Maternal psychological stress, depression, and anxiety during pregnancy (prenatal stress; PNS) have been related to different outcomes in the offspring, including physical diseases and psychopathology (Avitsur, Levy, Goren, & Grinshpahet, Reference Avitsur, Levy, Goren and Grinshpahet2015; Kim, Bale, & Epperson, Reference Kim, Bale and Epperson2015; O'Donnell, O'Connor, & Glover, Reference O'Donnell, O'Connor and Glover2009; Padmanabhan, Cardoso, & Puttabyatappa, Reference Padmanabhan, Cardoso and Puttabyatappa2016). The mechanisms underlying the associations are thought to be part of developmental programming phenomena (Kim et al., Reference Kim, Bale and Epperson2015; Kofink, Boks, Timmers, & Kas, Reference Kofink, Boks, Timmers and Kas2013; Padmanabhan et al., Reference Padmanabhan, Cardoso and Puttabyatappa2016). Prenatal developmental programming refers to the effects of the intrauterine environment on the developing fetus. The fetus receives signals of factors such as maternal stress, malnutrition, and hormonal perturbations. Depending on the timing of these exposures, more or less persisting effects occur on tissue structure and function, brain development, and metabolism (Räikkönen, Seckl, Pesonen, Simons, & van den Bergh, Reference Räikkönen, Seckl, Pesonen, Simons and van den Bergh2011; van den Bergh et al., Reference van den Bergh, van den Heuvel, Lahti, Braeken, de Rooij, Entringer and Schwab2017).
These effects on the developing fetus are linked to increased risks for future physical and psychological unbalances and diseases, and may also be transmitted to the next generations (Matthews & Phillips, Reference Matthews and Phillips2012). On a molecular level, the programming takes place through phenomena such as epigenetic mechanisms (Kofink et al., Reference Kofink, Boks, Timmers and Kas2013) and telomere physiology (Belsky & Shalev, Reference Belsky and Shalev2016; Shalev et al., Reference Shalev, Entringer, Wadhwa, Wolkowitz, Puterman, Lin and Epel2013).
Many of the hypothesized programming effects are thought to be through persisting changes in fetal neurodevelopment. In the prenatal period, fetal neurons proliferate, differentiate, migrate, and aggregate, with peaks in neuronal migration between 12 and 20 weeks, and in dendritic arborization and synaptogenesis in the third trimester (Tau & Peterson, Reference Tau and Peterson2010). The developmental organization of the fetal brain is an incredibly complex process that is influenced by genes and environment, and neuroplastic responses to experiences that will modulate neuronal connectivity and communication (Tau & Peterson, Reference Tau and Peterson2010). Intrauterine programming of the fetal brain will hence influence later brain functioning and physical and psychological health.
In animal models the causality of the effects of PNS on offspring development has been shown (Bale, Reference Bale2014; Maccari, Krugers, Morley-Fletcher, Szyf, & Brunton, Reference Maccari, Krugers, Morley-Fletcher, Szyf and Brunton2014). In humans, research has mostly been observational, causality is difficult to determine, and the picture is much more complex. Although maternal reports of PNS have been related to outcomes in the offspring, and these outcomes can be long term and even reach into adulthood, the observed relations are often quite small (see meta-analysis by Littleton, Bye, Buck, & Amacker, Reference Littleton, Bye, Buck and Amacker2010). In addition, the proposed maternal physiological reaction to PNS and key candidate for programming effects on the developing fetus is an unbalanced hypothalamic–pituitary–adrenal (HPA) axis characterized by heightened cortisol concentrations (Mina & Reynolds, Reference Mina and Reynolds2014; Reynolds, Reference Reynolds2016; Zijlmans, Riksen-Walraven, & de Weerth, Reference Zijlmans, Riksen-Walraven and de Weerth2015). However, reported maternal PNS is not always significantly or strongly associated with maternal cortisol assessments (Harville, Savitz, Dole, Herring, & Thorp, Reference Harville, Savitz, Dole, Herring and Thorp2009; Voegtline et al., Reference Voegtline, Costigan, Kivlighan, Laudenslager, Henderson and DiPietro2013).
