; modified after Bryan, Martí & Cas (Reference Bryan, Martí and Cas1998).
The Las Cañadas volcano in the central part of Tenerife started to grow more than 3 Ma ago (Fuster et al. Reference Fuster, Ibarrola, Snelling, Cantagrel, Huertas, Coello and Ancochea1994). The formations of the Las Cañadas volcano were divided into a Lower and an Upper Group (Fig. 1b) by Marti, Mitjavila & Araña (Reference Martí, Mitjavila and Araña1994). The Lower Group was erupted between > 3 Ma (Fuster et al. Reference Fuster, Ibarrola, Snelling, Cantagrel, Huertas, Coello and Ancochea1994) and 2 Ma (Marti, Mitjavila & Araña, Reference Martí, Mitjavila and Araña1994) and comprises basaltic, phonolitic and trachytic lavas and subordinate pyroclastic rocks (Ancochea et al. Reference Ancochea, Fuster, Ibarrola, Cendero, Coello, Hernan, Cantagrel and Jamond1990). The volcanic rocks of the Upper Group are dominated by pyroclastic deposits that have been erupted during cyclic explosive volcanic activity (Marti, Mitjavila & Araña, Reference Martí, Mitjavila and Araña1994).
The summit region of the Las Cañadas volcano is characterized by a large depression, known as the Las Cañadas caldera. The origin of this depression is still the subject of controversy; gravitational collapses resulting in giant landslides, vertical collapses caused by emptying of shallow magma chambers or a combination of vertical and lateral collapses are the most plausible processes (for detailed discussion, see Brown et al. Reference Brown, Barry, Branney, Pringle and Bryan2003; Carracedo et al. Reference Carracedo, Rodríguez Badiola, Guillou, Paterne, Scaillet, Perez Torrado, Paris, Fra-Paleo and Hansen2007; Edgar et al. Reference Edgar, Wolff, Olin, Nichols, Pittari, Cas, Reiners, Spell and Martí2007). Inside the Las Cañadas caldera, the stratovolcanoes of Pico de Teide and Pico Viejo post-date the caldera forming Upper Group cycles, and have a constructional age of > 150 ka (Araña, Barberi & Ferrara, Reference Araña, Barberi, Ferrara, Callao and Araña1989).
Marti, Mitjavila & Araña (Reference Martí, Mitjavila and Araña1994) subdivided the Upper Group into three explosive volcanic cycles: the Ucanca Formation (1.59–1.18 Ma), the Guajara Formation (0.85–0.65 Ma) and the Diego Hernández Formation (0.37–0.17 Ma). The Bandas del Sur Formation comprises more than seven widespread ignimbrite sheets erupted from the Las Cañadas edifice (Brown et al. Reference Brown, Barry, Branney, Pringle and Bryan2003) during two explosive volcanic cycles (Cycle 2: > 0.76–0.57 Ma and Cycle 3: > 0.32–0.17 Ma; Bryan, Martí & Cas (Reference Bryan, Martí and Cas1998); Fig. 1b). Each cycle is inferred to have started with flank eruptions of alkali basalt lava and the growth of cinder cones. Three widely eroded cinder cones, cropping out in the east of the Bandas del Sur (Fig. 1a), are all the remains of the basaltic eruptions at the initial phase of Cycle 2 (Bryan, Martí & Cas, Reference Bryan, Martí and Cas1998). The Cycle 3 basalts (Series III basalts of Fuster et al. Reference Fuster, Araña, Brandle, Navarro, Alonso and Aparicio1968) are widely distributed within the Bandas del Sur. The youngest episode of flank eruptions within the Bandas del Sur resulted in the lavas and cinder cones of the San Lorenzo lava field (the Recent basalts sensu Bryan, Martí & Cas, Reference Bryan, Martí and Cas1998). These are equivalent to the Recent Series of Marti, Mitjavila & Araña (Reference Martí, Mitjavila and Araña1994) and Series IV (Series Recientes IV e Historica) of Fuster et al. (Reference Fuster, Araña, Brandle, Navarro, Alonso and Aparicio1968). Each cycle evolved from initial basaltic to phonolitic eruptions (Bryan, Martí & Leosson, Reference Bryan, Martí and Leosson2002). The aim of this study is to determine the stratigraphic position and age distribution of the cinder cones in the Bandas del Sur, using magnetic stratigraphy, geochemistry and K–Ar ages.
