Introduction
Biomacromolecules are biomolecules which have a bulky size of 800–1000 Daltons, having complex structure and high molecular weight. These are biological polymers of various simple or monomer units, that is, lipids, carbohydrates, proteins and nucleic Acids (Refs Reference Zhang, Sun and Jiang1–Reference Hanna3). They have immersed increased attention as carriers in biomedicine in current years due to their innate biophysical and biochemical properties including biocompatibility, biodegradability, non-toxicity, renewability, targeting ability and high blood circulation time. Recently, advancements in the field of biomacromolecules show that synthetic carrier replaced by biological-based carriers has improved output because of stable half-life, functional stability and manufacturing feasibility (Ref. Reference Zhang, Sun and Jiang1).
The burden of cancer continues to rise globally and puts immense physical, emotional and financial pressure on people, families, societies and health care systems (Refs Reference Hanna3, Reference Kanwal, Ding and Cao4). Many health systems in low- and middle-income countries are least equipped and ill-prepared to cope with this burden (Ref. Reference FAO2). Across the globe, significant numbers of cancer patients are unable to access quality diagnosis and treatment on time and thus leading to an increase in death (Ref. Reference Hanna3). In contrast, due to affordable early detection, survival care and quality treatment, survival rates are increasing in countries with strong health services. Globally, the leading cause of mortality is cancer that accounts for probable 9.6 million deaths in 2018. Pulmonary cancer is still considered as the foremost leading cause of death in both genders globally with almost 2.08 million cases and 1.73 million casualties in 2018 (Ref. 5).
Air pollution and smoking are the main causes of lung cancer; yet, frequent studies have confirmed that genetic factors also add to the development of lung cancer (Ref. Reference Kanwal, Ding and Cao4). Many synthetic and environmental factors dragged lung cancer to be the leading cause of mortality (Refs Reference Manchado6, Reference Shimomura7). Second-hand smoke such as cigarettes and hookah whose smoke is composed of radioisotopes, benzopyrene and nitrosamine are the basic factors contributing towards lung cancer. Air pollution is full of fine particles and aerosols as well ejected by cars and channels from factories that have worse consequences. In addition to it, asbestos is a toxin and causes mesothelioma (cancer of pleural membrane of lungs) (Ref. Reference Sheu8) (Fig. 1).

Fig. 1. Risk factors to lung cancer and global statistics. The morbidity of lung cancer is almost similar in both men and women; however, the incidence is greater in men (14.5%) as compared to women (8.4%) across the globe. Smoking is the primary risk factor for lung cancer that signifies 85% deaths associated with lung cancer. This risk increases with the quantity of smoked cigarettes/day plus years of smoking. In addition, non-smokers are more exposed to lung cancer as compared to smokers. Air pollution containing carcinogens upon inhalation contributes towards pulmonary cancer. Airborne fibre contains broken shards of asbestos that are carcinogenic and lead to lung cancer (3–4%). Radon is a radioactive gas obtained from radium-226 decay; the isotope produces α particles that lead to cell damage and ultimately lung cancer. Radon is considered as a second leading cause of pulmonary cancer.
The post-transcriptional silencing gene with RNAi is a promising novel approach to gene therapy. One feature of cancer is an abnormally large number of oncogenes (Refs Reference Manchado6, Reference Sheu8). SiRNA has the ability to target any gene associated with cancer and pose a new form of cancer therapy. In recent years, the engineering of siRNA carriers has gained considerable interest, that is, development of efficient delivery systems capable of transmitting siRNA to tumour tissue and tumour cell cytoplasm. RNA interferences are fundamental pathways triggered by double-strand ribonucleic acids. An endonuclease dicer acts on this double-strand RNA and cleaves it into fragments (Refs Reference Postmus9, Reference Chen10). siRNA gets loaded into RISC–RNA induced silencing complex. This complex is composed of a protein called Argonaut-2 which has the capability of cutting and detaching the passenger strand of siRNA duplex. Then via complementary base pairing, the argonaute-guided RNA specifies and recognises the target messenger RNA. Finally, the protein (argonaute) destroys the target mRNA exist complementary to the anti-sense strand, and between bases 10, 11 relative to the 5` end of the antisense strand, an endo-nucleolytic cleave occurs that eventually leads to gene silencing (Ref. Reference Usmani11).
In the battle against various crucial diseases, an effective carrier system for significant delivery therapeutic cargoes is a major challenge. In recent years, miscellaneous drug carriers for the treatment and diagnosis of diseases have been developed, that is, magnetic nanoparticles, micelle, liposomes, hydrogel, microcapsule, microspheres, etc. (Refs Reference McKelvey12–Reference Durymanov and Reineke19). Minimal cargo loading, immature drug release (hydrophobic drugs), high cost, non-biodegradability and poor stability are some of the obvious shortcomings associated with liposomes and nanomaterials. All these problems make them inappropriate as a drug delivery carrier (Refs Reference Dammes and Peer20, Reference Pei and Buyanova21). Therefore, biomacromolecules of natural origin perform a complex range of functions in their native environment. Polysaccharides act as a storage sites and mean of intracellular communication within the membranes. Similarly, proteins act as a catalyst, transport vehicles and structural materials. Due to such a diverse and inspiring role of biomacromolecules, scientists started to utilise them as a delivery tool (Refs Reference Dominska and Dykxhoorn22–Reference Large24). Biomacromolecule-based carriers for the delivery of various cargos are reported as a pro-drug, nanoparticle, drug conjugates, hydrogel, etc. Using bio-macromolecular-based scaffolds as a carrier poses an improved pharmacokinetic profile and reduced systemic toxicity followed by immunogenicity of the targeted payloads. In addition to it, the existence of special groups on these biomacromolecules, pose them to chemical modification and thus make them a versatile class of agents in biomedical field (Refs Reference Hanna3–Reference Sheu8, Reference Postmus9, Reference Chen10, Reference Usmani11–Reference Lee36).
This review highlights the various barriers/challenges in the path of siRNA-biomacromolecules cargo to lungs with special emphasis on strategies to overcome such barriers, paving the way for achieving maximum therapeutic outcomes.