The fact that associations found between PNS and outcome are often small and that PNS is not always associated to cortisol concentrations may be because several physiological mechanisms are linked to PNS. As mentioned before, the traditional research focus in humans has mostly been on cortisol as a physiological marker for maternal PNS. This may have limited the possibilities of uncovering other mechanisms that may act in concert with the HPA axis or even independently from the HPA axis (Beijers, Buitelaar, & de Weerth, Reference Beijers, Buitelaar and de Weerth2014). Moreover, many programming target systems, such as the fetal HPA axis, appear to be most vulnerable for programming effects in late pregnancy, further stressing the need to uncover additional early pregnancy explanatory mechanisms for the effects of PNS on the offspring (Rakers et al., Reference Rakers, Rupprecht, Dreiling, Bergmeier, Witte and Schwab2017). Recently, other pathways that go beyond the HPA axis have been proposed and studied. These mechanisms include the immune system (Avitsur et al., Reference Avitsur, Levy, Goren and Grinshpahet2015; Christian, Glaser, Porter, & Iams, Reference Christian, Glaser, Porter and Iams2013; Coussons-Read, Okun, & Nettles, Reference Coussons-Read, Okun and Nettles2007), the intestinal microbiota (Beijers et al., Reference Beijers, Buitelaar and de Weerth2014; Zijlmans, Korpela, Riksen-Walraven, de Vos, & de Weerth, Reference Zijlmans, Korpela, Riksen-Walraven, de Vos and de Weerth2015), serotonin (Bonnin & Levitt, Reference Bonnin and Levitt2011; St.-Pierre, Laurent, King, & Vaillancourt, Reference St.-Pierre, Laurent, King and Vaillancourt2016), the placenta (Appleton et al., Reference Appleton, Armstrong, Lesseur, Lee, Padbury, Lester and Marsit2013; Jensen Peña, Monk, & Champagne, Reference Jensen Peña, Monk and Champagne2012; O'Donnell et al., Reference O'Donnell, O'Connor and Glover2009), reactive oxygen species and oxidative stress (Padmanabhan et al., Reference Padmanabhan, Cardoso and Puttabyatappa2016; Rakers et al., Reference Rakers, Rupprecht, Dreiling, Bergmeier, Witte and Schwab2017), and the sympathetic nervous system (La Marca-Ghaemmaghami, Dainese, La Marca, Zimmermann, & Ehlert, Reference La Marca-Ghaemmaghami, Dainese, La Marca, Zimmermann and Ehlert2015; Wroble-Biglan, Dietz, & Pienkosky, Reference Wroble-Biglan, Dietz and Pienkosky2009). All these mechanisms may “program” the offspring by affecting fetal neurodevelopment, which in turn may affect the offspring's behavior, emotions, and metabolism (Mina & Reynolds, Reference Mina and Reynolds2014; Rakers et al., Reference Rakers, Rupprecht, Dreiling, Bergmeier, Witte and Schwab2017; Schuurmans & Kurrasch, Reference Schuurmans and Kurrasch2013). In sum, maternal stress transmitted to the fetus through additional pathways may at least in part explain why the associations between PNS, assessed with maternal cortisol concentrations, and child outcome are often small (Zijlmans, Riksen-Walraven, et al., Reference Zijlmans, Riksen-Walraven and de Weerth2015).
An additional problem that may be slowing our advances in this research area is that PNS may lead to different physiological mechanisms depending on how the woman copes with this stress. For example, PNS may affect different aspects of a woman's lifestyle behaviors, more specifically her patterns and quality of sleep, diet, and exercise, as well as in some cases, her use of alcohol, cigarettes, and drugs/medication. Lifestyle behaviors are often seen as “loose” risk factors (Marques, Bjørke-Monsen, Teixeira, & Silverman, Reference Marques, Bjørke-Monsen, Teixeira and Silverman2015; Monk, Georgieff, & Osterholm, Reference Monk, Georgieff and Osterholm2013) or included as confounders in studies of maternal PNS and outcome (e.g., for sleep: O'Connor et al., Reference O'Connor, Tang, Gilchrist, Moynihan, Pressman and Blackmore2014; Rallis, Skouteris, McCabe, & Milgrom, Reference Rallis, Skouteris, McCabe and Milgrom2014). However, they may have a much more fundamental role in models of PNS: they may constitute essential and understudied mediators between PNS and physiology. Uncovering their potential mediating roles will aid the understanding of programming mechanisms behind maternal PNS. Examples of investigations providing evidence for such mediating roles for lifestyle factors are a prospective study that showed that prenatal maternal depression was directly associated with lowered cognition in the 8-year-old offspring, as well as indirectly through maternal nutrition choices (Barker, Kirkham, Ng, & Jensen, Reference Barker, Kirkham, Ng and Jensen2013), and a study showing that pregnancy-specific stress was directly related to preterm delivery and indirectly to low birth weight through an association with maternal smoking (Lobel et al., Reference Lobel, Cannella, Graham, DeVincent, Schneider and Meyer2008).