2. Methods
2.a. Palaeomagnetism
Palaeomagnetic studies were carried out on 44 cinder cones within the Bandas del Sur, using a modified version of the fluxgate magnetometer ‘Fluxmaster’ (Stefan-Mayer Instruments). Field measurements using a portable magnetometer have been successfully carried out in the Canary Islands (e.g. Carracedo, Reference Carracedo1979; Guillou et al. Reference Guillou, Carracedo, Paris and Pérèz Torrado2004a, Reference Guillou, Perez Torrado, Hansen Machin, Carracedo and Gimenob; Paris et al. Reference Paris, Guillou, Carracedo and Perez Torrado2006). The magnetization of 85% of the samples measured during field work has been identical with samples measured in laboratory (Guillou et al. Reference Guillou, Perez Torrado, Hansen Machin, Carracedo and Gimeno2004b). In a study by Doell & Cox (Reference Doell and Cox1962), the error margin of the samples measured in the field was about 5% for volcanic rocks. Accordingly, a minimum of six measurements were carried out for each cinder cone, using orientated samples of the cores from large in situ volcanic bombs and/or samples of lava flows issued from the cones. The samples were collected over the entire extent of each cone, in order to obtain statistically viable results and to avoid local polarity reversals caused by lightning. For readability, the following terms are used: ‘R-Basalts’ for cones and lavas with reverse remanent magnetization, ‘N-Basalts’ for cones with normal remanent magnetization (except the younger cones of the San Lorenzo lava field) and ‘N-Basalts-SL’ for all cinder cones of the San Lorenzo lava field, owing to the fact that these cones invariably show normal remanent magnetization.
2.b. Geochemistry
Major and trace element concentrations have been determined for 16 whole-rock samples at the Institute of Mineralogy, University of Stuttgart, and at the IFM-GEOMAR, Kiel, by XRF methods (Table 1). After crushing they were pulverized using an automatic tungsten carbide mill. The analyses were carried out using a Rh-tube, calibrated by international geological standards. The CO2 and H2O concentrations were dissected by infrared photometry after the pulverized samples were heated up to 1000 °C.
Table 1. Major and trace element data for the cinder cones in the study area and the syenite from Montaña Pelada

2.c. K–Ar age dating
For the K–Ar age determination, unweathered samples were used. The age determinations were carried out for two samples at the Laboratoire des Sciences du Climat et l'Environment (France). The materials used were whole rock samples, crushed to particle sizes varying between 0.250 and 0.125 mm, and ultrasonically washed in HC2H3O2. The converted ages were calculated using the following constants: 40K/K = 1.167 10−4 g/g; λɛ = 0.572 10−10/a; λ'ɛ = 0.0088 10−10/a; λβ = 4.962 10−10/a. The error margin for the age is 2σ (Appendix Table A1).
3. New data
3.a. Palaeomagnetic data
The cinder cones in the study area show both normal and reverse remanent magnetization (Fig. 2, Table 2, and Appendix Table A2). Thirteen cinder cones exhibit reverse polarities, 27 normal polarities, and four cinder cones do not show any clear palaeomagnetic signal (Fig. 2), due to either the lack of adequate volcanic bombs or to demagnetization induced by lightning (Graham, Reference Graham1961). The locations (UTM-Coordinates) and sample denominations are listed in Table 2. According to the geomagnetic polarity time scale (GPTS), the last geomagnetic polarity reversal event (Matuyama/Brunhes) was dated at 778.7 ± 1.9 ka ago (Singer & Pringle, Reference Singer and Pringle1996). Accordingly, at least 13 cinder cones in the study area must be considered to be older than 778.7 ka (Figs 2, 3a–d). The other cones, displaying normal polarity, erupted during the Brunhes (> 778.7 ka).

Figure 2. Position and magnetization of cinder cones in the study area. Light type: known K–Ar ages are given from Carracedo et al. (Reference Carracedo, Rodríguez Badiola, Guillou, Paterne, Scaillet, Perez Torrado, Paris, Fra-Paleo and Hansen2007); bold type: new K–Ar ages presented in this study.
Table 2. UTM-coordinates, magnetization and trace element ratios for the cinder cones in the study area; XRF data are recalculated to anhydrous conditions

N: normal polarity; R: reverse polarity.