An overview of lung cancers
Lung cancer basically has two categorisations based on therapy, biology and prognosis, that is, SCLC (small-cell lung carcinoma) and NSCLC (non-small-cell lung carcinoma). SCLC is also termed as oat cell carcinoma – a very dangerous tumour causing almost 15% of lung cancers and is biologically, pathologically, molecularly and clinically very dissimilar from other lung cancers. This form of lung cancer is characterised by rapid proliferation of tumours, early metastatic spread and initial therapy responsiveness. Constricted-stage cancer, potentially curable, is treated with chemotherapy and radiation, with surgical treatment reserved for selected patients with stage I cancer (Ref. Reference Gazdar, Bunn and Minna25). The most common (85%) and probable type of lung cancer is NSCLC. People who smoke or who breathe a lot of smoke are most likely to get NSCLC. Non-small-cell lung carcinoma is further divided into squamous cell carcinoma and non-squamous cell carcinoma (adenocarcinoma, large-cell carcinoma) (Ref. Reference Postmus9).
NSCLC patients require major staging workup to evaluate the extent of the disease, even though stage plays a significant role in determining treatment choice.
Conventional treatment of lung cancer
Its treatments depend upon the requirement and condition of the patient; however, radiation therapy, chemotherapy and surgery are the available treatment for pulmonary cancer (Ref. Reference Chen10). On the other hand, in the metastatic stage (advance stage) of pulmonary cancer (i.e. extensive peak stage for small-cell lung carcinoma and stage 4 for non-small-cell lung carcinoma), chemotherapy has many drawbacks that limit the lungs therapy (Ref. Reference Sadhasivam and Sugumaran26). The main concerned problem with chemotherapy is utilizing platinum-based entities and drugs such as cisplatin and carboplatin which are dangerous having many adverse effects, that is, weakness, fatigue, hair loss, neuropathy, nephropathy, cardiotoxicity, anaemias, blood clots, bone issues, dental problems and trouble breathing, etc., which ultimately affect the life quality of cancer patients. Moreover, chemotherapy is not cell-specific, hence normal tissues are also destroyed. Thus, using high-dose anti-cancer chemotherapeutics is not effective because of the lack of efficient targeting of payloads to target site (Refs Reference Oun, Moussa and Wheate27, Reference Itani and Al Faraj28).
Fortunately, latest novel treatments for lung cancer are rising with the purpose to boost up the life quality of cancer suffering patients and to avoid unwanted side effects concerned with conventional chemotherapy. Among such strategies, one is treating lung cancer through the delivery of biomacromolecules.
Pulmonary pathological changes and its influence on siRNA delivery
There are a variety of significant host factors that should be determined first when evaluating whether a patient with a given lung disease will benefit from siRNA inhalation. In determining whether medicine can be inhaled deep into the skin, pulmonary mechanics play a critical role. The lungs were preserved and made open to target cells. The regular fluctuation of respiration in most patients with asthma or chronic obstructive pulmonary diseases is sufficient for metered-dose inhalers, dry powder inhalers (DPIs) or nebulisers (Ref. Reference Usmani11). It is also important to understand tissue composition. The composition of airway tissue changes dramatically in obstructive lung diseases, such as asthma or chronic bronchitis, with increases in mucus production, stimulated immune cells, hypertrophy of the basement epithelial membrane, accompanying smooth muscle and its so many inflammatory factors. This often results in differences in the composition of the aqueous and mucous layers that cover the airway, thus reducing fluidity and increasing viscosity in most instances (Ref. Reference Fehrenbach, Wagner and Wegmann29). A major challenge remains whether siRNA particles can pass through this dynamic admixture en route to target cells that lie underneath.
The levels of endogenous DNA and actin filaments derived from necrotic neutrophils are increased in cystic fibrosis, adding further to the thick mesh airway structure (Ref. Reference McKelvey12). The increase in oxidative stress in cystic fibrosis improves the disulphide bridge between mucin sheets, which adversely changes the properties of mucus transport for particles (Ref. Reference Yuan13). Nucleic acid delivery vectors used in clinical cystic fibrosis studies have been shown to be unable to penetrate cystic fibrotic mucus effectively, most likely because of the formulation's positively charged surface that interacts with negatively charged mucus. In asthma, by defective close junction formation, the barrier function of the airway epithelium is disrupted. Increased epithelial resistance results from close junction proliferation. Nonetheless, the permeability of adherens junctions is decreased by pro-inflammatory cytokines and bacterial toxins, indicating that the barrier properties of the airway epithelium can differ with the condition of the disease (Ref. Reference Gon and Hashimoto14).
Barriers to nucleic acid-based pulmonary delivery
Physiological barriers
The treatments for pulmonary diseases are mainly by parenteral injection and pulmonary administration through intranasal instillation, aerosol or inhalation. Hence, the first barriers that nucleic acid drugs via these two routes encounter are blood and respiratory tract (Fig. 2).