This theoretical review will focus on the associations of lifestyle changes in sleep, diet, and exercise with PNS, as well as on the potential underlying mechanisms and causal pathways through which these factors may affect the offspring. The goal is to stimulate thought and discussion that may help advance the field of PNS research. The papers that are discussed are examples and often reviews on the topics, and not a comprehensive review of the literature. The term PNS will be used throughout the manuscript as an overbridging term for maternal stress, anxiety, and depression. Research has shown that stress leads to increases in substance (ab)use, also in pregnancy (Lobel et al., Reference Lobel, Cannella, Graham, DeVincent, Schneider and Meyer2008). Nonetheless, because the prenatal use of teratogens such as nicotine, alcohol, and drugs, also in relation to PNS, has been extensively reviewed in the past (Forray & Foster, Reference Forray and Foster2015; Huizink, Reference Huizink2012; Kofink et al., Reference Kofink, Boks, Timmers and Kas2013; Shankaran et al., Reference Shankaran, Lester, Das, Bauer, Bada, Lagasse and Higgins2007), it will not be a subject of this review. See Figure 1 for a pictorial representation of the heuristic model that is presented in this review. Note that potential links between PNS, lifestyle, and physiology will be moderated by person-specific variables of the mother such as genes, personality, social support, coping skills, and experience. For example, women with high self-esteem and high perceived control over pregnancy have been found to practice more health-promoting behaviors during pregnancy (Auerbach, Lobel, & Cannella, Reference Auerbach, Lobel and Cannella2014). These factors are beyond the scope of the present paper, but should be kept in mind in future human studies on PNS as they also add to the complexity of understanding the impact of PNS on the developing fetus.
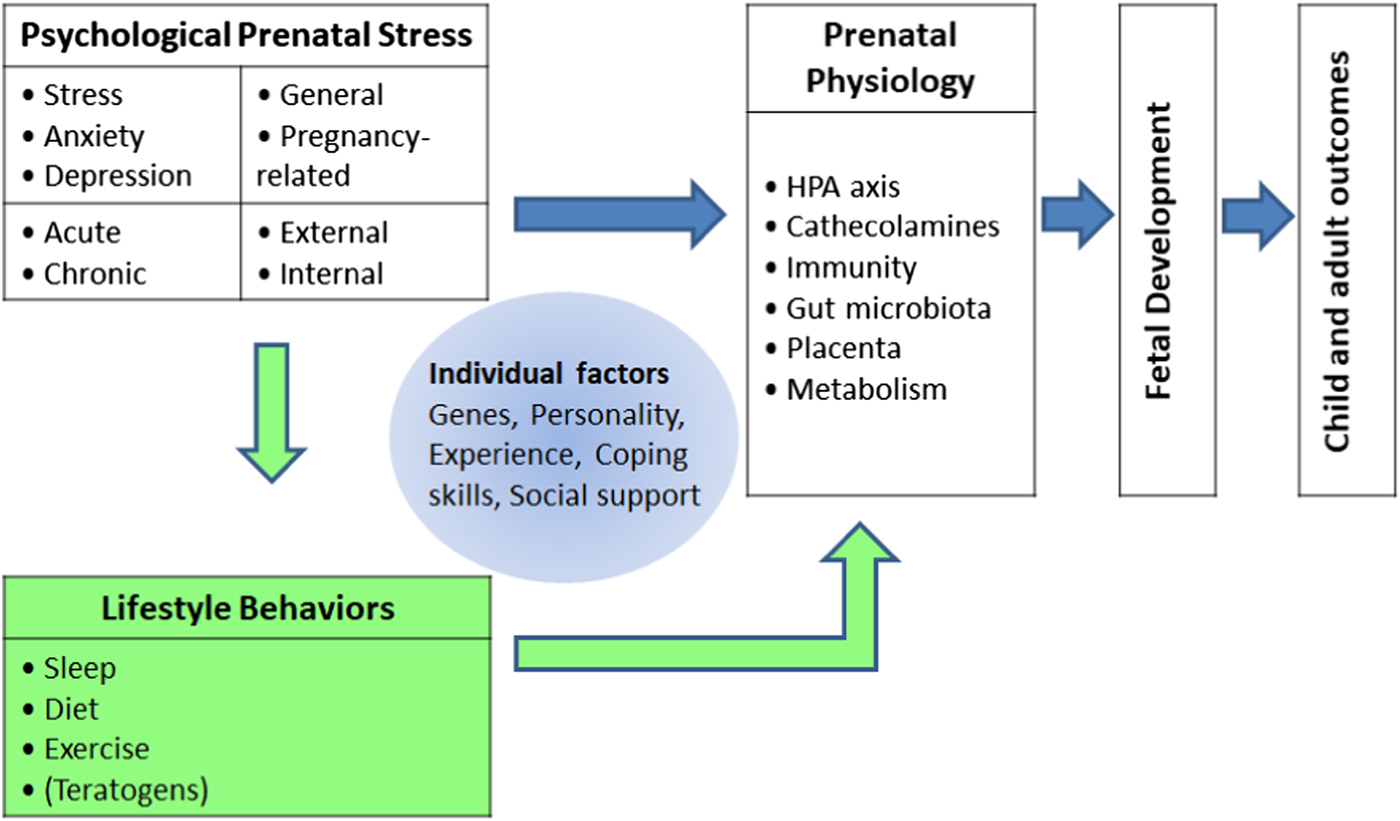
Figure 1. Multidimensional model of mechanisms through which maternal prenatal stress may affect fetal and later child development. Factors triggering prenatal stress may be diverse, ranging from socioeconomic and cultural problems to current and past relationship problems. The focus of this review is on the potential mediating role played by sleep, diet, and exercise in the links between prenatal stress and prenatal physiology.