Figure 3. (a) General overview of the distribution of cinder cones within the Bandas del Sur, southern Tenerife; a chain of cinder cones orientated along the trend of the S-Rift can be recognized in the centre of the photo. (b) Intensively eroded cinder cone of Montaña Ifaro with gently inclined flanks that are partly covered by ignimbrites (constructional age of c. 950 ka, Cycle 2). (c) Slightly eroded cinder cone of Montaña Gorda with steep flanks (constructional age of c. 300 ka, Cycle 3). (d) Largely unweathered cinder cone of Buzanada with steep flanks and fresh scoria (constructional age of c. 100 ka, Cycle 4).
3.b. Geochemical data
The geochemical data, in particular the immobile trace elements, were used as an additional tool to distinguish between the R-Basalts, N-Basalts and N-Basalts-SL. The results of the analyses for major and trace elements are presented in Table 1. Geochemically, the samples show an alkalic trend, plotting in the basanite, tephrite and trachybasalt field of the Total Alkali–Silica diagram (Le Bas et al. Reference Le Bas, Le Maitre, Streckeisen and Zanettin1986). One sample plots in the field for basaltic trachyandesites (Fig. 4a). The SiO2 contents range from 41.7 wt% to 47.5 wt% for N-Basalts and from 42.4 wt% to 52.1 wt% for R-Basalts, indicating that during the eruption of the N- and R-Basalts, the magma evolved from a primitive basanitic to a more differentiated melt (Fig. 4a).

Figure 4. (a) Classification of the R-Basalts (reverse polarity), N-Basalts (normal polarity), N-Basalts-SL (normal polarity, San Lorenzo lava field) and syenite (tephriphonolite; TF-P1) using the TAS-diagram (Le Bas et al. Reference Le Bas, Le Maitre, Streckeisen and Zanettin1986). (b) Multi-element plot for the R-Basalts, N-Basalts and the syenite (tephriphonolite), normalized to primordial mantle (Sun, Reference Sun1980; McDonough et al. Reference McDonough, Sun, Ringwood, Jagoutz and Hofman1992). The MORB-line is taken from Saunders & Tarney (Reference Saunders, Tarney, Kokelaar and Howells1984) and Sun (Reference Sun1980).
Multi-element plots for several trace elements (Fig. 4b), normalized to primary mantle composition (Sun, Reference Sun1980; McDonough et al. Reference McDonough, Sun, Ringwood, Jagoutz and Hofman1992), show enrichment in elements ranging from Rb to Ti as compared to N-MORB element distribution (Saunders & Tarney, Reference Saunders, Tarney, Kokelaar and Howells1984; Sun, Reference Sun1980). Potassium is depleted relative to Ba, Th and Nb. The R- and N-Basalts and N-Basalts-SL exhibit chemical signatures typical for ocean island basalts and Canary Island basalts enriched in the more incompatible elements and a negative K anomaly (Ancochea & Huertas, Reference Ancochea and Huertas2003), similar to those of HIMU-type basalts (Weaver Reference Weaver1991). The N-, R-Basalts and N-Basalts-SL show comparable chemical signatures, indicating that they all were derived from the same mantle source (Fig. 4b). The content of lithophile elements like Na2O, K2O, Rb and Nb increases with decreasing MgO content (Fig. 5). The depletion of CaO, Fe2O3 and V with decreasing MgO is indicative of fractionation processes involving the formation of olivine, titanoferous magnetite and clinopyroxene (Price & Chappell, Reference Price and Chappell1975). The N-Basalts are generally more enriched in Na2O, K2O and in the incompatible elements at similar MgO concentrations compared to the R-Basalts and N-Basalts-SL (Fig. 5). This may indicate that the N-Basalts were generated as a result of lower degrees of partial melting. The trace element plot Y/Nb versus Zr/Nb separates the R-Basalts, the N-Basalts and the N-Basalts-SL into three well-defined fields (Fig. 6). The N-Basalts exhibit Y/Nb ratios <0.35, the R-Basalts show Y/Nb ratios between 0.37 and 0.41 and the N-Basalts-SL display Y/Nb ratios ~0.47 (Table 2). Additionally, this plot is useful to evaluate the degree of enrichment of the mantle source (Pearce & Norry, Reference Pearce and Norry1979; Abratis, Schmincke & Hansteen, Reference Abratis, Schmincke and Hansteen2002); hence, the N-Basalts are derived from a more enriched mantle source compared to R-Basalts and N-Basalts-SL (see Fig. 6).

Figure 5. Incompatible and compatible elements plotted against MgO for the samples studied in this work.