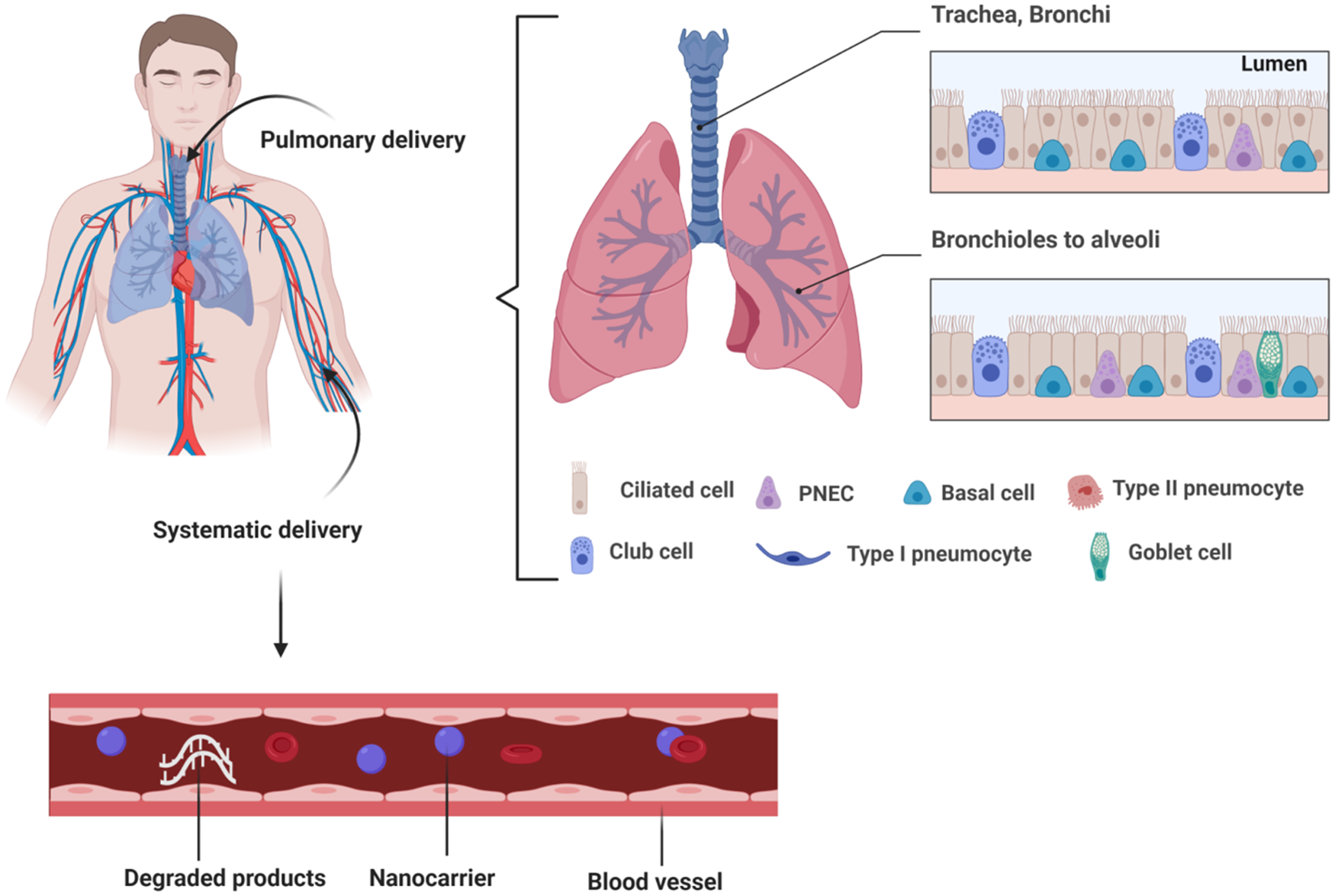
Fig. 2. Barriers to pulmonary delivery of therapeutics. Therapeutics administered via pulmonary route is removed by mucociliary actions present in mucus of lungs. Similarly, administration through systemic route leads to degradation of therapeutics by serum nucleases before going into the target site. Apart from these barriers, therapeutics also faces the challenge of engulfment by the endosomes. All these barriers lead to loss of therapeutic stability and immature release of cargo. The desired therapeutic effect or gene silencing by the payload is not obtained.
Therapeutics administered via pulmonary route is removed by mucociliary actions present in mucus of lungs. Similarly, administration through a systemic route leads to the degradation of therapeutics by serum nucleases before going into the target site. Apart from these barriers, therapeutics also faces the challenge of engulfment by the endosomes. All these barriers lead to loss of therapeutic stability and immature release of cargo. The desired therapeutic effect or gene silencing by the payload is not obtained (Ref. Reference Chen15). Parenteral administration of unmodified nucleic acids has been set back by their very short half-life in the bloodstream, serum nuclease degradation, quick renal clearance and poor biodistribution. The parenteral route of administration circulates the nucleic acid in the whole body so inefficiently delivers the loaded cargo at the target site (Ref. Reference Dammes and Peer20). To bypass serum nuclease enzymatic degradation and renal clearance of nucleic acids, local drug administration routes have been proposed to directly deliver the drugs to the site of interest. Pulmonary administration reveals a strong potentiality as it could transport therapeutic agents to diseased lung tissue in a non-invasive manner. While the degradation by nucleases is negligible compared to systemic administration, delivery through the airway could be hampered by physiological barriers. The mucociliary clearance (MCC) action, the surface liquid that lines the airway and carries macrophages along different parts of the airways, limits the transport of nucleic acids to the site of action (Ref. Reference Koli16). The highly viscous mucus layer in the airways traps and prevents nucleic acids reaching the underlying epithelium and propelled them out with the impact of ciliated cells (Ref. Reference de Souza Carvalho, Daum and Lehr17). Mucins with densely glycosylated and negatively charged regions are glycoproteins in nature. The mucus lining the epithelium of the airway that has one of the biggest barriers to siRNA delivery has been recognised. Electrostatic interactions with positively charged siRNA particles can capture the mucus layer particles, leading to increased MCC and reduced efficacy of delivery (Ref. Reference Dua18). Therefore, the development of particles that can sufficiently penetrate the mucus barrier, without disturbing its protective features, is a major challenge for improving pulmonary drug delivery.
Intracellular barrier
Even if the nucleic acids successfully penetrate through and escape from all the extracellular barriers mentioned previously, they still face the challenge to cross the cell membrane and reach the site of action in the cytoplasm or nucleus (Ref. Reference Durymanov and Reineke19). Negative charge and large molecular weight make it hard for naked nucleic acids to enter the cell (Ref. Reference Dammes and Peer20). The endocytosis of nucleic acids could be improved with the help of cationic biomaterials or targeting moieties which interact with the negative proteins or receptors on the cellular surface. One of the most challenging intracellular barriers for nucleic acids delivery is their tendency to remain entrapped in endosomes (Ref. Reference Pei and Buyanova21). Intracellular nucleic acids are transported in early endosomal vesicles where various nucleases exist and the pH further reduces to 4.5 in the process to delay endosomes and lysosomes, and most nucleic acids are degraded in the endosomes before reaching the site of action (Refs Reference Dominska and Dykxhoorn22, Reference Gao23).