This review's first research question is: How is PNS associated with sleep, diet, and exercise? In other words, what evidence is there for relations between PNS and lifestyle changes in sleep, diet, and exercise? Second, an important issue in PNS research is that many types and aspects of stress are put under the umbrella of maternal prenatal psychological stress. However, as depicted in Figure 1, there are clearly distinct types of PNS, such as anxiety, stress, depression, and acute versus chronic, as well as general or pregnancy related, and possibly also PNS originating from external, situational, “objective” factors (e.g., poverty, war, or a natural disaster) or internal, “subjective” factors (e.g., psychopathology or reported distress; Alderdice, Lynn, & Lobel, Reference Alderdice, Lynn and Lobel2012; Beijers et al., Reference Beijers, Buitelaar and de Weerth2014; King & Laplante, Reference King and Laplante2015; Shapiro, Fraser, Frasch, & Séguin, Reference Shapiro, Fraser, Frasch and Séguin2013). These distinct types of PNS and adversity have been linked to different outcomes in offspring (Alkon et al., Reference Alkon, Boyce, Tran, Harley, Neuhaus and Eskenazi2014) and may be uniquely related to specific changes in lifestyle. For example, women with pregnancy-related anxiety may be especially prone to sleeping problems, or women with chronic stress may be especially likely to adopt poor diets. The second research question is therefore: Can specific patterns of associations between types of PNS and lifestyle behaviors be found? Finally, the different lifestyle behaviors that will be reviewed may have different effects on fetal (neuro)development. For example, excessive exercise may increase cathecolamines and reduce blood flow to the uterus and result in growth retardation, while poor diet may result in vitamin deficiency, which in turn affects neurodevelopment. This leads to our third research question: Through which underlying mechanisms can lifestyle behaviors affect maternal physiology and, in turn, fetal development?
In the coming sections, sleep, diet, and exercise will be discussed individually, attempting to answer these three research questions for each. The paper will finalize with a general conclusion, discussion, and suggestions for future research.
PNS and Sleep
Sleep: Evidence of associations
Pregnancy brings about many physical, psychological, and physiological changes in women. Sleep disorders and complaints are common in pregnancy, and may occur in all three trimesters (Palagini et al., Reference Palagini, Gemignani, Banti, Manconi, Mauri and Riemann2014; Tamanna & Geraci, Reference Tamanna and Geraci2013). The quality of sleep also gradually declines throughout pregnancy (Hung, Tsai, Ko, & Chen, Reference Hung, Tsai, Ko and Chen2013; Polo-Kantola, Aukia, Karlsson, Karlsson, & Paavonen, Reference Polo-Kantola, Aukia, Karlsson, Karlsson and Paavonen2017). The nature of the disturbances varies and includes insomnia, short duration of sleep, poor quality of sleep, sleep-disordered breathing, parasomnias, and restless legs syndrome (Pien & Schwab, Reference Pien and Schwab2004; Santiago, Nolledo, Kinzler, & Santiago, Reference Santiago, Nolledo, Kinzler and Santiago2001).