Figure 6. Trace element variations between the R-Basalts, N-Basalts and N-Basalts-SL showing different mantle source characteristics; modified after Pearce & Norry (Reference Pearce and Norry1979). EMS – enriched mantle source; DMS – depleted mantle source.
3.c. K–Ar age determination
Age determination resulted in an age of 300 ± 8 ka for the sample TF-4/Amarilla (N-Basalts) and 948 ± 15 ka for the sample TF-47/Roja (R-Basalts) (Table 2). Carracedo et al. (Reference Carracedo, Paterne, Guillou, Pérez Torrado and Paris2003, Reference Carracedo, Rodríguez Badiola, Guillou, Paterne, Scaillet, Perez Torrado, Paris, Fra-Paleo and Hansen2007) presented K–Ar ages of 95 ± 5 ka for a cinder cone near La Buzanada, 323 ± 6 ka for the cinder cone Montaña Gorda, and 311 ± 6 ka for a basaltic lava flow at the Autopista Sur km 30. The cinder cone near La Buzanada comprises N-Basalts-SL, while Montaña Gorda comprises N-Basalts (Fig. 2, Table 2). Based on these dates, a minimum age for the R-Basalts is between the last geomagnetic polarity reversal event (Matuyama/Brunhes) at 778.7 ± 1.9 ka (Singer & Pringle, Reference Singer and Pringle1996) and the K–Ar age of 948 ± 15 ka (TF-47/Roja). The eruption of N-Basalts started at a minimum age between 323 ± 6 ka and 300 ± 8 ka. Volcanic activity of the San Lorenzo lava field began c. 95 ± 5 ka ago (Fig. 7).

Figure 7. Volcanic cycles documented in the Bandas del Sur and related volcanic deposits. Data sources: Fuster et al. (Reference Fuster, Ibarrola, Snelling, Cantagrel, Huertas, Coello and Ancochea1994); Bryan, Martí & Cas (Reference Bryan, Martí and Cas1998); Bryan, Martí & Leosson (Reference Bryan, Martí and Leosson2002); Huertas et al. (Reference Huertas, Arnaud, Ancochea, Cantagrel and Fúster2002); Brown et al. (Reference Brown, Barry, Branney, Pringle and Bryan2003); Carracedo et al. (Reference Carracedo, Paterne, Guillou, Pérez Torrado and Paris2003, Reference Carracedo, Rodríguez Badiola, Guillou, Paterne, Scaillet, Perez Torrado, Paris, Fra-Paleo and Hansen2007) and Edgar et al. (Reference Edgar, Wolff, Olin, Nichols, Pittari, Cas, Reiners, Spell and Martí2007). For volcanic deposits that are not absolutely dated, the relative age is defined by their stratigraphic position.
4. Characteristics of the S-Rift
Compared to the NW- and NE-Rift, the evolution of the southern rift zone proceeded unequally in many respects. The NW- and NE-Rift zones display steep ridges built up by fissure eruptions and are characterized by a narrow chain of cinder cones and an extensional stress field, triggering flank collapses (e.g. Icod, Orotava, Güimar: Carracedo et al. Reference Carracedo, Rodríguez Badiola, Guillou, Paterne, Scaillet, Perez Torrado, Paris, Fra-Paleo and Hansen2007). Eruptive activity along the NE- and NW-Rift located in medial and distal zones of the Las Cañadas caldera mainly produced mafic lavas; felsic eruptions exclusively occurred in proximal zones near or within the caldera (Carracedo et al. Reference Carracedo, Rodríguez Badiola, Guillou, Paterne, Scaillet, Perez Torrado, Paris, Fra-Paleo and Hansen2007).
In the S-Rift the volcanic features are arranged in widely scattered clusters and parallel chains following the direction of the S-Rift (Fig. 2); it does not display a distinct ridge and instead has a fan-shaped distribution of the monogenetic cones (fig. 1 in Carracedo et al. Reference Carracedo, Rodríguez Badiola, Guillou, Paterne, Scaillet, Perez Torrado, Paris, Fra-Paleo and Hansen2007). In the distal part of the southern rift zone, basaltic cinder cones occur together with two phonolitic eruption centres (Montaña Guaza and Caldera del Rey). According to Martí, Andujar & Wolff (Reference Martí, Andujar and Wolff2004), the phonolitic centres (see Figs 1, 8a) represent the only manifestations of highly evolved magma outside the Las Cañadas caldera. In the tuff cone deposits of Montaña Pelada, syenitic components (‘tephriphonolite’ TF-P1 in Fig. 4a, b) occur as ballistic blocks ranging from a few centimetres to about 1 m in size (Fig. 8b). The syenitic components of intermediate character (Fig. 4) are composed of K-feldspar, plagioclase and feldspathoides (mainly nepheline) (Fig. 8c). On Tenerife, syenitic rock fragments have been described exclusively as components of ignimbrites (e.g. El Abrigo ignimbrite: Wolff, Grandy & Larson, Reference Wolff, Grandy and Larson2000) that were derived from the Las Cañadas edifice; the tuff cone deposits of Montaña Pelada, however, do not display any components derived from reworked ignimbrites. Wolff (Reference Wolff1987) proposed that the Tenerife syenitic components crystallized on the roof of a phonolitic magma chamber.

