In this study, we provide a new, comparative description of Chondrenchelys problematica (Traquair Reference Traquair1888b) (Fig. 1) from the Lower Carboniferous (Viséan) of Scotland. C. problematica is the earliest undoubted holocephalan (Moy-Thomas Reference Moy-Thomas1935; Patterson Reference Patterson1965) known from both substantial cranial and postcranial material. Three exceptionally preserved specimens, discovered by Mr. S. P. Wood, are central to our project. Two of the specimens are new to science, while the third was previously misidentified as an actinopterygian. The principal pair of specimens derives from the Mumbie Quarry exposure (Coates & Gess Reference Coates and Gess2007) of the Glencartholm fish beds (Schram Reference Schram1983; Dineley & Metcalf Reference Dineley and Metcalf1999). Each of the fossils has been prepared to a higher standard than previously described specimens of C. problematica (Traquair Reference Traquair1888b; Moy-Thomas Reference Moy-Thomas1935), facilitating a more detailed analysis of this remarkable fish. Such data-rich material contributes directly to our broader aims of improving the understanding of early chondrichthyan diversity, and broadening the range of morphological data available for phylogenetic analyses of early chondrichthyans.

Figure 1 Traditional reconstruction of Chondrenchelys problematica, from Moy-Thomas (Reference Moy-Thomas1935, text-fig.12), as used and adapted in many standard references.
Extant Chondrichthyes (cartilaginous fish) comprises two reciprocally monophyletic clades, Elasmobranchii (sharks, skates, and rays) and Holocephali (chimaeras: including ratfish, rabbitfish, spookfish and elephant sharks) (Maisey Reference Maisey1984; Winchell et al. Reference Winchell, Sullivan, Cameron, Swalla and Mallatt2002; Inoue et al. Reference Inoue, Miya, Lam, Tay, Danks, Bell, Walker and Venkatesh2010; Licht et al. Reference Licht, Schmuecker, Huelsken, Hanel, Bartsch and Paeckert2012). Crown-clade holocephalans (chimaeroids) are characterised by a suite of highly distinctive apomorphies, including the complete fusion of the palatoquadrate with the neurocranium (holostyly), a dentition of large tooth plates, a “complete” hyoid arch possessing a pharyngohyal cartilage (but see: Maisey Reference Maisey1984; Didier Reference Didier1995), branchial arches positioned under the otic capsule with an associated anterior displacement of the mandible, large, narrowly-separated orbits positioned high on the cranium, and a polyspondylous axial skeleton composed of chordacentra (Dean Reference Dean1906; de Beer & Moy-Thomas Reference de Beer and Moy-Thomas1935; de Beer Reference de Beer1937; Patterson Reference Patterson1965; Didier Reference Didier1995, Stahl Reference Stahl and Schultze1999; Gillis et al. Reference Gillis, Rawlinson, Bell, Lyon, Baker and Shubin2011). There is also a high degree of sexual dimorphism in holocephalans, with pre- and postpelvic claspers and a frontal clasper organ (tenaculum) in males (Stahl Reference Stahl and Schultze1999).
Monophyly of the crown clades of Chondrichthyes, Elasmobranchii and Holocephali is not in doubt: unequivocal support from both molecular and morphological data exists for each of these clades. However, the early fossil membership of each of these groups, especially as concerns the stem lineages preceding the crown-clade radiations, is uncertain. This ambiguity is linked to a more general problem in early chondrichthyan phylogeny: fossil shark-like fishes that are not holostylic have historically been classified as elasmobranchs. The result of this circular criterion for taxonomic assignment is an elasmobranch–holocephalan split that is deep within the chondrichthyan tree (Maisey Reference Maisey2012).
Lack of any substantial evidence to support such a profound divergence led Maisey (Reference Maisey2012) to conclude that “Elasmobranchii” should refer solely to the crown-clade and its immediate fossil relatives, while also commenting, “considerable progress has been made in elucidating the evolution of holocephalans.” In fact, published phylogenies of fossil holocephalans show little agreement. Grogan et al.'s (Reference Grogan, Lund, Greenfest-Allen, Carrier, Musick and Heithaus2012) tree of chimaeriforms and their fossil relatives differs profoundly from Stahl (Reference Stahl and Schultze1999), and both conflict with the topology of the holocephalan taxa in Pradel et al. (Reference Pradel, Tafforeau, Maisey and Janvier2011). This problem persists at deeper phylogenetic levels. Symmoriids (Zangerl Reference Zangerl and Schultze1981; Maisey Reference Maisey2007), a distinctive and diverse group of Palaeozoic chondrichthyans, might be stem-holocephalans (Janvier Reference Janvier1996; Coates & Sequeira Reference Coates and Sequeira2001), stem-elasmobranchs (Coates & Sequeira Reference Coates and Sequeira2001; Grogan et al. Reference Grogan, Lund, Greenfest-Allen, Carrier, Musick and Heithaus2012) or even stem chondrichthyans (Pradel et al. Reference Pradel, Tafforeau, Maisey and Janvier2011; Davis et al. Reference Davis, Finarelli and Coates2012). Moreover, recent conjectures about plesiomorphic holocephalan conditions include revived comparisons with placoderm-grade fishes (Trinajstic et al. Reference Trinajstic, Long, Johanson, Young and Senden2012), perhaps including Stensioella (Janvier Reference Janvier1996; Pradel et al. Reference Pradel, Maisey, Tafforeau and Janvier2009b), as well as speculation that the apparent Devonian chondrichthyan Gladbachus might be a stem-holocephalan (Heidtke & Krätschmer Reference Heidtke and Krätschmer2001).
This is not to say that the current state of affairs is entirely negative. New and detailed data on Palaeozoic chondrichthyans are becoming increasingly available (Maisey Reference Maisey2005, Reference Maisey2007; Pradel et al. Reference Pradel, Maisey, Tafforeau and Janvier2009a, Reference Pradel, Tafforeau, Maisey and Janvier2011, Reference Pradel, Maisey, Tafforeau, Mapes and Mallatt2014; Maisey & Lane Reference Maisey and Lane2010; Pradel Reference Pradel2010), complementing the extraordinary diversity of holocephalans from the Upper Mississippian of Bear Gulch (see: Grogan et al. Reference Grogan, Lund, Greenfest-Allen, Carrier, Musick and Heithaus2012 and references therein; and classic studies by Stahl (Reference Stahl and Schultze1999) and Patterson (Reference Patterson1965)). Additionally, the recent publication of the elephant shark genome (Callorhinchus milii) (Venkatesh et al. Reference Venkatesh, Lee, Ravi, Maurya, Lian, Swann, Ohta, Flajnik, Sutoh, Kasahara, Hoon, Gangu, Roy, Irimia, Korzh, Kondrychyn, Lim, Tay, Tohari, Kong, Ho, Lorente-Galdos, Quilez, Marques-Bonet, Raney, Ingham, Tay, Hillier, Minx, Boehm, Wilson, Brenner and Warren2014) has promoted interest in holocephalans as a potential model system for comparative genomics (Venkatesh et al. 2007; Larsson et al. Reference Larsson, Tay, Sundstrom, Fredriksson, Brenner, Larhammar and Venkatesh2009), evolutionary physiology (Baker Reference Baker2010, Kakumura et al. Reference Kakumura, Watanabe, Bell, Donald, Toop, Kaneko and Hyodo2009), sensory system evolution (Davies et al. Reference Davies, Carvalho, Tay, Hunt, Brenner and Venkatesh2009; Niimura Reference Niimura2009; Callaway Reference Callaway2012) and the evolution of developmental regulatory mechanisms (Gillis et al. Reference Gillis, Dahn and Shubin2009; Mulley et al. Reference Mulley, Zhong and Holland2009; Ravi et al. Reference Ravi, Lam, Tay, Tay, Brenner and Venkatesh2009; MacDonald et al. Reference MacDonald, Debiais-Thibaud, Martin, Poitras, Tay, Venkatesh and Ekker2010; Oulion et al. Reference Oulion, Debiais-Thibaud, d'Aubenton-Carafa, Thermes, Da Silva, Bernard-Samain, Gavory, Wincker, Mazan and Casane2010).
It is commonly assumed that holocephalans represent primitive conditions for jawed vertebrates, both morphologically (Carroll Reference Carroll1997) and genomically (Ravi et al. Reference Ravi, Lam, Tay, Tay, Brenner and Venkatesh2009; Inoue et al. Reference Inoue, Miya, Lam, Tay, Danks, Bell, Walker and Venkatesh2010; Venkatesh et al. Reference Venkatesh, Lee, Ravi, Maurya, Lian, Swann, Ohta, Flajnik, Sutoh, Kasahara, Hoon, Gangu, Roy, Irimia, Korzh, Kondrychyn, Lim, Tay, Tohari, Kong, Ho, Lorente-Galdos, Quilez, Marques-Bonet, Raney, Ingham, Tay, Hillier, Minx, Boehm, Wilson, Brenner and Warren2014). Yet, long internal branches separate the most recent common ancestor of the holocephalan crown clade from that of the chondrichthyan crown (Maisey Reference Maisey1984; Inoue et al. Reference Inoue, Miya, Lam, Tay, Danks, Bell, Walker and Venkatesh2010; Licht et al. Reference Licht, Schmuecker, Huelsken, Hanel, Bartsch and Paeckert2012), and the degree of lost morphologic and taxonomic diversity along each branch is unknown. Living chimaeroids are anatomically conservative, in the sense that we observe low morphologic disparity within the clade today, but this modern body plan is not representative of the morphologic diversity in early holocephalans (Patterson Reference Patterson1965; Pradel Reference Pradel2010; Finarelli & Coates Reference Finarelli and Coates2012; Grogan et al. Reference Grogan, Lund, Greenfest-Allen, Carrier, Musick and Heithaus2012). A better understanding of the skeletal anatomy of early holocephalans, such as Chondrenchelys, is needed to shed further light on the evolution of chondrichthyan body plans; to corroborate inferences drawn from fossils preserved in contrasting conditions (Lund Reference Lund1982; Lund & Grogan Reference Lund, Grogan, Arratia, Wilson and Cloutier2004); to resolve phylogenetic relationships (Brazeau Reference Brazeau2009; Davis et al. Reference Davis, Finarelli and Coates2012; Grogan et al. Reference Grogan, Lund, Greenfest-Allen, Carrier, Musick and Heithaus2012; Zhu et al. Reference Zhu, Yu, Ahlberg, Choo, Lu, Qiao, Qu, Zhao, Jia, Blom and Zhu2013); to test hypotheses of tempo and mode of morphological and molecular evolution (Inoue et al. Reference Inoue, Miya, Lam, Tay, Danks, Bell, Walker and Venkatesh2010; Davis et al. Reference Davis, Finarelli and Coates2012; Licht et al. Reference Licht, Schmuecker, Huelsken, Hanel, Bartsch and Paeckert2012; Venkatesh et al. Reference Venkatesh, Lee, Ravi, Maurya, Lian, Swann, Ohta, Flajnik, Sutoh, Kasahara, Hoon, Gangu, Roy, Irimia, Korzh, Kondrychyn, Lim, Tay, Tohari, Kong, Ho, Lorente-Galdos, Quilez, Marques-Bonet, Raney, Ingham, Tay, Hillier, Minx, Boehm, Wilson, Brenner and Warren2014); and to inform hypotheses of evolutionary developmental biology (Gillis et al. Reference Gillis, Dahn and Shubin2009; Ravi et al. Reference Ravi, Lam, Tay, Tay, Brenner and Venkatesh2009; Oulion et al. Reference Oulion, Borday-Birraux, Debiais-Thibaud, Mazan, Laurenti and Casane2011) in this species-poor, but fundamental division of living vertebrate diversity.
1. History and context
1.1. History of discovery, previous interpretations and likely related fossil material
Traquair (Reference Traquair1888b) erected Chondrenchelys problematica on the basis of specimens from Glencartholm, Scotland. Moy-Thomas (Reference Moy-Thomas1935) re-described Chondrenchelys in detail, providing the standard reconstruction (Fig. 1). This was reviewed briefly by Patterson (Reference Patterson1965), and has subsequently been reproduced in numerous texts, notably Stahl's (Reference Stahl and Schultze1999) handbook on the Holocephali. Chondrenchelys is allied with holocephalans based on its fused tooth plates and holostylic skull (Moy-Thomas Reference Moy-Thomas1935; Patterson Reference Patterson1965; Stahl Reference Stahl and Schultze1999). Lund (Reference Lund1982) re-described key anatomical features of Chondrenchelys in his comparative description of the slightly younger holocephalan Harpagofututor volsellorhinus from the Bear Gulch fauna, placing both genera in the Family Chondrenchelyidae (Berg Reference Berg1940), based on their anguilliform (eel-like) body plans, and general similarities in the morphologies of the pectoral fin and tooth plates.
To date, Chondrenchelys remains monotypic, although Ginter & Sun (Reference Ginter and Sun2007) provisionally assigned isolated tooth plates from China to Chondrenchelys sp. The morphology of the dentition and inferred mode of dental growth in Chondrenchelys was completely revised (Finarelli & Coates Reference Finarelli and Coates2012), based upon specimens that we further describe here. In addition, isolated tooth plates matching known chondrenchelyids include those of the ichthyolith genus Platyxystrodus (Hay Reference Hay1899), as reviewed by Lund (Reference Lund1982, table 1) and Stahl (Reference Stahl and Schultze1999, fig. 46 A–J). These range stratigraphically from the Lower to Upper Carboniferous of Great Britain, Northern Ireland, Belgium, North America and Russia. Xystrodus striatus (McCoy Reference McCoy1855) (now Platyxystrodus), specimens GS 48785–48788 of the British Geological Survey collections at Keyworth (UK), originating from the Carboniferous Limestone series of Cumbria and Northumberland, England, are strikingly similar to the lower posterior tooth plates of Chondrenchelys.
1.2. Geological context and age of Mumbie Quarry
The two new Chondrenchelys specimens, NMS 1998.35.1 and NMS 2002.68.1, originate from the Mumbie Quarry locality (Fig. 2), a site opened and developed by the distinguished Scottish fossil collector S. P. Wood (Coates & Gess Reference Coates and Gess2007; Finarelli & Coates Reference Finarelli and Coates2012). Mumbie Quarry is adjacent to exposures of the Glencartholm Volcanic Beds (GVB), and is laterally equivalent to the Glencartholm Fish Bed (GFB) (Dineley & Metcalf Reference Dineley and Metcalf1999) that produced the classic Chondrenchelys material (Traquair Reference Traquair1888b; Moy-Thomas Reference Moy-Thomas1935): Glencartholm, Dumfries District, Dumfries and Galloway Region, Scotland, Ordnance Survey grid reference [NY 376 795]. The GFB comprises a thin unit within the GVB, which is in turn part of the larger Upper Border Group of the Calciferous Sandstone (Lumsden et al. Reference Lumsden, Tulloch, Howells and Davies1967). This unit was deposited in a near-shore marine environment (Schram Reference Schram1983). The GVB span the Holkerian/Asbian regional substage boundary (Livian/Warnantian) of the early Viséan Stage, Mississippian Subsystem, Early Carboniferous (George et al. Reference George, Johnson, Mitchell, Prentice, Ramsbottom, Sevastopulo and Wilson1976; Cossey et al. Reference Cossey, Adams, Purnell, Whitely, Whyte and Wright2004), approximately 336.5 Ma (Gradstein et al. Reference Gradstein, Ogg, Schmitz and Ogg2012).