Although to a certain extent these complaints are an inherent feature of human pregnancy, they have also been related to pregnancy complications and adverse outcomes, such as preeclampsia, gestational hypertension, gestational diabetes mellitus, fetal growth abnormalities, preterm birth, and intrauterine growth restriction (Abeysena, Jayawardana, & de A Seneviratne, Reference Abeysena, Jayawardana and de A Seneviratne2009; Cain & Louis, Reference Cain and Louis2016; Carnelio, Morton, & McIntyre, Reference Carnelio, Morton and McIntyre2017; Okun, Roberts, Marsland, & Hall, Reference Okun, Roberts, Marsland and Hall2009; Palagini et al., Reference Palagini, Gemignani, Banti, Manconi, Mauri and Riemann2014). Moreover, some of these proposed effects of sleep disturbances and disorders during pregnancy overlap with those of PNS, pointing at a possible mediating role of sleep in the link between PNS and outcomes.
Psychological stress has been broadly implicated in the etiology of sleep complaints or problems (Babson & Feldner, Reference Babson and Feldner2010; Harvey, Gehrman, & Espie, Reference Harvey, Gehrman and Espie2014; Omholt, Tveito, & Ihlebæk, Reference Omholt, Tveito and Ihlebæk2017; Taouk, Farrow, & Schulkin, Reference Taouk, Farrow and Schulkin2017). Moreover, sleep has also been proposed as a mediator between traumatic stress and behavioral and health problems in children and adults (Spilsbury, Reference Spilsbury2009; Vandrey, Babson, Herrmann, & Bonn-Miller, Reference Vandrey, Babson, Herrmann and Bonn-Miller2014). In addition, nearly all mood and anxiety disorders co-occur with sleep disturbances, and emotional processing in the brain and sleep are hypothesized to influence each other causally and bidirectionally (Goldstein & Walker, Reference Goldstein and Walker2014). Note, however, that most studies report correlational associations and causality cannot be implied. Evidence for the reverse causal relations has also been reported. For example, in a study of nonpregnant women, one night of sleep deprivation led to changes in cortisol rhythms (Omisade, Buxton, & Rusak, Reference Omisade, Buxton and Rusak2010).
In early pregnancy, different aspects of poorer sleep quality have been related to maternal perceived stress (Okun et al., Reference Okun, Kline, Roberts, Wettlaufer, Glover and Hall2013; Okun, Tolge, & Hall, Reference Okun, Tolge and Hall2014); higher scores on a questionnaire assessing depression, anxiety, and stress (Qiu et al., Reference Qiu, Gelaye, Zhong, Enquobahrie, Frederick and Williams2016); more perceived stress in women with a diagnosis of a mood or anxiety disorder (Qiu, Gelaye, Fida, & Williams, Reference Qiu, Gelaye, Fida and Williams2012); and stress-induced sleep disturbance (Zhong, Gelaye, Sánchez, & Williams, Reference Zhong, Gelaye, Sánchez and Williams2015). In a recent study using biological markers of stress in pregnant women, lower cortisol reactivity to a psychosocial stressor in the second trimester was related to reduced sleep quality (Crowley et al., Reference Crowley, O'Buckley, Schiller, Stuebe, Morrow and Girdler2016). In contrast, in overweight and obese pregnant women, depression, but not stress, was associated with more sleep problems (Chang, Brown, Nitzke, Smith, & Eghtedary, Reference Chang, Brown, Nitzke, Smith and Eghtedary2015). Although in general results point at maternal pregnancy stress to be associated with sleep problems, studies researching sleep in pregnancy in relation to reported psychological stress are as yet scarce.