Figure 8. (a) View northward to the phonolitic dome and flow complex of Montaña Guaza; phonolitic lava flow (behind the housing) is tens of metres thick; the phonolitic lava dome of Montaña Guaza is situated centre-right of the photograph. (b) Syenitic (tephriphonolitic) nodule as ballistic block in the tuff cone deposits of Montaña Pelada east of El Médano. (c) Thin-section micrograph of the syenitic component (Montaña Pelada) showing hypidiomorphic K-feldspar and plagioclase crystals.
5. Discussion
5.a. S-Rift zone
According to Walter & Troll (Reference Walter and Troll2003), the triaxial architecture of the rift zones on Tenerife is characteristic of a volcano that is centrally supplied with magma and is experiencing increases in the instability of a sector. This results in two rift zones with intense volcanic activity (corresponding with the NW- and NE-Rift zone on Tenerife) and a less pronounced rift zone, located in the stable part of the volcano (corresponding with the S-Rift zone on Tenerife). Acosta et al. (Reference Acosta, Uchupi, Smith, Muñoz, Herranz, Palomo, Lianes and Ballesteros2003) described rift characteristics of the nearby islands of La Palma and El Hierro that are comparable to the S-Rift of Tenerife. These are characterized by a broad fan-like distribution of monogenetic centres. They relate this to the stress field within the rift being insufficient to trap dykes within a narrow region and/or to dyke injection and volcanism shifting laterally through time. Additionally, a low rate of magma supply produces low magma pressures, responsible for randomly orientated dyke injections. The rift zones of the Hawaiian Islands generally consist of linear and steep ridges. The toes of some Hawaiian rifts partly fan out as well, perhaps as a result of low magma supply (Acosta et al. Reference Acosta, Uchupi, Smith, Muñoz, Herranz, Palomo, Lianes and Ballesteros2003).
In the distal part of the S-rift zone, basaltic cinder cones are associated with volcanic features formed by phonolitic eruptions (Fig. 9a, b). Additionally, syenitic components (TF-P1, Fig. 4) occur as ballistic blocks in the tuff cone of Montaña Pelada. Widom, Gill & Schmincke (Reference Widom, Gill and Schmincke1993) interpreted syenitic ballistic blocks at the Agua de Pao Volcano on the island of San Miguel (Azores) as fragments that had crystallized in the margins of a magma chamber ejected during an explosive eruption. Together with the phonolitic volcanic centres of Montaña Guaza and Caldera del Rey, we interpret the occurrence of syenitic rocks as evidence for shallow magma pockets, located in the distal part of the S-Rift (compare to Fig. 9b).

Figure 9. (a) Model of the triaxial rift zone on Tenerife illustrating the distribution of cinder cones; narrow chains of cinder cones on top of the steep ridges of the NW- and NE-Rift and a fan-like distribution of monogenetic volcanic structures within the S-Rift. (b) Interpretation of the occurrence of phonolitic volcanic structures in the distal part of the S-Rift with feeder dykes and assumed magma pockets underneath the Bandas del Sur. CR – Caldera del Rey; GZ – Montaña Guaza; PL – Montaña Pelada.