Figure 2 Map of southern Scotland detailing several important early vertebrate localities, including the Glencartholm Fish Beds, from which the original Chondrenchelys material described by Moy-Thomas (Reference Moy-Thomas1935) is derived, and the Mumbie Quarry locality, opened by S. P. Wood, from which the new specimens, NMS 1998.35.1 and NMS 2002.68.1 are derived. Base maps of the Republic of Ireland and the United Kingdom from Geomapapp: http://www.geomapapp.org. Inset map of the Glencartholm area redrawn from fig. 9.16, in Dineley & Metcalf (Reference Dineley and Metcalf1999). Glencartholm Fish Beds from Ordnance Survey Grid reference [NY 376795].
2. Materials
2.1. Image preparation
Photographs of fossils were prepared using a Leica DFC490 camera attached to a Zeiss Stemi SV6 microscope. Line drawings were prepared using a camera lucida attachment installed for this microscope. Digital image processing was performed in Image-Pro Plus 6.2. We created multiple z-stacks of digital images, which were aligned and processed using the enhanced depth of field function. We note in the figure captions when specimens were whitened with ammonium chloride to enhance depiction of surface profile detail. Figure 6e was produced by means of reflectance transformation imaging (RTI): for technical details, see: http://culturalheritageimaging.org/Technologies/RTI/. Details on the settings used within the GNU-licensed RTI software application (RTIViewer) are provided in the figure caption.
2.2. Abbreviations
Explanations of anatomical abbreviations are provided in figure captions. Terminology follows Patterson (Reference Patterson1965) and Didier (Reference Didier1995; Didier et al. Reference Didier, Kemper, McEachran, Carrier, Musick and Heithaus2012).
Institutional abbreviations. NMS, National Museum of Scotland, Edinburgh, Scotland, UK; RSM, Royal Scottish Museum, Edinburgh, Scotland, UK (now the NMS); BGS-GSE, British Geological Survey, Edinburgh, Scotland, UK; HM, Hunterian Museum, University of Glasgow, Glasgow, Scotland, UK; NHMUK, Natural History Museum, London, UK; CMNH, Carnegie Museum of Natural History, Pittsburgh, PA, USA.
2.3. Additional fossil chondrichthyan taxa
To facilitate our re-description of Chondrenchelys, we studied additional early chondrichthyan taxa. These include Carboniferous chondrichthyans from the Serpukhovian Bear Gulch Limestone of Montana, USA, in the collections of the Carnegie Museum of Natural History, Pittsburgh, PA, USA, including, Harpagofututor volsellorhinus (Lund Reference Lund1982). In addition, we examined specimens of Helodus (Agassiz Reference Agassiz1838) in the collections of the Natural History Museum, London, UK.
2.4. Systematic palaeontology
Class Chondrichthyes (Huxley Reference Huxley1880)
Subclass Holocephali (Bonaparte Reference Bonaparte1832)
Family Chondrenchelyidae (Berg Reference Berg1940)
Emended diagnosis. Holostylic chondrichthyans distinguished from other holocephalans by their anguilliform body, spineless, elongate dorsal fin, and dentition consisting of large, posterior tooth plates, preceded by smaller subtriangular anterior tooth plates in the upper and lower jaws. Axial skeleton composed of dorsal and ventral couplets of wedge-shaped (in lateral view) chordacentra consisting of non-tesselate calcified cartilage, supporting slender neural and haemal arches, and neural spines with long spines in the caudal region, which occasionally bifurcate. Pre-caudal axial skeleton varies from monospondylous to diplospondylous; ratio of vertebral arches to chordacentra becomes irregular within the caudal region. No synarcual is present. Pectoral fins with well-developed central axis and pre- and post-axial radials branching from close to fin base. Male pelvic fins bear a spatulate myxopterygial cartilage flanked proximally by a large, subtriangular distal radial cartilage.
Genus Chondrenchelys (Traquair Reference Traquair1888b)
Emended diagnosis. Chondrenchelyids distinguished by possession of a single pair of upper and a single pair of lower anterior tooth plates with high ridges, and an extramandibular arcade of small, low-crowned teeth. Cranium with elongated rostral region bearing a distinct trough along the dorsal midline, and widely separated orbits with subtriangular, highly mineralised antorbital crests. Postorbital rim broad and flanks, laterally, the anterior part of the otic capsule. Dorsal fin supported by two (proximal and distal) radial series. Anteriorly, the fin originates with a non-mineralised keel supporting paired denticles along the leading edge. The fin terminates posteriorly, short of the caudal extremity, with the axial skeleton terminating in a slender whip or rat-tail. Pectoral fins are sexually dimorphic. In both sexes, the fins are paddle-like; female pectoral fins are poorly preserved and distinctly smaller than male examples. The larger male pectoral fins have a dibasal articulation with the girdle, hypertrophied radials, claw-like denticles at the fin perimeter, and supported a distal fringe of short ceratotrichia.
Species Chondrenchelys problematica (Traquair Reference Traquair1888b)
Syntypes. GSE 2601 and counterpart GSE 2602, RSM 1885.54.5A and B (part and counterpart), RSM 1886.31.24 and counterpart NHMUK P.4085 (after Lund Reference Lund1982).
Referred specimens. NMS 1998.35.1 (Fig. 3) is a single slab with an individual (female) approximately 185 mm in length. The body is lying on its right side, with the cranium rotated and visible in dorsolateral aspect. The dentition of this specimen was figured by Finarelli and Coates (Reference Finarelli and Coates2012, fig. 2). The axial skeleton is exceptionally complete, and a faint, organic stain is visible for much of the body length, suggesting the outline of soft tissues at deposition. The cranium is approximately 18 mm in rostrocaudal length.

Figure 3 Chondrenchelys problematica, female, NMS 1998.35.1: (a) compound photograph and (b) camera lucida drawing of entire specimen. In the photograph in part (a), the specimen has been whitened with ammonium chloride. In parts (a) and (b), anterior is to the left, with dorsal to the top of the image.
NMS 2002.68.1 (Fig. 4) preserves an individual (male) approximately 140 mm in length in part and counterpart. The anterior portion (pectoral girdle and forward) of NMS 2002.68.1 is preserved in dorsal (part) and ventral (counterpart) views. Caudal to the pectoral girdle, the axial skeleton twists to preserve the body in lateral view. The counterpart, NMS 2002.68.1-cp (Fig. 4a) preserves, uniquely, the upper dentition in oral view, the neurocranium base in ventral view, and portions of the axial skeleton, right scapulocoracoid and distal parts of the left pectoral fin. The part, NMS 2002.68.1-p (Fig. 4b), preserves the mandible and dentition in oral view (figured in part by Finarelli & Coates Reference Finarelli and Coates2012, figs. 1B, 3A; Ginter & Sun Reference Ginter and Sun2007, fig. 8A), portions of the axial skeleton, the majority of the pectoral fins and parts of the pectoral girdle, the pelvic girdle and part of the pelvic fins.

Figure 4 Chondrenchelys problematica, male, NMS 2002.68.1, compound photographs of entire specimen: (a) counterpart, anterior to right; (b) part, anterior to left. Specimen has been whitened with ammonium chloride; in both images, the postcranial skeleton is shown with the dorsal surface towards the top of the figure.
HM V.7173 (Fig. 5), previously identified as the actinopterygian Phanerosteon mirabilie, was referred to Chondrenchelys problematica by Finarelli & Coates (Reference Finarelli and Coates2012). This specimen consists of a single, small slab containing the anterior trunk, pectoral and cranial skeleton. Precise locality information is not available (see: Finarelli & Coates Reference Finarelli and Coates2012), although ostracods in the attached matrix suggest it originated from the Calciferous Sandstone: Lower Limestone Group, and probably from the Glencartholm Fish Bed. HM V.7173 also includes phosphatic concretions suggestive of gut contents.

Figure 5 Chondrenchelys problematica, HM V.7173: photograph of entire specimen. Postcranium revealed in left lateral view; cranium broken to reveal upper dentition in dorsal view; specimen whitened with ammonium chloride. Anterior is to the left, with dorsal to the top of the image.
RSM 1885.54.5A (part), figured by Traquair (Reference Traquair1888a, Reference Traquairb), Moy-Thomas (Reference Moy-Thomas1935), Frickhinger (Reference Frickhinger1991) and Stahl (Reference Stahl and Schultze1999), and B (counterpart), presents an individual with a crushed and coarsely prepared cranium (on the part, 54.5A) and axial skeleton. The dentition is mostly intact, preserving at least three anterior tooth plates (Moy-Thomas Reference Moy-Thomas1935, text-fig. 1).
RMS 1886.31.24, figured by Traquair (Traquair Reference Traquair1888a, Reference Traquairb), displays the cranium figured by Moy-Thomas (Reference Moy-Thomas1935, text-fig. 4). Although in generally poor condition, the counterpart, NHMUK P.4085, includes well-preserved sections of the caudal skeleton.
RMS 1891.53.33, referred to Chondrenchelys and figured by Lund (Reference Lund1982), preserves several identifiable tooth plates, portions of the cranium and anteriormost axial skeleton, including a three-dimensionally intact series of centra, and a moderately complete pectoral fin.
NHMUK P.18054, figured by Moy-Thomas (Reference Moy-Thomas1935, plate II, fig. 2; text-figs. 2, 11; Field number J.A.M.T.2.1933) includes the mandible and dentition in oral view, and parts of the axial skeleton.
NHMUK P.18055 and counterpart NHMUK P.22967, figured by Moy-Thomas (Reference Moy-Thomas1935, plate II, fig. 1; text-fig. 6; Field number J.A.M.T.2.1934) includes well-preserved anterior axial skeleton, cranial outline, pectoral and pelvic skeletons.
NHMUK P.18056 and counterpart NHMUK P.18057, figured by Moy-Thomas (Reference Moy-Thomas1935) includes well-preserved pectoral and pelvic skeletons.
GSE 13328, figured by Lund (Reference Lund1982, fig. 5F), Ginter & Sun (Reference Ginter and Sun2007, fig. 8B) and Finarelli & Coates (Reference Finarelli and Coates2012, fig. 3B) is notable for well-preserved palatal tooth plates.
Diagnosis. As for genus: monotypic.
3. Description of Chondrenchelys problematica
3.1. Skull
We augment the comparative anatomical content of the following description by including images of the cleared and stained braincase and palate of a pre-hatchling elephant shark, Callorhinchus milii, juxtaposed with images of the cranium of Chondrenchelys problematica (Fig. 6). Further sources of chimaeroid cranial morphology are summarised in the introduction to the discussion section.