There are four primary endocytosis pathways. Firstly, the most widely studied mechanism is clathrin-mediated endocytosis, which is concerned with receptor-mediated nanoparticle uptake. For example, in order to mediate endocytic uptake, transferring receptor, low-density lipoprotein receptor and epidermal growth factor receptors have been investigated. Second, caviling-dependent endocytosis, which follows nanoparticle attachment to the cell membrane, induces cytosolic caveolar vesicles. Next, micropinocytosis, which produces large extracellular fluid-containing macropinosomes and soluble proteins. Last, certain endocytic processes that depend on protrusions of the actin-driven membrane that then merges with and detaches from the plasma membrane to create macropinosomes (Ref. Reference Large24).
Airway epithelial cells are the main focus for therapy for most obstructive lung diseases. These cells, unfortunately, produce lower endocytic activity on the apical surface (Ref. Reference Kim30). Agents such as lysophosphatidylcholine, the natural airway surfactant or EGTA – the calcium chelator have been used to temporarily interrupt close epithelial junctions and enhance the in vivo entry of vesicular stomatitis viruses (Ref. Reference Cooney, McCray and Sinn31). Particles smaller than 150 nm permit endocytosis and also restrict the uptake of macrophages, which both help to prevent lung clearance (Ref. Reference Bardoliwala32). The classic approach has been to use small-molecule endosomatic agents such as chloroquine to disrupt endosomes and release entrapped oligonucleotides from endosomes. Two similar types of small molecules have been reported recently with the help of a high-throughput screen of chemical libraries. These molecules substantially enhanced the pharmacological activities of oligonucleotides both in cell culture and murine model (Refs Reference Chernikov37, Reference Degors38). Although these endosomatic agents significantly enhanced the delivery efficiency, they currently display a narrow therapeutic window for clinical use.
To overcome these biological barriers, strategies such as chemical modification, conjugation, vector encapsulation and selection of administration route have been utilised to improve the delivery of nucleic acids to the lungs.
Overwhelming strategies
Chemical modification and conjugation
Since naked nucleic acid is prone to degradation in the biological fluid (Ref. Reference Chow, Qiu and Lam33), chemical modification at the sugar, backbone or the individual bases has been introduced to boost its efficacy and stability in biological systems (Ref. Reference Dong, Siegwart and Anderson34). Phosphorothioate (PS)-modified backbone is the most commonly used chemistry modification to increase the nuclease resistance. Based on PS backbones, nucleic acids designed with additional 2′-sugar modifications, that is, 2′-O-methyl (2′- OME) or 2′-O-methoxyethyl (2′-MOE), can not only further enhance stability and target affinity, but also largely block the activation of toll-like receptors and reduce immune responses (Ref. Reference Liao35). Besides chemical modification, conjugation strategies are often exploited to enhance stability and delivery efficiency. Representative biomolecules conjugated to nucleic acids conclude targeting ligands and membrane-active molecules, such as lipids, aptamers, peptides, carbohydrates and polymers (Ref. Reference Lee36). Cholesterol attachment to nucleic acids facilitates cellular import and improves intracellular uptake via lipoproteins-mediated pathways (Ref. Reference Lee39). Intravenous and intra-peritoneal injection of anti-MDR1 cholesterol-siRNA conjugate in healthy and tumour-bearing severe combined immune deficiency mice demonstrated efficient accumulation in liver and tumour cells cytoplasm and deep tissues as well deep in the tissue and the cytoplasm of almost all the liver and tumour cells. siRNAs conjugated to N-acetyl galactosamine molecule, a high-affinity ligand for the hepatocyte-specific glycoprotein, are undergoing clinical trials and provided promising results (Ref. Reference Chernikov37). Antibodies or aptamers could be conjugated directly to nucleic acids to realise targeted delivery to specific tissues or cell types. Because of the advantages such as good reproducibility and low system toxicity, chemical modification and conjugation of nucleic acids have been paid great attention and all the four FDA-approved ASOs are chemically modified and used without a delivery vehicle. While compared to vector-based systems, poor delivery efficiency and limited orientation are still great concerns of nucleic acid conjugates for their clinical translation (Refs Reference Jung40–Reference Chang42).
Use of vectors
Besides chemical modification, vectors offer important opportunities for nucleic acids to overcome delivery challenges (Ref. Reference Degors38). Ideal nucleic acid delivery vectors are expected to condense and protect nucleic acids, facilitate their transport to target cells, and sub-cellular compartments. Viruses, as naturally evolved transfection agents (Ref. Reference Su, Sena-Esteves and Gao43), could enter the cells via endocytosis and release viral genome that could replicate and transcribe into proteins for producing multiple copies. Due to their higher transfection efficiency, three major classes of viral vectors, namely, adenovirus, adeno-associated virus and lentivirus have been extensively used in nucleic acid therapy. However, the limitation of payload, inherent immunogenicity and the difficulty of large-scale production limited their clinical application (Refs Reference Barba44–Reference Gomes dos Reis46).
The advantage of non-viral vectors lies in low immunogenicity and toxicity, ease of production and the large payload over their viral counterparts (Ref. Reference Patil47). Widely investigated non-viral delivery vectors include polymers, lipids, polypeptides and inorganic nanomaterials (such as calcium phosphate and quantum dots) (Ref. Reference Mohammadinejad48). Most of the vectors for nucleic acids possess cationic charges that assist in loading nucleic acids through charge interactions. Based on various non-viral vectors, hybrid systems made up of condensed nucleic acid/polycation complexes as the core and lipid bilayer membrane as the shell have been developed. The use of endogenous phospholipids, such as phosphatidylcholine, can be considered a valid approach to increase the compatibility of nanoparticles with the lung environment (Ref. Reference d'Angelo49). Researchers combined a naturally derived pulmonary surfactant shell with a siRNA-loaded dextran nanogel to achieve effective siRNA delivery to murine alveolar macrophages, which are difficult to transfect, resulting in a substantial gene knockdown with a relatively low dose (Ref. Reference De Backer50). Diverse surface modifications and conjugation of targeting agents attached to the vectors could render their desirable properties and enhance the therapeutic efficiency of nucleic acid therapy. Surface modification with high molecular weight hyaluronic acid which can mediate active CD44 targeting in tumours and increase circulation time of cationic siRNA lipoplexes improved the delivery efficiency and achieved supported reduction of the expression of luciferase mRNA in tumour due to the siRNA inhibition (Refs Reference Ivanova51–Reference Patra53).