5.b. Cyclicity of volcanic activity
The major pyroclastic deposits in the Bandas del Sur correlate with eruptions that built up the major ignimbrite layers of the Ucanca, Guajara and Diego Hernández Formation cropping out in the caldera wall. According to Martí, Mitjavila & Araña (Reference Martí, Mitjavila and Araña1994) and Bryan, Martí & Cas (Reference Bryan, Martí and Cas1998), these formations represent three volcanic cycles: each cycle started with mafic flank eruptions and culminated in an explosive, caldera-forming eruption, collectively responsible for the development of the Las Cañadas caldera (Martí et al. Reference Martí, Hurlimann, Ablay and Gudmundsson1997). According to Brown et al. (Reference Brown, Barry, Branney, Pringle and Bryan2003) and Edgar et al. (Reference Edgar, Wolff, Olin, Nichols, Pittari, Cas, Reiners, Spell and Martí2007), numerous ignimbrite layers and pumice fall deposits of the Bandas del Sur give evidence for more than three caldera collapse events and a more complex development of the Las Cañadas caldera. On the other hand, the three cycles, represented by major pyroclastic deposits within the Bandas del Sur, are separated from each other by longer periods without significant volcanic activity, erosional disconformities and palaeosoils which are discordantly overlain by basaltic lava flows. In the aftermath of periods of non-pyroclastic deposition, ascending magma in the Las Cañadas volcano probably has been responsible for increasing flank eruptive activity in the southern rift zone, producing large lava fields and cinder cones.
Volcanic eruption cycles for the Santorini volcano (Greece) with an average duration of about 180 ka have been described by Druitt et al. (Reference Druitt, Mellors, Pyle and Sparks1989), Druitt et al. (Reference Druitt, Edwards, Mellors, Pyle, Sparks, Lanphere, Davies and Barreiro1999) and Zellmer, Turner & Hawkesworth (Reference Zellmer, Turner and Hawkesworth2000); each cycle ended with a major caldera-forming eruption. According to Christiansen (Reference Christiansen2001), the volcanic activity of the Yellowstone caldera volcano (Wyoming, USA) is characterized by three volcanic cycles during the last 2.1 Ma; each cycle totals about 640 ka to 800 ka. The early stage has been dominated by plateau basalt eruptions and each cycle ended with a major caldera forming eruption. However, basaltic as well as rhyolitic eruptions accompanied the entire period of volcanic activity. The time span of major volcanic activity of the three cycles ranges from about 340 ka to 580 ka, and the repose periods between the cycles from about 140 ka to 520 ka, respectively (Christiansen, Reference Christiansen2001).
5.c. Stratigraphy
Based on the dataset presented in this work and on the radiometric age dating of several volcanic units in previous literature, we define four volcanic cycles based on the stratigraphy developed by Bryan, Martí & Cas (Reference Bryan, Martí and Cas1998), Brown et al. (Reference Brown, Barry, Branney, Pringle and Bryan2003) and Edgar et al. (Reference Edgar, Wolff, Olin, Nichols, Pittari, Cas, Reiners, Spell and Martí2007) for the Bandas del Sur (Fig. 7). The volcanic cycles 1 to 4 depicted in Figure 7 are defined for the volcanic succession of the Bandas del Sur Group; in the NW- and NE-Rift, due to the lack of ignimbrites, a volcanic cyclicity has not yet been identified. According to Galindo & Soriano (Reference Galindo and Soriano2005), fissure eruptions along the NE-Rift have occurred since the early development of the rift up to the historical eruptions. Cycle 1 deposits are scarce in the Bandas del Sur and Cycle 1 is poorly defined in age and distribution. Pyroclastic deposits of Cycle 1 are preserved in the southwestern flank of Tenerife; however, the stratigraphic position of these units is not well defined. Additionally, neither the Helecho Member nor the Arafo Member of the Bandas del Sur Group have been dated yet. Further dating of pyroclastic deposits and lavas is necessary to constrain better the duration of each cycle. Cinder cones belonging to Cycle 1 have not yet been found in the study area.
The alignments of Cycle 2, 3 and 4 cinder cones occur mainly along NNE–SSW trends (Fig. 2), representing the main direction of the S-Rift zone (Figs 1a, 8a). The specific alignment indicates that persistent fractures acted as continuous channels for the mafic flank eruptions within the Bandas del Sur (Bryan, Martí & Cas, Reference Bryan, Martí and Cas1998; Bryan, Martí & Leosson, Reference Bryan, Martí and Leosson2002). In previous literature, all the cinder cones within the study area (except the San Lorenzo cinder cones) were assigned to the Cycle 3/Series III-basalts (e.g. Fuster et al. Reference Fuster, Araña, Brandle, Navarro, Alonso and Aparicio1968; Bryan, Martí & Cas, Reference Bryan, Martí and Cas1998; Bryan, Martí & Leosson, Reference Bryan, Martí and Leosson2002; Clarke et al. Reference Clarke, Troll, Carracedo, Byrne and Gould2005) with an eruption age between 690 ka and the Upper Pleistocene/Holocene boundary (e.g. Bellido Mulas et al. Reference Bellido Mulas, Brandle Matesanz, Hernández-Pacheco and Fernández Santín1978; Hernández-Pacheco & Fernández Santín, Reference Hernández-Pacheco and Fernández Santín1978; Carracedo, Reference Carracedo1979) or > 320–316 ka (Bryan, Martí & Cas, Reference Bryan, Martí and Cas1998).