Figure 6 Comparison of holocephalan crania: extant Callorhinchus millii and the Lower Carboniferous Chondrenchelys problematica (specimen NMS 1998.35.1). Callorhinchus millii, embryonic neurocranium and palate (stage 36, Didier et al. Reference Didier, Leclair and Vanbuskirk1998), cleared and stained (cartilage with methyl blue) shown in (a) lateral, (b) dorsolateral and (c) dorsal aspect; images illuminated via combination of transmitted and reflected light. (d) Chondrenchelys problematica NMS 1998.35.1, specimen whitened with light coating of ammonium chloride; (e) photographic rendering processed via RTI Viewer using specular enhancement option. In parts (d) and (e), the view is of the dorsal skull surface, with anterior is to the left. Abbreviations: adf=dorsal fontanelle; aoc=antorbital crest; asc=anterior semicircular canal; cd=cranial denticles; cqp=cranioquadrate passage; dlof=dorsolateral otic fossa; ds=dorsum sellae; el=external lamina; emt=extramandibular teeth; ep=ethmoid process; esc=external semicircular canal; ethr=ethmoid roof; fa=antotic foramen; fe=endolymphatic foramen; foa=foramen for orbital artery; fm=foramen magnum; hf=hypoglossal foramina; jun=jugal notch; lat=lower anterior tooth plate; lon=lamina orbitonasalis; lpt=lower posterior tooth plate; lrp=lateral rostral process; mc=Meckel's cartilage; mrp=medial rostral process; nc=nasal cartilage; occ=occipital cotylus; oec=opening (posterior) of ethmoid canal; onc=orbitonasal canal; op=otic process; pa=pila antotica; pop=postorbital process; por=postorbital rim; psc=posterior semicircular canal; q=quadrate; rf=rostral foramen of the ethmoid canal; rlc=rostrolabial cartilages; sc=sagittal crest; sor=suborbital ridge; sos=supraorbital shelf; upt=upper posterior tooth plate; II=optic nerve foramen; V=foramen for anterior branch of superficial ophthalmic nerve. Callorhinchus specimen prepared and supplied by Dr Andrew Gillis. Note that rostral processes (mrp, lrp) of C. millii are broken and bent toward the specimen's right side. Cranial cap denticles (cd) are a transitory feature of embryonic chimaeroids, generally shed at or shortly after hatching (Didier et al. Reference Didier, Kemper, McEachran, Carrier, Musick and Heithaus2012).
The Chondrenchelys cranium and mandible are composed of tessellate calcified cartilage. Although the absolute size of the tesserae varies considerably, the patterns of close-packed polygonal mineralisations at cartilage surfaces are indistinguishable from other Palaeozoic chondrichthyans and Recent elasmobranchs (Fig. 7a, b). In modern chimaeroids, tesserae are not obvious at the cartilage surface. Cartilage mineralisation is superficially smooth, although closer inspection reveals a microspherulitic, granular texture (Fig. 7c). Histological studies show that subsurface tesserae are indeed present in chimaeroids (A. Plamen, pers. comm. to MIC, 2012).

Figure 7 Calcified cartilage from three chondrichthyans, showing clearly defined tesserae in (a) the lateral surface of Squalus acanthias Meckel's cartilage and (b) the roof of Chondrenchelys problematica chondrocranium; (c) granular mineralisation forms the lateral surface of Hydrolagus collei Meckel's cartilage. All images are to same scale.
Rostrocaudal length of the cranium in both NMS 1998.35.1 (Figs 6a, b, 8d) and NMS 2002.68.1p (Fig. 8a–c) is approximately 18 mm, the otic roof is approximately 10 mm wide, and the inter-quadrate distance is approximately 17 mm. Moy-Thomas (Reference Moy-Thomas1935) reconstructed a wedge-shaped cranium, with subequal rostral, orbital and postorbital proportions. However, in NMS 1998.35.1 and RSM 1885.54.5, the rostral and orbital regions each occupy approximately 40% of total skull length, with the post-orbital region being correspondingly short. Unlike modern chimaeroids (Fig. 6a–c), where the jaw articulation with the quadrate (q) is positioned at, or anterior to, the level of the antorbital crest (aoc), in Chondrenchelys (Fig. 6d, e), the articulation is just anterior to the level of the postorbital rim (por).

Figure 8 Chondrenchelys problematica: (a) NMS 2002.68.1, cp, showing base of neurocranium in ventral view; (b) NMS 2002.68.1, p, showing the mandible and associated dentition in dorsal (oral) view; (c) part and counterpart mapped on onto each other, showing the degree of overlap; (d) NMS 1998.35.1, neurocranium exposed in dorsolateral aspect. Specimens shown in (a), (b) and (d) whitened with ammonium chloride. Part (d) in this figure is repeated from Figure 6d to provide a complete reference to the Chondrenchelys chondrocranium. Abbreviations: adf=dorsal fontanelle; aoc=antorbital crest; cp=condylar (mandibular) process; ds=dorsum sellae; el=external lamina; emt=extramandibular teeth; ethr=ethmoid roof; fa=antotic foramen; fe=endolymphatic foramen; gf=glenoid fossa; jun=jugal notch; lat=lower anterior tooth plate; lon=lamina orbitonasalis; lpt=lower posterior tooth plate; mc=Meckel's cartilage; mcs=mandibular cartilage shelf; occ=occipital cotylus; pgf=preglenoid lateral flange; pop=postorbital process; por=postorbital rim; psc=posterior semicircular canal; q=quadrate; sos=supraorbital shelf; tdb=transverse dentine bands; upt=upper posterior tooth plate; II=optic nerve foramen; V=foramen for anterior branch of superficial ophthalmic nerve. All images are to same scale.
Jaw suspension in Chondrenchelys is holostylic, like modern chimaeroids, with the palatoquadrate completely fused to the ventral surface of the neurocranium (Moy-Thomas Reference Moy-Thomas1935; Lund Reference Lund1982). In modern adult chimaeroids, large orbits are separated by a narrow septum of connective tissue, and are displaced dorsally relative to the cranial space housing the forebrain. The orbits are similarly large in Chondrenchelys, but widely separated, with substantially mineralised medial walls, such that there is no interorbital septum. In addition, there is no dorsal displacement, with the forebrain lying between the orbits, as in modern elasmobranchs.
The space occupied by the otic labyrinth in Chondrenchelys is substantially smaller than the orbit. There is no indication of “wedging” of the occipital arch between otic capsules (Fig. 6d, e) (Maisey Reference Maisey1983). The otico-occipital roof is subrectangular, with a posterolateral prominence formed by the outermost rim of the posterior semicircular canal (psc). The relation of this prominence to the lateral occipital process of other chondrichthyans (Schaeffer Reference Schaeffer1981) is uncertain. In NMS 1998.35.1, a concavity lies anterior to the ridge overlying the posterior semicircular canal: the dorsolateral otic fossa (dlof: Fig. 6). The anterior boundary of this fossa probably marks the location of the anterior semicircular canal (cf.asc: Fig. 6c). The otic roof bears no trace of a midline sagittal crest (sc) or metotic fissure. The occipital wall, cotylus and arch are well-mineralised and stand in high relief relative to surrounding cartilages and matrix. In contrast, the lateral wall of the otic region is poorly preserved in all specimens. The occipital arch and cotylus complex (occ) encompassing the foramen magnum is posteriorly prominent in all three specimens, also apparent in Harpagofututor (Lund Reference Lund1982, figs 2A, 3A).
The braincase roof between the orbits in NMS 1998.35.1 has collapsed to form a shallow trough, indicative of a broad, underlying, interorbital space. Two midline foramina are present, the margins of which are distinct and show no signs of being the result of breakage due to the collapse of the cranial roof. The elongate (approximately 0.7 mm), anterior foramen is identified as an anterior dorsal fontanelle (adf), and is positioned just anterior to the level of the postorbital process (pop), similar to the dorsal midline opening in the chondrocranial roof of Cladodoides wildungensis (“ms” in Maisey Reference Maisey2005, fig. 4). The small, foramen-like recess immediately posterior to the anterior dorsal fontanelle, might represent the exit of the endolymphatic ducts (fe), although it is substantially smaller than the endolymphatic opening in the similarly sized chondrocranium of Callorhinchus (compare Figs 6c and d).
A large, subtriangular antorbital crest (aoc) forms the anterior boundary of the orbit roof. More substantially calcified than other parts of the braincase, this crest persists as a prominent feature, and serves as a convenient landmark, even in severely-crushed specimens (e.g., RMS 1888.54.5). The absence of such a crest in Harpagofututor (Lund Reference Lund1982, text-fig. 2 and elsewhere) presents a distinctive difference between these otherwise similar genera. Posteriorly, the antorbital crest is continuous with the supraorbital shelf (sos), a concave lateral rim that is also robustly mineralised, and persists in otherwise crushed specimens (e.g., HM V.7173; RSM 1888.54.5).
The roof of the pre-orbital region (ethr) is concave, forming in a midline trough flanked, laterally, by high walls, presumably formed by the lamina orbitonasalis cartilage (lon) (de Beer Reference de Beer1937). In NMS 1998.35.1, the walls of the lamina orbitonasalis remain in position, with the cartilage of the roof of the ethmoid region visible along the midline between them. The position of this trough roughly corresponds to the ethmoid canal of extant chimaeroids, which is an enclosed extracranial space, into which the superficial and deep ophthalmic nerves pass upon exiting the orbit (oec). The ophthalmic nerves pass anteriorly through the canal to the nasal capsule, exiting via the rostral foramen (rf) (de Beer Reference de Beer1937; Didier Reference Didier1995). A large foramen is visible just caudal to the antorbital crest on each side of the neurocranial roof of NMS 1998.35.1. We interpret these as the exit for part of the superficial ophthalmic nerve (V) from onto the dorsal surface of the skull, as in modern elasmobranchs (Goodrich Reference Goodrich1930; Marinelli & Strenger Reference Marinelli and Strenger1959). We argue that there is no ethmoid canal in Chondrenchelys, and that the nerve supply to the rostral sensory array was on the dorsal, external aspect of the chondrocranium, and that the pre-orbital endocranial space in NMS 1998.35.1 enclosed only the olfactory tracts. Moreover, it is also important to note that the roof of the ethmoid region was closed and that there is no evidence of a precerebral fontanelle in Chondrenchelys.
The orbits of Chondrenchelys are substantially larger than previous reconstructions (Moy-Thomas Reference Moy-Thomas1935). The caudal border of the exposed left orbit of RSM 1888.54.5 (Traquair Reference Traquair1888b; Moy-Thomas Reference Moy-Thomas1935) is highly damaged, which lead to their underestimation of the orbit's size and overestimation of post-orbital length. In NMS 1998.35.1, the left orbit postorbital rim is nearly complete and remarkably well-developed. Importantly, the rim extends laterally and posteriorly to form the anterior part of the otic wall, as in modern chimaeroids (Fig. 6d, e, por). We interpret the deep notch near the caudoventral orbit border as a recess for passage of the jugular vein (jun).
Within the orbit, there is a large foramen in the posterior wall, which we interpret as the foramen antoticum (fa) (Fig. 6d, e), observed in similarly sized embryos of Chimaera (de Beer & Moy-Thomas Reference de Beer and Moy-Thomas1935) and Callorhinchus (Fig. 6b, c). The foramen antoticum transmits much of the trigeminofacial nerve complex (nerves V and VII) to the orbit. Close to the anterior base of the orbit in NMS 1998.35.1 there is a large foramen, the size and position of which match the entry of the optic nerve (II) into the orbit (Kesteven Reference Kesteven1932; de Beer & Moy-Thomas Reference de Beer and Moy-Thomas1935). The orbit wall shows no evidence for an optic pedicel. Other openings likely to have been present (e.g. foramina for the pituitary vein, and nerves III, IV and VI) are unknown, and probably obscured through postmortem compression.
The orbit is floored by a cartilage shelf, which likely supported the dental epithelium of the upper posterior tooth plates (upt). This shelf was probably reinforced by the underlying tooth plates (Fig. 9) (Finarelli & Coates Reference Finarelli and Coates2012). In modern chimaeroids, this floor is formed by the palatoquadrate cartilage, and extends posteriorly beyond the level of the postorbital rim (por), meeting the lateral extension of the otic wall to form the otic process (Fig. 6a, b: op) (Grogan et al. Reference Grogan, Lund and Didier1999). Whether this process is present in Chondrenchelys remains uncertain, as all specimens are damaged in this region. The orbit floor is visible in both NMS 1998.35.1 (Figs 6d, e, 8e) and RMS 1885.54.5. Unlike extant chimaeroids, there is no suborbital ridge (sor) forming a distinct lateral boundary to the orbit along its ventral and posterior margin. Rather, in Chondrenchelys, the lateral margin is reinforced by the external lamina (el) (=“descending lamina” of Patterson Reference Patterson1992) of the upper posterior tooth plate (upt) (Finarelli & Coates Reference Finarelli and Coates2012).