A multipurpose nanodevice envelope that targets the lung endothelium supplies the cytoplasm with its encapsulated siRNA, and eliminates lung metastasis (Fig. 3). The secret to efficacy can be attributed to the existence of a dual function surface-modified GALA peptide: approaching the pulmonary endothelium sialic acid-terminated sugar chains and consequently distributing the encapsulated cargoes through endosomal membrane fusion to the cytosol, similar to the influenza virus. Such a vector can be a promising approach for next generation therapeutics (Ref. Reference Kusumoto54).

Fig. 3. Liposomes in vivo disposition modified through GAL. (a) Schematic presentation of the artificial influenza virus-like vector, GALA/MEND, for RNAi therapy. (b) Inhibition of the cellular uptake of GALA-LPs. The HMVEC-L was preincubated with 10 μM lectins, Maackia amurensis agglutinin (MAM) and Sambucus sieboldiana agglutinin (SSA), and N-acetylneuraminic acid, and the cells were subsequently incubated with rhodamine-labelled GALA-LPs for 2 h. Lysosomes and cell nuclei were stained with LysoTracker Green and Hoechst 33342. Rhodamine signals, LysoTracker Green and Hoechst 33342 signals are coloured red, green and blue, respectively. (c) Suppression of the progression of pulmonary metastasis by the administration of GALA/MEND. C57BL/6 mice were challenged with 2 × 105 B16F10-luc2 at day 0 to establish experimental pulmonary metastasis. GALA/MEND encapsulating negative controlled siRNA (anti-GL3) or anti-CD31 siRNA (1.0 mg siRNA/kg) was administered at 3-day intervals from day 1 to day 16. The mice were sacrificed at day 17. Reprinted with permission from (Ref. Reference Kusumoto54). GALA, an amphiphatic peptide; MEND, multifunctional envelope-type nanodevice; PEI, polyethylene imine; DOTMA, di-oleoyloxomropyl-trimethylammonium chloride; STR-mPEG, stearyl-polyethylene glycol; Chol, cholesterol; CV, coefficient of variation.
Liposomes in vivo disposition modified through GAL. (A) Schematic presentation of the artificial influenza virus-like vector, GALA/MEND, for RNAi therapy. (B) Inhibition of the cellular uptake of GALA-LPs. The HMVEC-L was preincubated with 10 μM lectins, Maackia amurensis agglutinin (MAM) and Sambucus sieboldiana agglutinin (SSA), and N-acetylneuraminic acid, and the cells were subsequently incubated with rhodamine-labelled GALA-LPs for 2 h. Lysosomes and cell nuclei were stained with LysoTracker Green and Hoechst 33342. Rhodamine signals, LysoTracker Green and Hoechst 33342 signals are coloured red, green and blue, respectively. (C) Suppression of the progression of pulmonary metastasis by the administration of GALA/MEND. C57BL/6 mice were challenged with 2 × 105 B16F10-luc2 at day 0 to establish experimental pulmonary metastasis. GALA/MEND encapsulating negative controlled siRNA (anti-GL3) or anti-CD31 siRNA (1.0 mg siRNA/kg) was administered at 3-day intervals from day 1 to day 16. The mice were sacrificed at day 17 (reprinted with permission from (Ref. Reference Kusumoto54)).
Formulation and route of administration
Systemic administration of nucleic acids faces serious challenges, including rapid excretion, low bioavailability and systemic toxicity. While local administration allows lower delivery doses and reduced side effects, making it an attractive route (Ref. Reference De Carvalho Vicentini55). Most of the FDA-approved nucleic acid-based drugs are locally delivered: Fomivirsen is delivered to the eyes by intraocular injection, Spinraza is by intrathecal injection, and Luxturna is by subretinal injection. For pulmonary disease, the target organ could be reached through systemic administration or pulmonary administration. The pulmonary route of administration could possibly enhance nucleic acid retention time in the desired target site, reduce systemic toxic effects, and provide a therapeutic solution to a range of pulmonary disorders (Refs Reference Chen15, Reference Barba44, Reference Lam, Liang and Chan56). Inhalation and intranasal route represent the most suitable way to target nucleic acid into the airways due to convenient administration and non-invasive characteristics, and are the main administration routes in clinical trials. Biodistribution studies of aerosol inhalation of polyester-siRNA nanoparticles to mice bearing orthotopic lung tumours showed specific accumulation in the lungs (Ref. Reference Yan45). Nucleic acids can be administered through inhaler or nebuliser by formulating it in liquid aerosol form, or dry powder aerosol for pulmonary delivery (Ref. Reference Gomes dos Reis46). Liquid aerosol formulations were almost exclusively adopted in clinical trials involving pulmonary delivery of nucleic acids. Among the three major types of inhalation devices consisting of pressurised metered-dose inhalers (pMDIs), nebulisers and DPIs, pMDIs and DPIs are the most portable and commonly used devices. pMDIs, which involve the suspension of therapeutic moieties in the hydro fluoro alkane propellant, have been regarded as a golden standard delivery system for asthma and COPD therapies (Ref. Reference Gomes dos Reis46).