The palaeomagnetic data and K–Ar ages determined through this study indicate that a specific number of cinder cones belong to an older volcanic cycle. At least 13 cinder cones show reverse magnetization (R-Basalts; Fig. 2) and hence they must be older than the last geomagnetic polarity reversal event at 778.7 ± 1.9 ka (Singer & Pringle, Reference Singer and Pringle1996). The cones represent the initial stage of Cycle 2 eruptions. Bryan, Martí & Cas (Reference Bryan, Martí and Cas1998) set the beginning of Cycle 2 to a minimum age of > 760 ka. Using the age of TF-47/Roja (948 ± 15 ka) and the palaeomagnetic data, the start of flank eruptive activity at the base of Cycle 2 can be set at between > 948 ± 15 ka and 778.7 ± 1.9 ka (Fig. 7).
The basalts showing normal magnetization (N-Basalts and N-Basalts-SL; Fig. 2) belong to Cycle 3 and to the San Lorenzo lava field. The assumed onset of Cycle 3 > 320 ka ago (Bryan, Martí & Cas, Reference Bryan, Martí and Cas1998) correlates with the age of the cinder cone Montaña Gorda and with a lava flow by Carracedo et al. (Reference Carracedo, Paterne, Guillou, Pérez Torrado and Paris2003, Reference Carracedo, Rodríguez Badiola, Guillou, Paterne, Scaillet, Perez Torrado, Paris, Fra-Paleo and Hansen2007), as well as with the age of TF-4/Amarilla, presented in this study. It can be assumed that the majority of basaltic flank eruptions at the base of Cycle 3 took place between 323 ± 6 ka and 300 ± 8 ka.
The basaltic eruptions of the San Lorenzo lava field indicate the start of a possible further volcanic cycle (Kröchert, Maurer & Buchner, Reference Kröchert, Maurer and Buchner2008). The age of the San Lorenzo lava field is given from the K–Ar age of a cinder cone near La Buzanada (Carracedo et al. Reference Carracedo, Rodríguez Badiola, Guillou, Paterne, Scaillet, Perez Torrado, Paris, Fra-Paleo and Hansen2007), defining the onset of Cycle 4 to a minimum age of > 95 ka. The caldera-forming eruptions of Cycle 3 have been followed by the construction of the Pico de Teide and Pico Viejo stratocones that began with non- or minor-explosive eruptions of basaltic to intermediate and phonolitic lavas inside the Las Cañadas caldera; basaltic fissure eruptions occurred concurrently along the NW- and NE-Rift (Carracedo et al. Reference Carracedo, Rodríguez Badiola, Guillou, Paterne, Scaillet, Perez Torrado, Paris, Fra-Paleo and Hansen2007). The subplinian eruption of Montaña Blanca (Fig. 1) about 2 ka ago (Ablay et al. Reference Ablay, Ernst, Martí and Sparks1995) may represent a change from effusive basaltic flank eruptions and non-explosive volcanism at Pico de Teide and Pico Viejo to a more explosive phase (Bryan, Martí & Cas, Reference Bryan, Martí and Cas1998; Wolff, Grandy & Larson, Reference Wolff, Grandy and Larson2000), similar to the earlier volcanic cycles (Fig. 7).
The ends of the four cycles defined in this study are characterized by widespread erosional unconformities in the Bandas del Sur, by thick palaeosoil development (Bryan, Martí & Cas, Reference Bryan, Martí and Cas1998) and by extended periods without explosive volcanic eruptions. The gap between Cycles 1 and 2 lasted for about 180 ka, between Cycles 2 and 3 for about 250 ka, and between 3 and 4 for about 70 ka. The period of volcanic activity of Cycle 2 lasted for about 350 ka and of Cycle 3 for 180 ka (compare with Fig. 7).