Figure 9 Chondrenchelys problematica, the complete dentition: (a) reconstructed as if looking into the open mouth, number of extramandibular teeth estimated; (b) NMS 2002.68.1p, right lower posterior tooth plate, oral surface; (c) BGS-GSE 13328, left upper posterior tooth plate, oral surface; (d) NMS 1998.35.1, anterior dentition, camera lucida drawing; (e) NMS 1998.35.1, extramandibular teeth, coronal view. Abbreviations: emt = extramandibular teeth; lat = lower anterior tooth plate; tdb = transverse dentine bands; uat = upper anterior tooth plate. Tooth plates in (b) and (c) to same scale.
Extant chimaeroids lack an anterior skeletal wall to the orbit (Fig. 6c, d); the anterior orbit boundary is formed by a preorbital fascia, which is reinforced by the anterior belly of the M. adductor mandibularis (Anderson Reference Anderson2008). In Chondrenchelys, the orbit is deeply recessed relative to the preorbital cranial wall, with a marked anterior skeletal border. However, this anterior skeletal wall is not like those observed in other early chondrichthyans, such as Xenacanthus (Schaeffer Reference Schaeffer1981) or Cobelodus (Maisey Reference Maisey2005), in which a skeletal anterior orbit margin is formed by the posterior face of the nasal capsule (which is derived from the lamina orbitonasalis) (de Beer Reference de Beer1937). In modern chimaeroids and Chondrenchelys, the nasal capsule is separated from the orbit by an extended ethmoid region, and the resultant preorbital wall (itself formed by the lamina orbitonasalis) is occupied by M. adductor mandibularis. Due to the configuration of the lamina orbitonasalis cartilage and the median trough of the ethmoid region, we conjecture that the nasal capsules, although not preserved in Chondrenchelys, were well-separated from the orbits.
NMS 2002.68.1cp (Fig. 8a, c) preserves the basicranium in ventral view. A deep, transverse trough separates the orbital and otic regions, in the same position as the osteichthyan ventral otic fissure. We suggest that it reflects retention of a fragile region of cartilage in the adult (Janvier Reference Janvier1996). Immediately anterior to this trough, the basicranial floor collapsed to the interior of the braincase, over a robust ridge of cartilage, highlighting an internal feature that likely marks the location of the dorsum sellae (ds; see also Fig. 6a) (de Beer Reference de Beer1937). Although the cranial floor anterior to the dorsum sellae is indented and fractured, we find no convincing evidence for a hypophyseal opening (Goodrich Reference Goodrich1930); absence of a hypophyseal opening is shared with chimaeroids and iniopterygians (Khonsari et al. Reference Khonsari, Seppala, Pradel, Dutel, Clement, Lebedev, Ghafoor, Rothova, Tucker, Maisey, Fan, Kawasaki, Ohazama, Tafforeau, Franco, Helms, Haycraft, David, Janvier, Cobourne and Sharpe2013), and contrasts with other early chondrichthyans (Coates & Sequeira Reference Coates and Sequeira1998; Maisey Reference Maisey2005, Reference Maisey2007). The broken rim of the upper posterior tooth plate is visible on the left and right sides of the palate (Fig. 8a), and much of the area covered by this tooth plate is shown in Figure 9a and c.
The ventral surface of the occipital region is incompletely known. Although much of the basicranial surface is preserved in NMS 2002.68.1cp, mapping the part onto the counterpart reveals a rim of poorly preserved cartilage embedded within the matrix behind this lower jaw, suggesting that the complete ventral extent of the occipital region in NMS 2002.68.1p remains concealed in the matrix. It is not possible to determine whether this cartilage includes a canal for the dorsal aorta. No such canal is present in modern chimaeroids, yet an anterioposteriorly short and ventrally prominent aortic canal is present in Helodus (Patterson Reference Patterson1965; pers. obs. JAF & MIC: NHMUK P.8212). Other basicranial openings in Chondrenchelys are difficult to identify because of the likely size similarity with the calcified tesserae and post mortem compression. However, there is no evidence for a cranioquadrate passage in NMS 2002.68.1, consistent with NMS 1998.35.1.
The lateral surface of Meckel's cartilage is most visible in NMS 1998.35.1, although the lateral profile of the mandible is best observed in NHMUK P.18055. In NMS 1998.35.1, the articular portion has been deformed nearly 90 degrees relative to the corpus of the jaw, such that the articular surfaces are directed medially and obscured. NMS 2002.68.1p provides the first undistorted view of these surfaces (Fig. 8b). As in extant chondrichthyans, the mandible of Chondrenchelys possesses a two-part jaw articulation, with a well-formed lateral glenoid fossa (gf) flanking a medial condylar process (cp) couplet. This contrasts with much less distinct fossa-condyle couplets observed in iniopterygians (Pradel Reference Pradel2010) and in Helodus (pers. obs. JAF & MIC: NHMUK P.8212). As in extant chimaeroids, the articular surface of Chondrenchelys is located on the rearmost extremity of Meckel's cartilage (mc), oriented in a principally anteroposterior direction. There is no retroarticular process or flange on the mandible, and no offset between the two articular surfaces: the glenoid is situated immediately lateral to the condyle. In contrast, we note that in Hydrolagus, the glenoid is displaced anteriorly relative to the condyle. In addition, the two halves of the lower jaw are united firmly (presumably fused) across the symphysis. No otherwise compressed, distorted or broken examples of Chondrenchelys show isolated halves of the complete mandible.
Much of Meckel's cartilage is concealed beneath the prominent lower posterior tooth plate (lpt) (Finarelli & Coates Reference Finarelli and Coates2012). However, NMS 2002.68.1 shows that the ventral-most part of the jaw is curved medially, to form an incomplete shelf or floor to the intermandibular area (Fig. 8b). In dorsal view, this shelf (mcs) bears a series of laterally-oriented, elliptical depressions arranged in an anteriorposterior series. These depressions coincide with the major transverse dentine bands (tdb) of the tooth plate, and this registration between tooth plate banding and skeletal features in the jaw suggests coordinated, episodic dentine accretion and cartilage growth (see: Finarelli & Coates Reference Finarelli and Coates2012). A flange (pgf) projects from the lateral face of Meckel's cartilage below the posteriormost third of the external lamina (el) of the lower posterior tooth plate. This feature is visible in NMS 1998.35.1 and NMS 2002.68.1, and might be equivalent to a pre-glenoid lateral flange in Hydrolagus (pers. obs. JAF & MIC). A similarly-positioned ventrolateral flange is clearly present in Helodus (pers. obs. JAF & MIC: NHMUK P.8212), and is possibly also present in Iniopera (Pradel Reference Pradel2010).
The dentition of Chondrenchelys is described in detail by Finarelli & Coates (Reference Finarelli and Coates2012). Here (Fig. 9a), we figure the complete dentition, reconstructed as if looking into the open mouth (following the convention employed by Patterson Reference Patterson1965; Stahl Reference Stahl and Schultze1999; Stahl & Hansen Reference Stahl and Hansen2000; Stahl & Parris Reference Stahl and Parris2004). Each quadrant of the jaws bears a large posterior tooth plate (Fig. 9a–c) and a smaller anterior tooth plate (Fig. 9a, d). Chondrenchelys is further distinguished by possessing an extramandibular dentition (emt), consisting of small teeth arranged around the anterior of the gape that bear striking resemblances to isolated teeth referred to petalodonts (Fig. 9a, d, e). The skeletal support of these extramandibular teeth remains uncertain, although it is possible that rostrolabial cartilages (rlc) were involved (c.f. Callorhinchus, Fig. 6b, c).
3.2. Branchial arches
The branchial cartilages (bc) of NMS 1998.35.1 (Fig. 10a–c) are preserved in a restricted space between the rear of the skull and the scapulocoracoid. The identity of individual arch components in NMS 1998.35.1 is unclear, as they are disarticulated. HM V.7173 also preserves traces of arches, but these remain mostly covered in matrix. Given the proximity of the scapulocoracoid to the caudal margin of the skull, the branchial basket almost assuredly contacted the pectoral girdle, although it is significant that in both specimens the branchial arches are positioned, at least in part, behind the caudal skull margin, and are not completely “subterbranchialian,” like extant chimaeroids (Zangerl Reference Zangerl1973, Reference Zangerl1997; Didier Reference Didier1995).

Figure 10 Chondrenchelys problematica, branchial skeleton and pectoral girdle: (a) NMS 1998.35.1; (b) camera lucida drawing showing branchial skeleton visible between the occiput and pectoral girdle; (c) NMS 1998.35.1 with low angle illumination, white chevrons indicating radiating cartilage ridges; (d) HM V.7173, showing scapulocoracoid in lateral view; (e) camera lucida drawing, with female-pattern pectoral fin outline included, although the fin is barely visible in specimen photograph. Specimens in (a) and (d) whitened with ammonium chloride. Abbreviations: art=articular area for pectoral fin; bc=branchial arch cartilage; cc=chordacentrum; cp=coracoid plate; mpt=metapterygium; na=neural arch; por=postaxial radial; prr=preaxial radial; r=cartilage ray; spr=scapular process.
The most robust of the branchial cartilages are subrectangular and overlain by gracile rods composed of bead-like tesserae, which are similar in form to the dorsal fin radials (see below). These cartilaginous rays (r) are variably-oriented, and in some cases are curved, which suggests that they were flexible (Fig. 10a–c). In NMS 1998.35.1, several rays cross the anterior margin of the scapulocoracoid, and low-angle illumination highlights a series of ridges and rays extending posteriorly from the rear of the gill chamber. The structures might represent either continuation of the gill skeleton, branchial rays, or cartilaginous supports for an opercular flap. Branchial rays are absent in modern adult chimaeroids, but are clearly present in juveniles (Gillis et al. Reference Gillis, Rawlinson, Bell, Lyon, Baker and Shubin2011).
3.3. Pectoral girdle and fins
Most of the information on the pectoral girdle is obtained from NMS 1998.35.1 (Fig. 10a–c) and HM V.7173 (Fig. 10d, e). The girdle in NMS 2002.68.1 is incomplete and compressed dorsoventrally, and the specimens used in Moy-Thomas's (Reference Moy-Thomas1935) description are, as he acknowledged, in generally poor condition.
NMS 1998.35.1 and HM V.7173 preserve the girdle in lateral view, with the left scapulocoracoid overlying the right. Three or four vertebral segments are visible between the occiput and the anterior edge of the scapular process. Previous reconstructions proposed a short coracoid plate relative to the length of the scapular process (Moy-Thomas Reference Moy-Thomas1935; Patterson Reference Patterson1965). However, in NMS 1998.35.1 and HM V.7173, the coracoid plate (cp) and scapular process (spr) are subequal in length. The scapular process is oriented nearly orthogonal to the axial skeleton until the level of the fin articulation (art), below which the coracoid plate is directed anteriorly, forming a near right angle in lateral view (most clearly visible in HM V.7173, Fig. 10d, e).
The lateral face of the scapular process in Chondrenchelys is mostly smooth (Fig. 10a, b). Significantly, there is no trace of the dorsoventrally oriented ridge that is present in modern chimaeroids, providing insertion sites for a suite of muscles, including the cucullaris superficialis and the retractor mesoventralis pectoralis. The fin articulation faces posteriorly and is positioned low on the flank, contra previous reconstructions (Moy-Thomas Reference Moy-Thomas1935; Patterson Reference Patterson1965). Although we find no evidence for a distinct ‘backwardly-directed knob’ on the shoulder girdle (Moy-Thomas Reference Moy-Thomas1935), the articular region is thicker and more prominent than adjacent areas of cartilage.
In NMS 1998.35.1, the preservation of the pectoral girdle gives the appearance of a forward projection under the occipital region, resembling modern and fossil chimaeroids (Patterson Reference Patterson1965; Didier Reference Didier1995) and iniopterygians (Zangerl Reference Zangerl1973, Reference Zangerl1997; Pradel et al. Reference Pradel, Tafforeau and Janvier2010). However, post-mortem deformation has translated the cranium in NMS 1998.35.1 such that it now lies caudally and ventrally to its in-life position. HM V.7173 (Figs 5, 10d, e) more accurately preserves the relative in-life positions of the cranium and pectoral girdle. In this specimen, the pectoral girdle does not contact the occipital region, and the branchial region is more substantially posterior to the skull (as in elasmobranchs, but unlike chimaeroids). A small portion of the forward-projecting coracoid plate is also preserved in NMS 2002.68.1p, and is visible anterior to the large dislocated occipital cartilage (see above). As such, the coracoid does reach the occiput and projects slightly forward of the caudal margin of the cranium; however, not to the extent observed in iniopterygians (Zangerl Reference Zangerl1973, Reference Zangerl1997). In life, the left and right coracoids formed a broad, flat, near-horizontal plate, flooring the branchial chamber.
Specimens of Harpagofututor (e.g., CMNH 27326) confirm Lund's (Reference Lund1982) reconstruction of a scapulocoracoid with near-equal proportions of the scapular and coracoid processes in that taxon as well. Additionally, the coracoid plate probably projected anteriorly under the gill basket, as in Chondrenchelys. We also note a general similarity to the scapulacoracoid of the enigmatic Bear Gulch chondrichthyan Squatinactis caudispinatis (Lund & Zangerl Reference Lund and Zangerl1974), which displays the sharp anterior re-direction of the coracoid under the gill basket. Comparisons with other early chondrichthyans (Stahl Reference Stahl and Schultze1999; Coates & Gess Reference Coates and Gess2007; Lane & Maisey Reference Lane and Maisey2009) suggest that the shape of pectoral girdle in Chondrenchelys and Harpagofututor represents a shared character. Like other early holocephalans, the chondrenchelyid coracoid region lacks a pronounced anterior shoulder, there is no diazonal foramen in lateral view, and the apex of the scapular process has no more than a weakly developed posterolateral angle. It should be noted that these features are also observed in iniopterygians (Pradel et al. Reference Pradel, Tafforeau and Janvier2010)
In NMS 1998.35.1 (Fig. 3) and HM V.7173 (Fig. 10e), the pectoral fins are poorly preserved. In NMS 1998.35.1, remains of left and right pectoral fins are superposed, and include a few stout, axial cartilages and narrower radials along the dorsal and perhaps the postaxial side, with pre-axial radials appearing broader. This pattern is also observed in the slightly better preserved left pectoral fin of HMV.7173 (Fig. 10e), in which a broad central axial cartilage supports thin radials. Notably, these fins are similar to the pectorals of Harpagofututor. Lund (Reference Lund1982) first observed that although this morphotype is present in both males and females of Harpagofututor, in Chondrenchelys it is restricted to females (as determined by the dimorphic pelvic fins). The Mumbie Quarry specimens support Lund's argument: we observe the hypertrophied, paddle-like pectoral fin morphology described by Moy-Thomas (Reference Moy-Thomas1935) only in male Chondrenchelys specimens.
The classic restoration of the male pectoral fin (Moy-Thomas Reference Moy-Thomas1935; reproduced repeatedly: e.g., Patterson Reference Patterson1965; Stahl Reference Stahl and Schultze1999) was compiled from specimens NHMUK P.18056 and NHMUK P.18057 (part and counterpart of the same fin, from the same individual) and NHMUK P.18055. We complement these data with male-pattern left (Fig. 11a–c) and right (Fig. 11a, d, e) pectoral fins from NMS 2002.68.1, and an incomplete left fin from NMS 1891.35.33. Combining data from the left and right fins from NMS 2002.68.1 permits reconstruction of a near-complete articulated fin skeleton (Fig. 11f). This new reconstruction closely resembles Moy-Thomas's (Reference Moy-Thomas1935) estimate, but several substantial differences deserve attention.