A pMDIs formulation containing mannitol microparticles which encapsulated siRNA polyplex nanoparticles showed good aerodynamic properties for deep lung deposition and significant gene knockdown efficiency in lungA549 cells (Ref. Reference Ni57). DPIs are usually thought as a better option to deliver therapeutic nucleic acids than pMDIs because their dry particle form enhances the stability of nucleic acids and decreases the risk of microbial contamination (Ref. Reference Chow and Lam58). Chow et al. first formulated naked siRNA into inhalable dry powders (at 2% w/w) using spray drying technology with the incorporation of mannitol and L-leucine; the latter acted as powder dispersibility enhancer, and the integrity of siRNA was well retained. Although systemic administration does not provide the aforementioned advantages of local delivery, for some indications such as lung metastasis and pulmonary hypertension, the desired target sites might locate on the interstitium and lung alveolar and endothelial cells rather than the airway epithelium. Lung metastases are expected to have an endothelial origin and therefore may be better accessible through blood vessels than through airways (Ref. Reference Chen15). Although intravenous injection is not direct delivery to the lung, this route is still able to achieve high levels of transgenic expression in the lungs. A multifunctional lipid envelope-type nanodevice developed to target the lung endothelium was found to accumulate in the lung within 5 min after injection. This carrier did not quickly move to other organs and remained in the lungs for 6 h. Based on this carrier, systemic administration of anti-CD31 siRNA successfully suppressed the metastatic progression (Ref. Reference Kusumoto54). Therefore, the administration route should be carefully chosen according to the therapeutic application.
Nanoparticle-based pulmonary delivery
Nanotechnology has opened new corridors for the delivery of anti-cancer agents towards tumour site owing to its accurate and efficient targeting capability. Nanotechnology-based delivery involves the encapsulation of anti-cancer agents in nano-particles (nano-carriers) that precisely delivers the cargo by reducing undesired side effects – owing to change in the pharmacokinetic and bio-distribution properties of the loaded cargo (Ref. Reference Allen and Cullis59). Nanoparticle-based therapeutics efficiently delivers peptides, antibodies, radio nuclides, hydrophilic and hydrophobic drugs to the target site accurately as compared to conventional chemotherapy that does not carry the whole cargo to the target (Ref. Reference Liu60). Some of the main advantages of nanoparticle-mediated therapeutics include, reducing wanted side effects, reduction in dose, minimizing drug-related toxicity, boosting drug plasma half-life, controlling drug release and enhancing permeability and retention effect (Ref. Reference Ivanova51). Additionally, altering chemical and physical properties of encapsulated agents results in improved localisation of cargo at the target site and increases tumour response to the delivered agent (Ref. Reference Parhi, Mohanty and Sahoo52). Lately, a diverse range of nanoparticles have been fabricated and designed specifically to deliver anticancer drugs and nucleic acids (RNA/DNA) to the lungs thus starting new channels to lung cancer therapy (Ref. Reference Patra53). These nanoparticles are classified briefly in the following figure (Fig. 4)

Fig. 4. Representative of the employed nanocarriers for siRNA delivery and type of administration.
Nanoparticles based on lipids
Liposomes and micelles are the two main categories of lipid-based nanoparticles that are vastly explored (Ref. Reference Ansari61). Structurally, liposomes are composed of an inner layer that is aqueous in nature encapsulating hydrophilic agents and an outer phospholipids layer that consolidates the hydrophobic agent in its environment. To achieve maximum effects, extended blood circulation and efficient tumour localisation, the lipid-based nanoparticles are tuned in terms of their size, shape and charge (Ref. Reference Narenji, Talaee and Moghimi62). By nature, these nanoparticles are inert, non-toxic, compatible with body, lacking immunogenicity and soluble, that's why they are widely used as a delivery carrier for anti-cancer therapeutics. ‘Stealth liposomes’ are very rarely detected by the reticulo-endothelial system and phagocytosis, thus it prolongs the circulation half-life of loaded cargo up to 44 h and improving the treatment efficiency (Ref. Reference Chuang63).
Polymer-based nanoparticles
Nanoparticles fabricated from polymers are a major part of nano-medicines that include polymeric micelle, dendrimers, polymersomes, polymer-lipid hybrids, etc. (Refs Reference Chen, Ng and Weil64, Reference Liarou65). They present a versatile class of therapy entities as an anticancer therapy (Refs Reference Indoria, Singh and Hsieh66, Reference Shukla67). Combination therapy for albumin nanoparticles has been approved by FDA encapsulating paclitaxel and carboplatin for the NSCLC treatment in A549 xenograft lung tumour model (Ref. Reference Jung40) (Table 1).
Table 1. The overwhelming strategies with their definition and examples

Targeting other pulmonary diseases via biomacromolecule-based anti-sense oligos
Pulmonary fibrosis
It has been shown in the literature that biomacromolecule-mediated siRNA delivery to lung cancer is effective; however, it faces some challenges. Besides, biomacromolecule-mediated anti-sense oligos were also delivered to treat multiple pulmonary diseases. Among them, a severe lung condition with minimal treatment options is pulmonary fibrosis. This fibrotic disease is mimicked through the action of TGF-β (transforming growth factor β). To treat pulmonary fibrosis, this cytokine is a significant target (Ref. Reference Miao41). A research study in this area was carried out to highlight the downregulation of TGF-β in pulmonary fibrosis (Ref. Reference Chang42). A dimeric human β-defensin 23 was complexed with polymeric anti-sense oligonucleotide for targeted pulmonary fibrosis delivery. The results indicated that anti-sense oligonucleotide showed improved uptake and serum stability both in vitro and in vivo (Ref. Reference Kim68). Similarly, NLRP3 are considered active in controlling inflammatory cytokines that lead to pulmonary fibrosis. The anti-sense oligo therapy for NLRP3 can be used as an effective treatment for pulmonary fibrosis (Ref. Reference Bai69). It shows that antisense oligo delivery can be employed as a promising gene delivery platform for the treatment of multiple lung diseases with low side effects.
COPD and asthma
Excessive production and inadequate clearance of mucus along with goblet cell metaplasia are the exacerbating factors towards COPD and asthma. In addition, multiple types of cell including goblet cells are modulated by notch signalling. An anti-sense oligonucleotide was delivered to mice model with asthma targeting notch signalling pathway. It was concluded that the chemically modified anti-sense oligonucleotide significantly reduced mucus production and goblet cell metaplasia (Ref. Reference Carrer70). It indicates that multiple types of chronic respiratory disorders can be treated using anti-sense oligo therapy.