5.d. Geochemistry
The geochemical data reveal a magmatic evolution from primitive magma (SiO2 41.7 wt%) to a more evolved tephritic and trachybasaltic magma for the R-Basalts and N-Basalts, due to fractional crystallization processes involving the removal of olivine, clinopyroxene and titaniferous magnetite (Figs 4, 5). The N-Basalts-SL, with high MgO, Fe2O3, V and Cr abundances represent a primitive magma with relatively short storage periods. Rapid ascent of magma from the mantle to upper crustal levels was proposed by Neumann, Wulff-Pedersen & Simonsen (Reference Neumann, Wulff-Pedersen and Simonsen1999), based on the presence of ultramafic xenoliths in the cinder cones and dykes of Tenerife. The range in the trace element abundances of the R-Basalts and N-Basalts (Fig. 4) could be explained by fractional crystallization processes, assimilation or different degrees of partial melting of the mantle source. Lower alkali (Na2O+K2O), Rb and Nb contents of the R-Basalts at similar MgO content in comparison to the N-Basalts and N-Basalts-SL (Fig. 5) may suggest a higher degree of partial melting. Immobile trace element plots for Y/Nb versus Zr/Nb (Pearce & Norry, Reference Pearce and Norry1979) show that the basalts plot into three different fields. The N-Basalts with Y/Nb ratios <0.35 indicate that they were derived from a more enriched mantle source compared to the R-Basalts (Y/Nb: 0.37–0.41) and the N-Basalts-SL (Y/Nb: ~0.47); however, these ratios could also be influenced by magma mixing and mingling processes, characteristic for magma compositions on Tenerife (Ablay et al. Reference Ablay, Carrol, Palmer, Martí and Sparks1998; Bryan, Martí & Leosson, Reference Bryan, Martí and Leosson2002; Bryan, Reference Bryan2006). Fractional crystallization in periodically refilled magma chambers and the assimilation of syenite and amphibolite are also processes, present on Tenerife, which can change element ratios/abundances (Neumann, Wulff-Pedersen & Simonsen, Reference Neumann, Wulff-Pedersen and Simonsen1999; Simonsen, Neumann & Seim, Reference Simonsen, Neumann and Seim2000) and may overprint primary mantle source characteristics. However, the Y/Nb versus Zr/Nb plot combined with the palaeomagnetic data appears to be a good tool to determine the stratigraphic position of cinder cones within the Bandas del Sur.
6. Conclusions
(1) The S-Rift zone has characteristics (morphological features, geochemistry of the volcanic deposits) that are different from those of the NW- and NE-Rift zones. It does not display a distinct ridge and has a fan-shaped distribution of monogenetic cones. Together with phonolitic volcanic structures, we interpret the occurrence of syenitic rocks as evidence for shallow magma pockets, located in the distal part of the S-Rift.
(2) We identified four volcanic cycles in the volcanic succession of the Bandas del Sur; cycles 1 to 3 commenced with intense mafic flank eruptions and culminated in a series of explosive phonolitic eruptions. However, the formation of phonolitic volcanic structures outside the caldera as well as caldera forming eruptions occurred over a longer period and are not necessarily restricted to the final stage of each cycle.
(3) We define the end of each cycle by the occurrence of erosional unconformities, palaeosoils and longer periods without volcanic activity. The gap between Cycle 1 and 2 lasted for about 180 ka, between Cycle 2 and 3 for about 250 ka, and between 3 and 4 for about 70 ka.
(4) The cinder cones within the Bandas del Sur can be split into three different age groups, belonging to the Cycle 2, 3 and 4 episodes of basaltic flank volcanism.
(5) The Cycle 2 cinder cones have a wide distribution in the Bandas del Sur Formation and were erupted between > 948 ± 15 ka and 778.7 ± 1.9 ka.
(6) The main eruptive activity of the Cycle 3 basalts occurred between 323 ± 6 ka and 300 ± 8 ka.
(7) The basaltic eruptions which formed the San Lorenzo lava field abut 95 ka ago may define the start of a fourth volcanic cycle.
Acknowledgements
We kindly thank Dr Steffen Kutterolf for helping us to improve the quality of our manuscript. We would also like to thank Dr Holger Maurer and Martin Schmieder for helpful comments and Anita Czambor for sample preparation and assistance during analytical work. We are grateful to the following institutions for financial support: Stifterverband für die Deutsche Wissenschaft and Graduiertenförderung des Landes Baden-Württemberg. Our London colleague Hilary Randall, who proofread the manuscript, is kindly acknowledged. We are grateful to the two reviewers, Prof. Dr Juan Carlos Carracedo and Dr Richard James Brown, for detailed and helpful comments on our manuscript.