Figure 11 Chondrenchelys problematica, male-pattern pectoral fins from NMS 2002.68.1: (a) NMS 2002.68.1p, with parts of NMS 2002.68.1cp superposed to create a composite image of the pectoral skeleton in dorsal view; anterior at top of page. Boxed area of right pectoral fin encloses propterygium; inset image shows higher magnification image of propterygium and adjoining coracoid region (matrix masked to highlight fin and girdle cartilage); (b) left pectoral fin from NMS 2002.68.1p, distal at upper margin, leading edge at right margin; (c) drawings of left pectoral fin skeleton from part and counterpart; (d) Right pectoral fin from NMS 2002.68.1p, distal at upper margin, reversed to show leading edge at right margin; (e) drawings of right pectoral fin skeleton from part and counterpart; (f) combined fin skeletons of left and right pectoral fins; (g) restoration of complete pectoral fin skeleton. All specimens whitened with ammonium chloride except for inset image of propterygium. Abbreviations: cp=coracoid plate; ctr=ceratotrichia; dnt=denticles; mpt=metapterygium; por=postaxial radials; prpt=propterygium; prr=preaxial radials.
Most importantly, we find that the pectoral fin in Chondrenchelys is dibasal, resembling other holocephalans (see: Stahl Reference Stahl and Schultze1999), not monobasal as in previous reconstructions (Moy-Thomas Reference Moy-Thomas1935; Lund Reference Lund1982). The combined part and counterpart of NMS 2002.68.1 (Fig. 11a) clearly shows two cartilages articulating with the scapulocoracoid, in both the left and right fins. For the sake of convention, we refer to the leading-edge articulating cartilage as the propterygium (prpt) and the posterior cartilage as the metapterygium (mpt). The metapterygium corresponds to the most proximal cartilage in the monobasal axis of Moy-Thomas's (Reference Moy-Thomas1935) reconstruction. The propterygium occurs in conjunction with the metapterygium in the left fin of NMS 2002.68.1 (Fig. 11b, c), but it is more completely preserved in the right fin (Fig. 11d, e) in which the metapterygium is incomplete. The propterygium is L-shaped (inset box in Fig. 11a), bearing a process that forms the most proximal part of the fin's leading edge (Fig. 11f, g). At least two radials articulate within the notch of this ‘L’, although when camera lucida copies from all fins are superimposed (Fig. 11f) there appears to be room for three. Thus far, there is no evidence of a propterygium in the female pectoral fin skeleton of Chondrenchelys, in agreement with Lund (Reference Lund1982).
The main axis of the pectoral fin is formed by three robust cartilages. The metapterygium is the most proximal of these, and is about half the proximodistal length of the second segment. The third, distalmost segment is the smallest and is variable in shape; it is squat in NMS 2002.68 but elongate in NHMUK P.18056. The arrangement of radials extending outward from this axis, toward the leading fin edge is conserved in all examples, and is more regular than previously reconstructed. Pre-axial radials consist of a short, subcylindrical, proximal segment, a longer and spatulate mid segment with a proximal, mineralised shaft flattening distally to a truncated, lozenge-shaped profile, and finally a short distal radial terminating the series. The pre-axial series includes approximately nine radials in total (Fig. 11f, g).
The distal portion of the fin is supported by three or four radials extending from the third axial segment. Each radial is composed of simple, subcylindrical segments. The total number and pattern of post-axial radials is less certain (Fig. 11a–c, f). They are generally larger and more irregularly shaped than their pre-axial counterparts, and form a cartilage pavement much as in the pectoral post-axial fin skeleton in modern Chimaera (Stahl Reference Stahl and Schultze1999).
Claw-like denticles are arrayed along the leading edge of the male-pattern fin (Figs 11, 12). As far as we can determine, denticles are not present on the female-pattern fin. Moy-Thomas (Reference Moy-Thomas1935) described these denticles as attached to distal cartilages, tipping the pectoral fin rays and embedded within the fin edge. Lund (Reference Lund1982) reinterpreted these denticles as scales associates with the lateral line. Lund is mistaken, as these denticles are distinct, in both position and morphology, from the lateral line in NMS 2002.68.1. Individual denticles bear a single, pointed crown on a subcircular and proximally concave based (Fig. 12). Denticle crowns are slightly curved, so that the convex surface seems to face the distal part of the fin. Most examples are buried in matrix, but a single denticle presents its concave surface, showing the span of the crown with an almost fingernail-like morphology above a slightly constricted waist (Fig. 12). These fin denticles form a paired series, with one set along the dorsal surface of the leading edge, and a matching series along its ventral surface. Denticle bases are positioned proximal to the fin rim, close to the distal extremity of the endoskeletal radials. Unusually, for fossil material, the full extent of the fin beyond the endoskeleton can be inferred from traces of ceratotrichia (Fig. 11e, f). These are preserved mostly on the right pectoral fin of NMS 2002.68.1p, with traces overlapping the terminal radial cartilages and indicating that the fin is markedly plesodic.

Figure 12 Chondrenchelys problematica, male pectoral fin denticles from left side, NMS 2002.68.1p. Image masked to highlight denticles against surrounding matrix and adjacent cartilages. Anterior (proximal) to right; leading edge towards upper margin.
Finally, we note that the male pectoral fin in Chondrenchelys is proportionately larger than estimated by Moy-Thomas (Reference Moy-Thomas1935) and the pectoral fins in Harpagofututor. The maximum length of the endoskeleton, measured from the proximal articulation to distal tip is almost 25% longer than the mandible, implying that the pectoral fin area is approximately the size of the entire head in lateral view. In comparison, the pectoral fin of a male Harpagofututor is approximately 20% shorter than the lower jaw.
3.4. Pelvic girdle and fins
The most complete male pelvic skeletons are those of NHMUK P.18055 and NHMUK P.18057 (Fig. 13a), with additional data from NMS 2002.68.1 (Fig. 13b). Our restoration of the male girdle and fin is in broad agreement with Moy-Thomas (Reference Moy-Thomas1935, text-fig. 9) and comments by Lund (Reference Lund1982). The well-preserved pelvic girdle (pg) in NMS 2002.68.1 is simple, as in Harpagofututor, narrow anteriorly and broad posteriorly, with two articulation surfaces for a dibasal fin. Thus, the pelvic fin in Chondrenchelys is not polybasal, as Lund (Reference Lund1982) suggested for Harpagofututor. The anterior, propterygial pelvic radial (prpt) is small, supporting a single distal radial. The posterior proximal radial is a large basipterygium (bpt), with a stepped or faceted ventral/lateral edge supporting six or seven distal radials. About five shorter and broad intermediate cartilages (intc) articulate in line between the caudal or distal end of the basipterygium and the proximal end of the long myxopterygial cartilage (mxpt), and further distal radials (dra) articulate with these intermediate cartilages. These are more distinctive, morphologically, than anterior pelvic radials, possibly contributing to Moy-Thomas's disputed restoration of a pelvic fin with twin axes (see: Lund Reference Lund1982). The proximal part of the myxopterygium is flanked posteriorly by a narrow, triangular, cartilage that appears to be the most posterior member of the distal radial series, a feature that is also observed in Harpagofututor (Lund Reference Lund1982, text-fig. 3).

Figure 13 Chondrenchelys problematica, male pelvic fin: (a) NMHUK P.18057, camera lucida drawing from original specimen, showing left and right fins in ventral view with anterior to the left. Gray shaded areas probably represent denticle bases; (b) NMS 2002.68.1p, girdle and fin, with many of the cartilaginous radials obscured by large denticles (specimen coated with ammonium chloride). Specimen is in latero-ventral view with anterior to the left; (c) NMS 2002.68.1p, detail of radial tips with terminal denticles (matrix masked to highlight skeletal material). Distal is to the bottom of the image; (d) reconstruction of pelvic girdle and fin skeleton. Abbreviations: bpt=basipterygium; dra=distal radials; dnt=denticles; intc=intermediate cartilages; mxpt=myxopterygium; pg=pelvic girdle; prpt=pelvic propterygium.
The full complexity of the pelvic claspers remains unclear. There is no evidence for a pre-pelvic tenaculum in any of the new Chondrenchelys specimens. Pelvic fin cartilages in NMS 2002.68.1 are more poorly preserved than in NHMUK P.18057; however, they do preserve a series of large denticles (Fig. 13b, dnt) arrayed at the tips of the distal radials (Fig. 13c) and at the distal end of the myxopterygium, similar to male specimens of Harpagofututor (Lund Reference Lund1982). NHMUK P.18057 shows a further series of small objects associated with the intermediate cartilages (shaded grey in Fig. 13a), whose exposed surfaces suggest a different histology, and which might be the bases of additional denticles aligned with the main axis of the male pelvic fin. A restoration of the pelvic skeleton, without denticles, is shown in Figure 13d.
Lund (Reference Lund1982) identified the first specimen of a female pelvic skeleton in NMS 1885.54.5, describing it as tiny and splint-like, with about seven fine radials. A second example of a female pelvic skeleton is observed in NMS 1998.35.1 (Fig. 3), although the remains of this fin are limited to a small cartilage, approximately 5 mm in length, ventral to the vertebral column and just anterior to the haemal arch series. The anterior end of the cartilage is incomplete, but the posterior portion bears a series of fine radials composed of small bead-like cartilages, again similar in composition to the midline fin radials (see below). These extend along the medial edge of the primary cartilage, and the relative size of these elements agrees with the description of female pelvic fins in Harpagofututor (Lund Reference Lund1982).
3.5. Vertebral column
The axial skeletons of Chondrenchelys and Harpagofututor are remarkably similar (Fig. 14a, b). Both taxa possess neural arches (na) and spines (nsp), heamal arches (ha) and spines (hsp), and mineralised central elements that enclose the notochordal space. In agreement with Patterson (Reference Patterson1965; see also: Didier Reference Didier1995; Stahl Reference Stahl and Schultze1999) we regard these mineralisations as chordacentra (chc) (contra Lund Reference Lund1982, who interpreted these as arcualia). Each chordacentrum appears to be composed of calcified cartilage (c.f. Moy-Thomas Reference Moy-Thomas1935). In living chimaeroids, chordacentra form complete mineralised rings within the notochordal sheath, are closely packed, and are anteroposteriorly short (Goodrich Reference Goodrich1930; Patterson Reference Patterson1965; Didier Reference Didier1995; Stahl Reference Stahl and Schultze1999). In contrast, the chordacentra of Chondrenchelys and Harpagofututor are much longer, anteroposteriorly. Most of the chordacentra are broken and/or displaced, but short coherent sequences are preserved in place. For Chondrenchelys, examples include specimens NMS 1891.53.33 (Fig. 14c) and NMS 1998.35 (Fig. 3b); and for Harpagofututor, specimens CM 272326 (Fig. 14b) and CM 41073. These show that each chordocentral segment is composed of distinct dorsal and ventral, thin walled half-rings (hemicentra), at least within the trunk region. Each hemicentrum is wedge-shaped in lateral aspect (Fig. 14c), although there is regional differentiation in form. The thin walled profile of these units is shown well in a series of collapsed centra facing laterally within the trunk region of NMS 1998.35 (Fig. 14d). Each centrum bears a small, sub-triangular boss (sb) in the mid–ventral region, the significance of which is unknown. Within the caudal series, these hemicentra are more irregularly shaped (see also: Moy-Thomas Reference Moy-Thomas1935). NMS 1891.53.33 preserves lozenge-shaped gaps between adjacent chordocentra pairs (Fig. 14c, icg), visible in lateral view. In Chondrenchelys, the chordacentra are not tightly packed as in extant chimaeroids. Lund's (Reference Lund1982) reconstruction of Harpagofututor shows very widely-spaced centra. This differs from our observations of CMNH 27326 (Fig. 14b), which more closely resemble Chondrenchelys.