Pulmonary delivery of cargoes is exposed to two main concerns, that is, leakage to blood due to lung small molecule permeability and immune response (Ref. Reference Glassman71). These two concerns must be considered during lung therapy. Moreover, the alveolar phagocytic macrophages that reside in alveolar spaces can be overcome through the accumulation of locked nucleic acid complexed with antisense oligos and exert a knockdown effect. These associated risks can be overwhelmed by the aforementioned locked nucleic acid complexed with antisense oligos (Ref. Reference Yoon72). Such a hypothesis was tested in a research study that reveals that targeting inhaled locked nucleic acid complexed with antisense oligos to macrophages successfully accumulates in alveolar macrophages and avoids the concern leakage into the bloodstream (Ref. Reference Uemura and Kobayashi73). This strategy will pave the way for inhalational therapeutics in pulmonary delivery of cargoes, especially anti-sense oligos. The pro-inflammatory cytokines are responsible for lung inflammation. Microparticle-loaded anti-sense oligonucleotide was targeted to NF-κB for the inhibition of pro-inflammatory cytokine. The results show that microparticle-based anti-sense oligonucleotide delivery significantly reduces the level of inflammatory cytokine release and can be used as an effective approach for the treatment of pulmonary inflammation (Ref. Reference Ubale74).
Drug delivery technologies based on micro and nanoplatforms employing anti-sense oligonucleotide provide a range of applications and promising therapeutic solutions for multiple pulmonary pathological conditions (Ref. Reference Vij75). The disturbed ion/water balance also may lead to various pulmonary consequences.
Cystic fibrosis
In a recent study, anti-sense oligonucleotide was targeted to epithelial sodium channels for the purpose to address sodium hyperabsorption during the treatment of cystic fibrosis. The results indicate that targeting epithelial sodium channel with anti-sense oligonucleotide significantly down-regulates the epithelial sodium channel and thus reducing sodium currents during cystic fibrosis (Ref. Reference Kolonko76). Genetic conditions-associated pulmonary disease leads to significant morbidity and mortality, especially in paediatric population. Anti-sense oligonucleotide therapy was applied in paediatric population with surfactant protein metabolism and cystic fibrosis disorders. This therapy has given fruitful results yet needs further exploration (Ref. Reference Martinovich77). In order to assess the therapeutic potential of this therapy in the treatment of lung disorders of genetic origin, investigations into specific molecular targets via prospective anti-sense oligonucleotides will provide an effective therapy platform.
Comprehensive summary of challenges and their possible solutions
Although nanoparticle-based therapeutics efficiently delivers the intact cargo with accurate targeting; however, in case of inhalational therapy for lung cancer, the translation of such therapeutics is a major challenge (Refs Reference Guk78, Reference Patil79). In addition to it, fabrication of lipid-based nanoparticles with an optimal size distribution, giving shelter from rapid clearance and providing more resident time at the target site are complex and extremely challenging (Ref. Reference Mukhtar80). Thus, it is mandatory to determine the biological behaviours (size, shape, surface properties) of such therapeutics in physiological environment. Apart from it, the aggregation of nanoparticles due to poor optimisation, interaction between particles, protein adsorption on the surface of nanoparticles and triggering of immunological responses are also some main hurdles in the path of such targeted delivery. In the case of siRNA delivery to lung cancer, it needs to overcome the intracellular and extracellular barriers (Ref. Reference Vermeulen81). Lungs comprise phagocytes and macrophages that engulf the siRNA encapsulated nanoparticles, overwhelming such phenomenon is also an extremely challenging aspect for the nanoparticle-based delivery of biomacromolecules. This issue can be overcome by formulating injectable form with surface modification of nanoparticles that will not be detected by the immune cells (Ref. Reference Wang and Mooney82). Also, reducing the interaction between siRNA encapsulated nanoparticles and other particles can also help to reduce such immunogenicity (Ref. Reference Baharifar83).
Intracellular barriers cause partial delivery of siRNA to the target site (Ref. Reference Ni, Feng and Chau84). It includes endocytosis and reticulo-endothelial system; both pose threat to the accurate delivery of siRNA-linked nanoparticles (Ref. Reference Sawada85). In case of endocytosis, the siRNA-nanoparticles complex is engulfed by phagocytes and pre-target release occurs that leads to the failure of therapy (Ref. Reference Ahmadzada, Reid and McKenzie86). Similarly, the siRNA-nanoparticles complex detected by the reticulo-endothelial system transfers the cargo to the spleen and liver instead of the target site. This ultimately results in the unavailability of therapeutic cargo at the desired site. To overcome these issues, size, shape and surface modifications of a carrier are the possible solutions to the efficient delivery of siRNA into the lungs. The siRNA must escape from the endosomes into the cytoplasm upon endosomal cellular entry. Several methods have been studied to resolve and facilitate siRNA endosomal escape, which is directly linked to pulmonary transmission (Ref. Reference Saw and Song87). Effective targeting via ligand substituent's binding on the surface of target cells to over-expressed receptors is a widely studied technique to increase cellular uptake and improve therapeutic efficacy (Ref. Reference Hong88). For example, transferring and melittin-PEI conjugate T-cell targeted pulmonary siRNA delivery demonstrates efficient uptake into active T cells and endosomal escape for the treatment of asthma (Ref. Reference Keil, Baldassi and Merkel89). Since cholesterol is an integral part of the cell membrane and can be metabolised, it is sometimes used as a lipid anchor to increase stability and cellular diffusion, as well as to decrease the carrier's cytotoxicity (Ref. Reference Ercole90). SiRNA particles must resolve many intracellular barriers, not all of which are limited to endosomes and lysosome, once they have been taken by the target cells. In addition to the lungs, various organs and tissues share these barriers and have been thoroughly studied extensively (Ref. Reference Bansal91).
Currently, there are no specific rules and regulations for the analysis and testing of nanoparticle-based therapeutics, that is, manufacturing procedures, safety and testing. Just like other newly developed entities, such therapeutics also need evaluation check such as optimal design, reproducible production process, valid analytical testing procedures, valid characterisation analytical procedures, toxicology, pharmacokinetic profile and more importantly effective clinical trials. Unlike, conventional therapy, nanoparticles-based therapeutics is multi-component and complex that needs more modifications for efficient availability in next-generation dosage forms.