Figure 14 Chondrenchelyid axial skeletons: (a) Chondrenchelys problematica NMS 1998.35, detail of anterior caudal region showing extremities of neural and heamal spines, and anastomising neural spines; (b) Harpagofututor volsellorhinus CM 27326, detail of anterior caudal region; (c) Chondrenchelys problematica NMS 1891.53.33, intact series of chordacentra forming a cylindrical axial column; (d) Chondrenchelys problematica NMS 1998.35 detail of trunk chordacentra; (e) Chondrenchelys problematica NMS 1885.54.5A, detail of caudal skeleton showing bifurcating neural spine and dorsal fin radials. Anterior to left of page; images in (b) and (e) reversed. Abbreviations: ans=anastomosed neural spines; bf=bifurcated neural spine; chc=chordacentrum; dfr=dorsal fin radial; ha=haemal arch; hsp=haemal spine; icg=inter-central gap; na=neural arch; nsp=neural spine; sb=subtriangular boss.
The register of centra (each consisting of a chordacentral pair) to the neural, and posteriorly haemal, arches varies throughout the axial skeleton of Chondrenchelys. A simple segmental arrangement of complete vertebral units (one centrum to one arch and spine) appears to be present in the pectoral region, and possibly persists through the entire pre-pelvic region (Fig. 3), but it is difficult to find any region of the axial skeleton that is consistently mono- or diplospondylous. It is noteworthy that in living chimaeroids, the ratio of arches to centra is fundamentally irregular throughout the vertebral column (Didier Reference Didier1995).
The axial skeleton is substantially longer than previously appreciated (compare Figs 1, 3 and 4). Moy Thomas's (1935) reconstruction produced a pectoral–pelvic length relative to total axial column length ratio of 1:2.5 (using specimens now known to be males). Here, NMS 1998.35.1 (female) has ratio of 1:3, and NMS 2002.68.1 (male) is proportionally (though not absolutely) longer, at 1:3.25. Characterising the axial skeleton in terms of vertebral counts is difficult. Both Moy-Thomas (Reference Moy-Thomas1935) and Patterson (Reference Patterson1965) estimated approximately one hundred vertebrae in Chondrenchelys. Lund (Reference Lund1982) was more cautious, noting that in Harpagofututor it is “impossible to obtain accurate counts past the midcaudal part of the tail.” We counted precaudal neural arches, using each arch as a proxy for a “vertebral segment.” Defining the start of the caudal region at the first haemal arch (Sallan Reference Sallan2012), in NMS 1998.35 (large female: Fig. 3), there are at least 36 pre-caudal vertebral segments. In NMS 1885.54.5A (small female) and NMS 2002.68.1 (large male: Fig. 4), the counts are around 35 segments. These counts are substantially larger than Moy-Thomas's (Reference Moy-Thomas1935) reconstruction (26 segments), and are more compatible with Lund's (Reference Lund1982) restoration of Harpagofututor (32 pre-caudal vertebrae) and modern Rhinochimaera (34 pre-caudal segments) (Patterson Reference Patterson1965, fig. 1).
Neural and heamal arch morphology conforms to previous descriptions (Moy-Thomas Reference Moy-Thomas1935; Patterson Reference Patterson1965). In contrast to the chordacentra, to which their bases are firmly attached, the arches consist of what might be described as tessellate calcified cartilage, at least proximally. The anteriormost cervical series, including those overlapped by the scapular process, consists of about five segments. These cervical arches in addition to the following five or six arches are anteroposteriorly broad (Moy-Thomas Reference Moy-Thomas1935). Caudal to these, neural arches and spines narrow rapidly and are preserved as delicate chains of bead-like calcifications (Moy-Thomas Reference Moy-Thomas1935; Stahl Reference Stahl and Schultze1999). In NMS 1998.35, these beads are slightly spool-shaped, so that the spines are, in effect, segmented (Fig. 14a, d).
As preserved, many of these arches and spines exhibit a sinuous curvature (Fig. 14a) reminiscent of fossil crinoid appendages, suggesting a high degree of flexibility in life. Functionally, this would be highly unusual for a vertebrate; the straighter vertebral arches and spines of Harpagofututor (Fig. 14b) are far more conventional in this respect. A further unusual feature of the axial skeleton, first noted in Chondrenchelys by Moy-Thomas (Reference Moy-Thomas1935) and subsequently reported in Harpagofututor (Lund Reference Lund1982), is that some of the heamal spines bifurcate distally. We confirm this, and also note bifurcations in caudal neural spines as well, e.g. NMS 1885.54.5a (Fig. 14e, b, f), although the occurrence of these bifurcations is rare along the axial skeleton. Finally, Chondrenchelys specimens NHMUK P.4085 and NMS 1998.35.1 display a further apomorphic characteristic: irregularly spaced anastomoses between distal parts of adjacent members of the neural spine series and haemal spine series, (Fig. 14a, ans).
3.6. The midline fin
The dorsal fin is most completely preserved in NMS 1998.35.1 (Fig. 3). Gracile radials extend from a level above the neural spines, starting at approximately the scapulocoracoid and continuing caudally. However, they do not reach the posterior distal extremity (see below). These radials are well preserved in NMS 1998.35.1 (Fig. 3) and HM V.7173 (Fig. 5). In NMS 2002.68.1 (Fig. 4), the midline fin radials are only preserved in the more caudal portion of the body. Radials (dfr) consist of a single column of bead-like or spool-shaped calcifications, which resemble the adjacent distal extremities of the neural spines. Throughout most of the fin, such radials are arranged in proximal and distal rows, and several fin radials correspond to each neural arch/spine segment. In the anterior part of the fin, around four radials intercalate between each neural spine (c.f. Moy-Thomas Reference Moy-Thomas1935), whereas in the posterior trunk and caudal region this ratio decreases (Fig. 14e). Moy-Thomas illustrated a series of robust, anteroposteriorly broad radials of the dorsal fin of male specimens, forming a robust fan in the cervical and pectoral region of the axial column (Moy-Thomas Reference Moy-Thomas1935, plate 2, fig. 1; easily confused with the broad, anteriormost neural spines). Re-examination of NMS 1891.53.33 reveals this is actually a preservational artifact and that that these radials are actually the hypertrophied radials of a subjacent male pectoral fin.
Importantly, in all examined specimens, there is no evidence for a series of radials beyond the haemal spines. As such, and in agreement with Lund (Reference Lund1982), we note that Chondrenchelys, like Harpagututor, lacks a hypocaudal fin. Previous reconstructions (Moy-Thomas Reference Moy-Thomas1935, reproduced in both Patterson (Reference Patterson1965) and Stahl (Reference Stahl and Schultze1999)) show arches/spines and a series of radials supporting both the dorsal and ventral body walls, with a separate dorsal and ventral midline fins reconstructed externally to all of these skeletal structures (see Fig. 1). In fact, the dorsal body wall is supported by the neural arches/spines, with the midline fin supported by the radials and, in the more posterior parts of the fin, by the distal extremities of the neural spines that extend outwards as far as the tips of the radials. In addition, the dorsal fin, as indicated by the caudalmost extent of the neural radials and spines (NMS 1998.35.1, NMS 2002.68.1), terminates short of the distalmost extremity of the axial skeleton by 25–30% of the post-pelvic axial column length, giving Chondrenchelys a distinctive “rat tail”. This is only evident only in better-preserved and prepared specimens (Figs 3, 4, 16). The unusual extent of the dorsal fin, in conjunction with no ventral midline fin, is reminiscent of the extant aba aba, Gymnarchus niloticus.
3.7. Squamation
Modern adult chimaeroids lack scales covering the body, and, in adults the squamation is mostly restricted to areas of the clasping organs in males, and a series of specialised, crescent-shaped denticles supporting the lateral line canals in both sexes (Didier Reference Didier1995). Previous descriptions, as well as the current work, highlight similarly minimal scale coverage for Chondrenchelys (Moy-Thomas Reference Moy-Thomas1935; Lund Reference Lund1982). Those scales that are present are restricted to specific structures, such as the extremities of the paired fins in males (Figs 11–13).
The paths of sensory canals are not well preserved in Chondrenchelys, but a short series of anteriormost main lateral line scales is preserved on the right side of the pre-pectoral area in NMS 1998.35.1 (Fig. 15a, ll), a female. These scales run from the posterolateral prominence of the neurocranial roof to the area dorsal to the scapular process. Lateral line scales are also visible, but very poorly preserve in NMS 2002.68.1, a male. All are broken and preserved as fragments overlying the chordacentra. The shape of these lateral line scales is difficult to determine, but each example appears to be anteroposteriorly elongate, forming a short gutter.

Figure 15 Chondrenchelys problematica, scales: (a) NMS 1998.35.1, area between occipital region and pectoral girdle, showing lateral line scales; (b) HM V.7173, showing pre-dorsal fin keel; (c) and (d) dark stain flanked by simple scale. Anterior to left of page; specimen in (a) coated with ammonium chloride and contrast enhanced to highlight lateral line scales. Abbreviations: bc=branchial cartilages; ll=lateral line scales; occ=occipital cotylus; pdk=pre-dorsal fin keel; spr=scapular process of pectoral girdle; asterisks mark scales aligned with perimeter of dorsal fin.
A further and previously unreported series of scales is associated with the dorsal fin. Thus far, these are exposed uniquely on HM V.7173 (Fig. 15b–d). In this female Chondrenchelys specimen, a keel-like area of dark material slopes dorsally and posteriorly from the occipital region. Note that in Figure 5, this dark film of material is mostly obscured by ammonium chloride sublimate. In Figure 15b, the leading edge of this pre-dorsal keel (pdk) is gently convex, the trailing edge is distinctly concave and slightly mineralised, and the apex continues posteriorly as a narrow, dark belt, dorsal to the neural spines and radials. Simple, flattened scales flank this dark strip. Each scale (examples marked by an asterisk in Fig. 15b–d) is about twice as long as wide, with a pointed, spearhead shape; these are morphologically distinct from lateral line scales. These scales are broadly similar to those associated with the male tenaculum preserved in Harpagofututor (e.g., CMNH 27326), but they do not appear to be recurved in Chondrenchelys.
Ornamentation, in the form of specialised scales and other tenaculum-like structures on the head, appears to be common a feature among stem holocephalans: Harpagofututor (Lund Reference Lund1982), Harpacanthus, (Lund & Grogan Reference Lund, Grogan, Arratia, Wilson and Cloutier2004), and among chondrichthyans more generally, including Falcatus (Lund Reference Lund1985), Damocles (Lund Reference Lund1986) and Akmonistion (Coates & Sequeira Reference Coates and Sequeira2001). Notably, embryonic and hatchling chimaeroids possess scales on the dorsal surface of the head and trunk (Didier et al. Reference Didier, Kemper, McEachran, Carrier, Musick and Heithaus2012), seen here in Callorhinchus (Fig. 6a, c). Such specialised and regionally-restricted squamation might represent a plesiomorphic condition for the Holocephali.
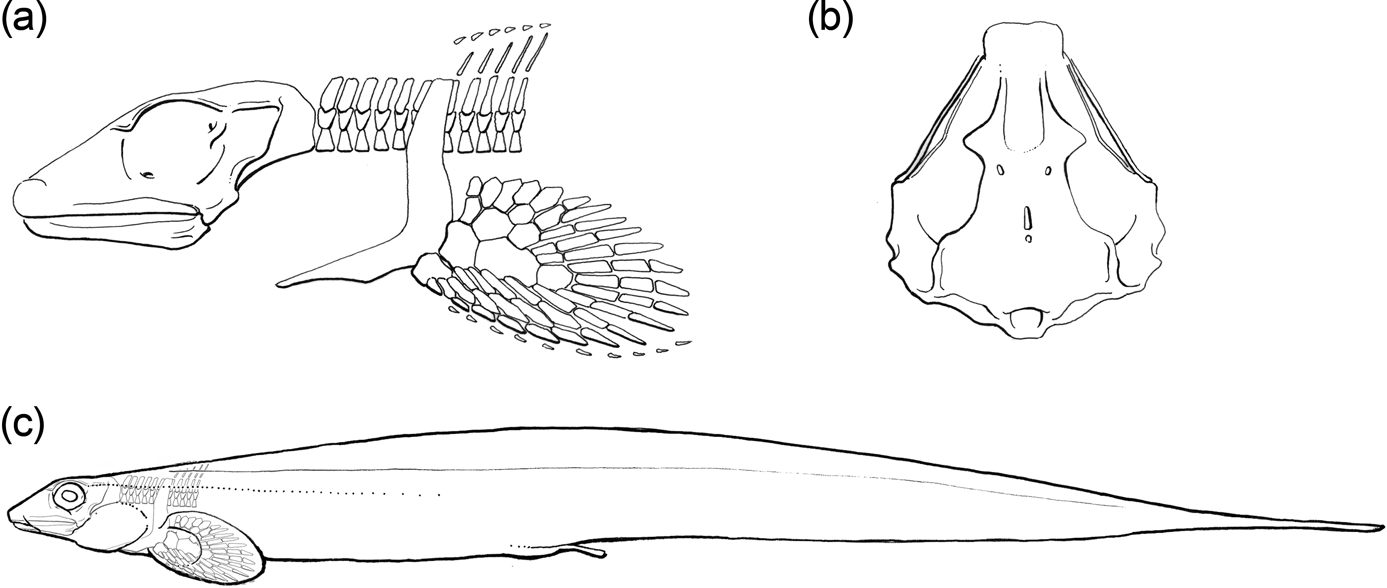
Figure 16 New reconstruction of Chondrenchelys problematica: (a) cranium, pectoral girdle and fin, and anterior section of axial skeleton in lateral view; (b) cranium in dorsal view; (c) complete body outline in lateral view. Compare (c) with Figure 1.
4. Discussion
Extant elasmobranchs and holocephalans are morphologically distinct and disjunct clades, and this marked dissimilarity in the Recent has contributed to a growing debate over the nature of ancestral chondrichthyan conditions and membership along the stem elasmobranch, holocephalan and chondrichthyan lineages. Effectively, the central question concerns the phylogenetic depth of the node defining the crown-clade of Chondrichthyes, and it is worth noting that this issue bears further on estimates of plesiomorphic gnathostome conditions (Maisey Reference Maisey1984, Reference Maisey2012; Janvier Reference Janvier1996; Coates & Sequeira Reference Coates and Sequeira2001; Brazeau Reference Brazeau2009; Anderson et al. Reference Anderson, Friedman, Brazeau and Rayfield2011; Gillis et al. Reference Gillis, Rawlinson, Bell, Lyon, Baker and Shubin2011, Reference Gillis, Modrell and Baker2013; Davis et al. Reference Davis, Finarelli and Coates2012; Finarelli & Coates Reference Finarelli and Coates2012; Pradel et al. Reference Pradel, Didier, Casane, Tafforeau and Maisey2013; Zhu et al. Reference Zhu, Yu, Ahlberg, Choo, Lu, Qiao, Qu, Zhao, Jia, Blom and Zhu2013). Relating the morphological disparity of living chondrichthyans to the increasingly detailed range of Palaeozoic forms presents a growing challenge; hence the significance of the new Mumbie Quarry specimens, which offer a more detailed and effective comparison between ancient and modern holocephalans.
The following discussion attempts to compare and contrast what we now know of the skeletal anatomy of Chondrenchelys to character states used in several recent phylogenetic analyses, highlighting likely new characterisations of morphology that may then be incorporated into future analyses. We do not undertake a phylogenetic reconstruction here; a comprehensive analysis will be undertaken in a related study (currently in preparation) on undescribed specimens from Bearsden, Scotland (Dick Reference Dick1978; Wood Reference Wood1982; Stahl Reference Stahl and Schultze1999). Character states discussed below are drawn principally from Pradel et al.'s (Reference Pradel, Tafforeau, Maisey and Janvier2011) analysis of early chondrichthyans, Stahl's (Reference Stahl and Schultze1999) summary cladogram of holocephalan interrelationships, and Patterson's (Reference Patterson1965) seminal list of “major characters peculiar to the living chimaeroids”.
Few detailed illustrations of the modern chimaeroid chondrocranium are available, in contrast to the numerous photographs, line drawings and, increasingly, rendered CT scans of modern and fossil elasmobranchs. The recently published CT scan of an embryonic Callorhincus milii (Khonsari et al. Reference Khonsari, Seppala, Pradel, Dutel, Clement, Lebedev, Ghafoor, Rothova, Tucker, Maisey, Fan, Kawasaki, Ohazama, Tafforeau, Franco, Helms, Haycraft, David, Janvier, Cobourne and Sharpe2013; Pradel et al. Reference Pradel, Didier, Casane, Tafforeau and Maisey2013) represents a notable addition to this still limited data set. Most depictions of chimaeroid anatomy are recycled from Dean (Reference Dean1906) and de Beer & Moy-Thomas (Reference de Beer and Moy-Thomas1935), although several others are available (e.g., Kesteven Reference Kesteven1932; Ribbnik Reference Ribbnik1971; Didier Reference Didier1995). This has prompted the preparation of new photographs of a cleared and stained braincase and palate of a pre-hatchling elephant shark, Callorhincus milii (Fig. 6a–c). This specimen is at a slightly younger stage than the example depicted in newly available CT scans (Khonsari et al. Reference Khonsari, Seppala, Pradel, Dutel, Clement, Lebedev, Ghafoor, Rothova, Tucker, Maisey, Fan, Kawasaki, Ohazama, Tafforeau, Franco, Helms, Haycraft, David, Janvier, Cobourne and Sharpe2013; Pradel et al. Reference Pradel, Didier, Casane, Tafforeau and Maisey2013). Importantly, these examples of pre-hatchling Callorhinchus display little of the dorsoventral offset between the levels of the basicranium and quadrate observed in adults (Kesteven Reference Kesteven1932, figs 3, 4; Didier Reference Didier1995, fig. 18A), permitting a remarkably straightforward comparison with many of the morphological features observed in Chondrenchelys.
4.1. Skeletal tissues, dentition and scales
Multilayered tesserae of calcified cartilage in the outer neurocranial wall was suggested by Schaeffer (Reference Schaeffer1981) and used by Pradel et al. (Reference Pradel, Tafforeau, Maisey and Janvier2011) to unite a subgroup of early chondrichthyans exclusive of holocephalans. Although Chondrenchelys shows no evidence of the multilayered condition, multilayered tesserae are evident in the laterally compressed and fractured preorbital region of Harpagofututor (CMNH 27326). The visibility of such tesserae (Fig. 7b) in Chondrenchelys and several other early holocephalan genera, contrasts markedly with the absence of superficially visible tesserae in extant chimaeroids. Consequently, we suggest that visible tesserae at the cartilage surface is plesiomorphic relative to crown clade holocephalans.
Chondrenchelys is united with the holocephalans on the basis of a reduced number of tooth families and the presence of tooth crowns reinforced with hypermineralised tissue (Stahl Reference Stahl and Schultze1999). Finarelli & Coates (Reference Finarelli and Coates2012) noted that the gross morphology of the large posterior tooth plates in Chondrenchelys closely resembles Mesozoic and Cenozoic holocephalans. Moreover, growth of the posterior tooth plates in Chondrenchelys and these more recent holocephalans is achieved via accretion along the caudal boundary. These similarities are consistent with our observation that Chondrenchelys and Harpagofututor share very similar tooth plate histologies (e.g., CM 27326A, CM 41091, CM 37521), and Lund's (Reference Lund1982, p. 944) observation that tooth plate histology in Harpagofututor is “virtually indistinguishable” from the Triassic and Jurassic genus Myriacanthus. However, while the crowns of these tooth plates contain tubular dentine (Patterson Reference Patterson1965), in Chondrenchelys, it is not clear that this distinctive histology is restricted to the tritors (Stahl Reference Stahl and Schultze1999).
Chondrenchelys does not possess a sparse scattering of simple conical scales, contra Stahl (Reference Stahl and Schultze1999). Scale covering is greatly reduced (e.g., Patterson Reference Patterson1965), but we note distinct scale morphologies specific to different body regions, with the pectoral fin extremity being the most notable example. Differing from Harpagofututor (Lund Reference Lund1982), in Chondrenchelys, scales are monocuspid, non-growing (synchronomorial) and placoid, and therefore structurally similar to modern elasmobranchs (Patterson Reference Patterson1965).
4.2. Cranial morphology
The continuous orbit floor in Chondrenchelys (Figs 6, 8) suggests that substantial development of a basitrabecular process (de Beer Reference de Beer1937; see also Pradel et al. Reference Pradel, Tafforeau, Maisey and Janvier2011) contributes to the holostylic cranium. It is also apparent that the skull is not tropibasic (Pradel et al. Reference Pradel, Tafforeau, Maisey and Janvier2011), and the orbits are neither elevated nor continuous across the midline dorsal to the space for the forebrain (Patterson Reference Patterson1965). The roof of the ethmoid region is closed in Chondrenchelys, with no precerebral fontanelle (Pradel et al. Reference Pradel, Tafforeau, Maisey and Janvier2011) (Fig. 6), a condition that is widespread among fossil holocephalans and iniopterygians (Pradel Reference Pradel2010). However, in Chondrenchelys, the ethmoid roof is depressed centrally, deflected upward on either side forming a ridge along a vertical cartilage (likely the orbitonasal lamina, Fig. 6d, e). In this respect, Chondrenchelys resembles Helodus (Patterson Reference Patterson1965; pers. obs. JAF & MIC: NHMUK P.8212), which also exhibits a troughed roof of the ethmoid region, flanked by upgrowths of the lamina orbitonasalis without a precerebral fontanelle (Moy-Thomas Reference Moy-Thomas1936; Patterson Reference Patterson1965). In Helodus, foramina for the superficial ophthalmic nerves are anteriorly-directed (Patterson Reference Patterson1965, fig. 39) suggesting that the steeply-downturned ethmoid roof reflects in-life morphology, not post-mortem collapse. The presence of a trough in the ethmoid region of Helodus has been proposed as an incipient ethmoid canal (de Beer & Moy-Thomas Reference de Beer and Moy-Thomas1935; Didier Reference Didier1995). However, Patterson (Reference Patterson1965) doubted this interpretation, hypothesising that the ethmoid canal appeared late in holocephalan phylogeny, due to lateral compression of an originally broad cranium. From our observations of these new specimens (which are manifestly uncompressed laterally), it is clear that there is no ethmoid canal in Chondrenchelys and that this trough is not homologous to the ethmoid canal. We suggest that this trough constitutes a potential early holocephalan synapomorphy, but the relationship to conditions in crown group holocephalans and other, putative members of the total group (e.g. sibyrhynchid iniopterygians (Pradel et al. Reference Pradel, Langer, Maisey, Geffard-Kuriyama, Cloetens, Janvier and Tafforeau2009a; Pradel Reference Pradel2010) remains unclear.
The presence of efferent superficial ophthalmic foramina opening below and anterior to the origin of the antorbital crest constitutes a further, as yet unexploited, morphological character visible in a subgroup of those holocephalan taxa that lack an ethmoid canal (e.g., Squaloraja, Helodus). In contrast, Chondrenchelys exhibits a condition similar to elasmobranchs (possibly plesiomorphic for chondrichthyans, although this remains to be tested) where the largest and most anterior superficial ophthalmic foramen perforates the cranial roof postero-medial to the antorbital crest.
As in living chimaeroids, the ethmoid region of Chondrenchelys is elongate, with separation of the anterior boundary of the orbit from the posterior margin of the nasal capsules presenting a broad pre-orbital surface in lateral view. This arrangement is strikingly different from that of iniopterygians (Pradel Reference Pradel2010). In living chimaeroids, the antorbital crest anchors substantial parts of the adductor mandibularis anterior and levator anguli oris muscles (Edgeworth Reference Edgeworth1935; Anderson Reference Anderson2008; MIC pers. obs.). The abbreviated postorbital region and proportionally large orbit imply that posteriorly oriented jaw adductors would impinge on the eye during contraction, whereas the combination of a pre-orbital cartilage wall (orbitonasal lamina) and well-formed antorbital crest in Chondrenchelys suggests that the bulk of the jaw adductor muscle inserted anterior to the eye, as in extant chimaeroids (Kesteven Reference Kesteven1932; Ribbnik Reference Ribbnik1971; Didier Reference Didier1995; Anderson Reference Anderson2008). The absence of a suborbital ridge in Chondrenchelys (present in modern chimaeroids: Fig. 6a, c) argues against any substantial muscle attachment below the eye. Re-positioning of the jaw adductor muscles to insert on the rostral wall has been repeatedly linked (Patterson Reference Patterson1965; Didier Reference Didier1995; Janvier Reference Janvier1996) to: (1) an anteriorly displaced jaw joint; (2) an anteriorly positioned gill basket; and (3) dorsally located orbits. Notably, all three of these characters are absent in Chondrenchelys.