Endosomal engulfment of siRNA conjugates is carried out by endosomes before reaching the target site and the whole cargo is either fully or partially disrupted (Ref. Reference Stewart92). Such type of challenge can be overcome by using certain sort of endosomal escape strategies including (1) proton sponge effect that involves the use of buffering agent in the composition of siRNA conjugates. At low pH of cell environment, the agent gets protonated, absorbs more ion and water and bursts the endosomes releasing the intact payload with destruction (Ref. Reference Guk78); (2) endosomal membrane pore formation – use of peptides (cationic hydrophobic peptides) in the conjugate system when touches the membrane of endosomes causes a reduction in the surface tension of membrane, forming a pore which delivers the cargo in the intact form to the cytosol; (3) membrane fusion – using fusogenic peptides in acidic pH leads to fusion in the endosomal membrane bypassing the intact conjugate system without disruption by endosomes; (4) photochemical internalisation – in this sort of strategy, photosensitisers are attached with the delivery conjugate system. Upon exposure to light, these sensitisers produce singlet oxygen species which are too reactive and cause the blast of endosomes and release the intact payload without disruption into the cell (Ref. Reference Patil79).
siRNA is negatively charged (Ref. Reference Michlewska93) and exhibition of the same charge on cellular membrane avoids its entrance to the cellular environment. Also, siRNA is degraded in the serum leading to inaccurate delivery of payloads to the target site. That's why it's a big challenge for the delivery of siRNA to target sites. Encapsulating siRNA in polymeric nanoparticles provides stability and ensures efficient delivery. Cationic polymers are used to deliver negatively charged siRNA but toxicity (off-target effects) of cationic polymers is a keen challenge for the delivery of SiRNA. However, the use of anionic polymers can overcome the posing challenge. MCC is another big dilemma. Several techniques for successful penetration of the mucus layer and avoidance of MCC have been studied, including mucus-penetrating particles (MPPs). MPPs diffuse through mucus and resist MCC, as opposed to mucoadhesive particles that are embedded in the luminal mucus layer and removed by the MCC. The surface characteristics of the mucus-penetrating particles are important in improving the penetration of mucus. Mucus-penetrating particles must be small enough to resist trapping in the mucin mesh and also have a water-soluble and favourable surface charge to prevent contact with the mucin groups that are lipophilic and negatively charged (Ref. Reference He94). Meticulous approaches for surface engineering are therefore required. Dense surface coating with polyethylene glycol (PEG) was the most promising approach among those. PEG coating on the surface influences the distribution of siRNA particles in the lung, mucus penetration and lung-residence time (Ref. Reference Osman95). A newly documented substitute to PEG to promote mucus penetration focuses on a special combination of perfluoroalkyl polymers' lip phobic and hydrophobic properties (Ref. Reference Ge96). SiRNA distribution, however, is mostly based on lipid-soluble and positively charged carriers. In case of siRNA encapsulated in aerosol or dry powder dosage form, the particle size and mean size distribution must be between 1 and 5 μm (Ref. Reference Asadi97). Below the lower limit, the particles will get out of the bronchial environment during normal breathing, while above the high limit, the particles will precipitate in the throat instead of going into the lungs. Thus, desired particle size distribution is too mandatory for such sort of delivery. This can be achieved by using optimal quantities of dispersants such as mannitol and L-lucine.
The antisense oligonucleotide delivery is easy and effective to multiple vital organs such as the liver, eyes and nervous system (Ref. Reference Kuijper98). However, due to complex pulmonary anatomy targeting the deepest pulmonary areas is challenging. Thus, considering a novel delivery system that can target deeper areas of the lung can overcome this issue. The rapid clearance and turnover of anti-sense oligonucleotide are other key challenges to its pulmonary delivery (Ref. Reference Geary99). The choice of therapeutic modalities and treatment repetition can bypass the concern issues. The higher cost for anti-sense oligo formulation is another challenge for the pharmaceutical industry. Thus exploring novel cost-effective methods for the production of such formulations will promote disease therapy. Detection of polymeric nanoparticles by the reticulo-endothelial system followed by rapid elimination from the body is another barrier and keen challenge for polymeric-based siRNA therapeutics. One of the possible solutions for such a problem is the decoration of polymeric nanoparticles with bio-mimetic materials that shelter the nanomaterials from the immune system, efficiently releasing the intact payloads at the target site. Clinical safety is another challenge associated with polymer-based siRNA therapeutics, which needs specific attention and more clinical observation and research at the clinical level (Ref. Reference Saw and Song87). In addition to it, efficiently knowing and understanding the microenvironment of lung tumours targeted by siRNA can hopefully overcome the associated issues and can lead to the optimal transition of polymer-based nanoparticles-siRNA conjugates clinical translation.
Conclusion
The efficiency of biomacromolecules is affected by many factors within the biological system. The consequence of each factor should carefully be evaluated experimentally to derive a more predictable translational outcome. Biological systems exhibit many barriers, thus use of novel and smart materials are required to successfully deliver the payloads at target site in intact form.
In addition to it, to boost up the translational processes from pre-clinical evaluation to clinical trials, instead of a single model, more animal models should be used for the successful predicted outcomes. The consequence of each factor should carefully be evaluated experimentally to derive more outcomes.
The substantial progress in the production of siRNA-based formulations will continue to highlight its future therapeutic potential. The appropriate nanocarriers' framework would shield the therapeutic RNAi from degradation due to the circulatory environment and would deliver the therapeutic agent to tumour cells in an efficient manner. Future work needs to focus on obtaining well siRNA delivery to the targeted cells without any off-target effects, increasing nuclease resistance, and minimizing immune responses. Nano-siRNA delivery offer great cancer treatment; more integrative investigation is required in this emerging and promising field of nano-siRNA based delivery. Safety and efficacy of siRNA-based therapeutics need an efficient investigation.
In a nutshell, fabrication, design of smart material system and then its proper assessment (strategies evaluation) would possibly result in an efficient translation of biomacromolecules. Such an overcoming of barriers can result in carrying the bimolecular-based therapeutics from bench top to patients effectively enhancing the life quality of patients with lung cancer and other pulmonary diseases.