Early chondrichthyans tend to exhibit a generalised crown gnathostome condition in which the postorbital arcade encloses the jugular canal (Pradel et al. Reference Pradel, Tafforeau, Maisey and Janvier2011), and the posteriorly continued route of the jugular vein is nested close to the otic capsule wall. Extant chimaeroids (Patterson Reference Patterson1965; Didier Reference Didier1995) and several fossil holocephalans (e.g., Helodus; Moy-Thomas Reference Moy-Thomas1936; Patterson Reference Patterson1965) are characterised by an alternative, specialised condition, in which the jugular vein passes through the subocular shelf via an opening known as the cranioquadrate passage (cqp) (Fig. 6b, c). In this configuration, the pathway of this major vein is remote from the otic region. Neither of these conditions is apparent in Chondrenchelys, with no evidence for either an enclosed passage or a jugular route close to the otic wall (Figs 6a, b, 8a, c). Rather, there is a laterally-positioned jugular notch (Fig. 6d, e), which is similar to the notch visible in the comparably sized six-month embryo of Hydrolagus colliei (see: Dean Reference Dean1906, fig. 84b). Later in ontogeny, this notch closes around the jugular vein with the expansion of the palatoquadrate cartilage to form the cranio-quadrate passage (see Fig. 6b, c). Significantly, a jugular canal is now known to be present in iniopterygians (Pradel Reference Pradel2010). Therefore, Chondrenchelys appears to show an alternative condition, a jugular notch in the ventral part of the lateral margin of the postorbital arcade or rim, much like pre-hatchling chimaeroids prior to full enclosure of the jugular vein (Dean Reference Dean1906; de Beer & Moy-Thomas Reference de Beer and Moy-Thomas1935; de Beer Reference de Beer1937).
The otico-occipital region is chimaeroid-like in several respects, including its diminutive proportions relative to the orbital and ethmoid regions, the absence of persistent cranial fissures and the absence of a dorsal otic ridge bordering part of the endolymphatic fossa (Schaeffer Reference Schaeffer1981; Coates & Sequeira Reference Coates and Sequeira1998; Pradel et al. Reference Pradel, Tafforeau, Maisey and Janvier2011; Davis et al. Reference Davis, Finarelli and Coates2012). The postorbital arcade requires additional mention here, as it flanks the anterior portion of the otic capsule, and therefore the inferred location of the anterior ampulla. However, with the exception of otico-occipital proportions, Pradel et al. (Reference Pradel, Tafforeau, Maisey and Janvier2011) note that these characteristics are also present, to varying degrees, in crown-clade elasmobranchs, making their status as holocephalan synapormorphies uncertain.
In placoderms, osteichthyans, Acanthodes and many early chondrichthyans, the occipital block is positioned posterior to the otic capsules, in contrast to extant and fossil elasmobranchs, where the occipital block is “wedged” between the otic capsules (Maisey Reference Maisey2005). There is no evidence in Chondrenchelys for any such “wedging” of the occipital unit and, in Callorhinchus, occipital arch intrusion between the otic capsules is minimal: note the anterior-posterior level of the posterior rim of the endolymphatic fossa (Fig. 6a–c, fe). Indeed, the condition in Chondrenchelys is similar to what is observed in Acanthodes (Davis et al. Reference Davis, Finarelli and Coates2012, supplementary figs 15a, c–e, End, Pdf) and Cladodoides (Maisey Reference Maisey2005, fig. 4). Thus, if slight intrusion of the occipital arch between the otic capsules represents a diagnostic character state, it must represent a synapomorphy for a much deeper node in gnathostome phylogeny.
Few chimaeroid synapomorphies have been identified in the lower jaw. Stahl (Reference Stahl and Schultze1999) comments on symphysial fusion, now known to be present in Chondrenchelys. The terminal posterior articulation of the mandible is also characteristic of chimaeroids and some broader set of fossil holocephalans, notably including Chondrenchelys.
4.3. Postcranial morphology
Like modern chimaeroids, the vertebral column of Chondrenchelys is polyspondylous with variable registration between arch and chordacentra elements. Yet, in other respects the axial skeleton of Chondrenchelys is not representative of extant holocephalan conditions. The anguiliform body shape is a morphotype confined to only a handful of Palaeozoic members of the clade. The absence of a synarcual and dorsal fin spine is also unusual, but it is unclear if this represents apomorphic or symplesiomorphic conditions. Among holocephalans, a synarcual cartilage seems to be functionally linked to muscular support for the dorsal fin spine (Didier Reference Didier1995), thus absence in Chondrenchelys might be secondarily derived. Harpacanthus (Lund & Grogan Reference Lund, Grogan, Arratia, Wilson and Cloutier2004), a non-holostylic cartilaginous fish, but likely close relative of the Holocephali from Bear Gulch, provides an intriguing comparison, as it also lacks a fin spine/synarcual, and possesses a sub-anguilliform postcranium with a single, extended dorsal fin. The posterior off-set of the gill skeleton and pectoral girdle relative to the position normally observed in holocephalans might also be linked to the anguiliform postcranial proportions in Chondrenchelys, rather than representing plesiomorphic chondrichthyan conditions. Mehta et al. (Reference Mehta, Ward, Alfaro and Wainwright2010), following Nelson (Reference Nelson1966), found a consistent relationship between ‘‘branchial-arch displacement’’ (rostral–pectoral length/cranial length) and degree of body elongation (total length/maximum body diameter). This suggests that longer eels have progressively more posteriorly displaced branchial arches, which implies that the relative positions of the gill skeleton and pectoral girdle in Chondrenchelys might be secondarily derived.
The pectoral girdle of Chondrenchelys is itself unremarkable, displaying no clear synapomorphies with the highly sculpted surfaces of extant chimaeroid examples. However, the pectoral fin of male examples (at least) is now known to be dibasal, as in other holocephalans. The monobasal condition of pelvic fins in chimaeroids is manifestly absent in Chondrenchelys, in which is also dibasal (in males at least).
The dorsal fin is highly specialised. The occasionally branched and reticulated network of axial endoskeletal in chondrenchelyids supports is unusual. The underlying network of cartilage and connective tissue in the dorsal fin was likely more extensive than the mineralised component that is preserved, which raises questions about the biomechanical consequences of this arrangement. A comparison may be made with the endoskeletal supports found in batoid wings, which also exhibit cross-bracing between radial elements that, occasionally, branch distally. Functionally, such cross-bracing is associated with oscillatory swimming requiring large amplitude deformations of the fin (Schaefer & Summers Reference Schaefer and Summers2005). In conjunction with the gross similarity in body plan and dorsal fin morphology to Gymnarchus niloticus, this could potentially offer insight into the functional nature of the midline fin and locomotion in Chondrenchelys.
4.4. General comments on the age, morphology and significance of Chondrenchelys
Perhaps the most cogent point to emphasise is that Chondrenchelys, which is the earliest holocephalan that is known from substantial skeletal remains (Moy-Thomas Reference Moy-Thomas1935; Patterson Reference Patterson1965), is much closer to conditions observed in crown-clade chimaeroids than previously appreciated (Lund Reference Lund1982; Stahl Reference Stahl and Schultze1999). This parallels previous observations that its dentition is similarly more derived than previously thought (Finarelli & Coates Reference Finarelli and Coates2012). Moreover, some of the apparent symplesiomorphies in chondrenchelyids might actually be secondary reversals associated with a highly specialised body plan. Until we have a more secure phylogenetic hypothesis for early chondrichthyans, conventionally shark-like Palaeozoic fishes must remain less securely attached to either the elasmobranch or chondrichthyan stems (Maisey Reference Maisey2012). It is, however, difficult to conceive of a credible phylogeny that would place Chondrenchelys anywhere other than the Holocephali. It follows that Chondrenchelys constitutes a secure calibration marker, providing a hard minimum age for the last common ancestor of elasmobranchs and chimaeroids.
If Chondrenchelys is to be used as a hard minimum for the divergence age estimate, we note that this age (336.5 Ma) is approximately 50 million years younger than the oldest tooth plate fragment that has been attributed to the Holocephali, from the Middle Devonian (Givetian) of France (Darras et al. Reference Darras, Derycke, Blieck and Vachard2008). However, rounded and low-crowned teeth or tooth plates with osteodentine or similar histologies evolved independently among several early gnathostome lineages. Examples include the varied shapes of lungfish tooth plates (Smith et al. Reference Smith, Smithson and Campbell1987; Smith & Chang Reference Smith and Chang1990), the specialised dentitions of hybodontid and orodontid sharks (Maisey et al. Reference Maisey, Naylor, Ward, Arratia and Tintori2004; Ginter et al. Reference Ginter, Hampe, Duffin and Schultze2010) and the bulbous surfaces of tooth plates in amphicentrid actinopterygians (Traquair Reference Traquair1879; Bradley Dyne Reference Bradley Dyne1939). The Darras et al. (Reference Darras, Derycke, Blieck and Vachard2008) datum is plausible, but alone it is insufficiently resolved to meet the criteria of a secure fossil marker (Parham et al. Reference Parham, Donoghue, Bell, Calway, Head, Holroyd, Inoue, Irmis, Joyc, Ksepka, Patane, Smith, Tarver, van Tuinen, Yang, Angielczyk, Greenwood, Hipsley, Jacobs, Makovicky, Mueller, Smith, Theodor, Warnoc and Benton2012).
By the time body fossils are observed in the fossil record, holocephalans look decidedly modern, and the stratigraphic incongruence with their outgroups implies a considerable quantity of missing data, even if a Middle to Upper Devonian date for the chondrichthyan crown radiation is postulated (consistent with the isolated tooth plate record: Stahl Reference Stahl and Schultze1999; Darras et al. Reference Darras, Derycke, Blieck and Vachard2008). Importantly, Chondrenchelys also demonstrates that the suite of cranial apomorphies characterising extant chimaeroids does not constitute a single morphological complex, despite several compelling ontogenetic arguments (Goodrich Reference Goodrich1930; de Beer & Moy-Thomas Reference de Beer and Moy-Thomas1935; de Beer Reference de Beer1937). Rather, there is a decoupling of the specialised forward-rotated jaw musculature, inserting on an extended rostral region, from other distinctly holocephalan features (e.g.: anteriorly-displaced jaw articulation; dorsally-displaced orbits with an inter-orbital septum; ethmoid canal housing the ophthalmic nerves).
Combined with observations of a decidedly modern dental morphology (Finarelli & Coates Reference Finarelli and Coates2012), Chondrenchelys expands the diversity and range of chondrichthyan ecomorphospace following the Hangenberg extinction events (Sallan & Coates Reference Sallan and Coates2010; Smithson et al. Reference Smithson, Wood, Marshall and Clack2012). The full impact of Chondrenchelys on early chondrichthyan phylogeny remains unclear, as many of the cranial features addressed in our descriptions (including Finarelli & Coates Reference Finarelli and Coates2012) are not yet adequately integrated into the context of existing phylogenetic datasets (Stahl Reference Stahl and Schultze1999; Coates & Sequeira Reference Coates and Sequeira2001; Pradel et al. Reference Pradel, Tafforeau, Maisey and Janvier2011; Grogan et al. Reference Grogan, Lund, Greenfest-Allen, Carrier, Musick and Heithaus2012). In closing, we note that body elongation and an anquliform body plan may be linked to particular ecological niches and complex feeding behaviours (Mehta et al. Reference Mehta, Ward, Alfaro and Wainwright2010) which appear to be consistent with many of the extraordinary dental and skeletal characters of this early holocephalan.
5. Acknowledgements
This project could not have been undertaken without the extraordinary dedication, talent, and particular genius for fieldwork of the late fossil collector Stan Wood. For access to specimens, we thank the staffs of British Geological Survey of Edinburgh and Keyworth; N. Fraser and S. Walsh of the National Museums of Scotland; N. Clark of the Hunterian Museum, University of Glasgow; C. Beard and A. Henrici of the Carnegie Museum, Pittsburgh; and Z. Johanson of the Natural History Museum, London. We thank L. C. Sallan for identification of the Hunterian Museum specimen; B. Masek for fossil preparation; and the staff of the Oriental Institute, University of Chicago, for RTI Viewer imaging. We are indebted to A. Gillis of Dalhousie University, Nova Scotia, for provision of the cleared and stained hatchling cranium of Callorhinchus millei. This project was supported by National Science Foundation grant DEB-0917922.