Pregnancy anxiety and depression symptoms have been more widely studied in relation to sleep and generally point in the direction of more psychological complaints being related to more sleep problems. Higher depression scores were related to worse sleep quality (Hung et al., Reference Hung, Tsai, Ko and Chen2013; Mellor, Chua, & Boyce, Reference Mellor, Chua and Boyce2014), short sleep duration, insufficient sleep or insomnia in early pregnancy (Okun et al., Reference Okun, Kline, Roberts, Wettlaufer, Glover and Hall2013), and more sleep disturbances in depressed women compared to nondepressed in late pregnancy (Okun, Kiewra, Luther, Wisniewski, & Wisner, Reference Okun, Kiewra, Luther, Wisniewski and Wisner2011). In late pregnancy also, women with depressive symptoms had worse quality of sleep, after controlling for anxiety (Ruiz-Robledillo, Canário, Dias, Moya-Albiol, & Figueiredo, Reference Ruiz-Robledillo, Canário, Dias, Moya-Albiol and Figueiredo2015). Studies looking at depression and anxiety symptoms together found that they were associated with sleep disturbances in middle and late pregnancy (Polo-Kantola et al., Reference Polo-Kantola, Aukia, Karlsson, Karlsson and Paavonen2017), to subjective sleep disturbances but not to objective sleep disturbances (Volkovich, Tikotzky, & Manber, Reference Volkovich, Tikotzky and Manber2016), and that women with comorbid depression and anxiety had more sleep disturbances than healthy women, but similar sleep disturbances to women with either depression or anxiety alone (Field et al., Reference Field, Diego, Hernandez-Reif, Figueiredo, Deeds, Ascencio and Kuhn2010). In early pregnancy, depression and generalized anxiety disorder were associated with poorer sleep quality (Zhong et al., Reference Zhong, Gelaye, Sánchez and Williams2015), and women with the highest levels of anxiety and depressive symptoms had the lowest sleep quality, and also the largest decreases in sleep quality from early to late pregnancy (Tomfohr, Buliga, Letourneau, Campbell, & Giesbrecht, Reference Tomfohr, Buliga, Letourneau, Campbell and Giesbrecht2015). Finally, in pregnant women seeking outpatient psychiatric treatment, depression and anxiety symptoms were related to insomnia (Swanson, Pickett, Flynn, & Armitage, Reference Swanson, Pickett, Flynn and Armitage2011).
In sum, PNS is related to sleep disturbances in pregnancy, and these relations cover all three trimesters. In general, sleep problems can therefore not be attributed solely to factors such as early pregnancy nausea or increased urinary frequency, or the increased abdominal size and physical discomfort that is especially prominent in late pregnancy.
Sleep: Specific patterns of associations
With respect to our second research question, the evidence reviewed here indicates that depression and anxiety may be more strongly related to sleep problems than stress, but given the scarcity of studies looking at stress variables, this is a tentative conclusion. Note that because depression and anxiety are often comorbid and also studied together, it is not possible to conclude whether one or the other is more strongly related to sleep disturbances.
Sleep: Potential underlying mechanisms
Several physiological mechanisms may explain the proposed effects of maternal sleep disturbances on fetal development and child outcomes. In general, sleep disturbances affect several biological systems, including the neuroendocrine, metabolic, and inflammatory systems (Okun et al., Reference Okun, Roberts, Marsland and Hall2009). Sleep is thought to regulate immune system functioning and the sympathetic nervous system, and insomnia has been related to decreases in natural immunity in the form of decreased natural killer cell activity and nocturnal sympathetic arousal (Irwin, Clark, Kennedy, Christian Gillin, & Ziegler, Reference Irwin, Clark, Kennedy, Christian Gillin and Ziegler2003). Maternal immunosuppression may render the mother vulnerable to prenatal infections due to toxoplasma, influenza, or herpes simplex viruses, which have been associated with severe neurodevelopmental pathologies in the offspring, such as autism, psychosis, and schizophrenia (Howerton & Bale, Reference Howerton and Bale2012; Kim et al., Reference Kim, Bale and Epperson2015).
Nocturnal sympathetic arousal due to sleep disturbances may result in increased catecholamine concentrations, which have been associated with preterm birth (Holzman et al., Reference Holzman, Senagore, Tian, Bullen, Devos, Leece and Sapkal2009). In turn, increased levels of catecholamines lead to decreases in uteroplacental perfusion, and decreases in fetal oxygen and nutrient supply, producing hypoxic stress in the fetus (Gitau, Fisk, & Glover, Reference Gitau, Fisk and Glover2001; Rakers et al., Reference Rakers, Bischoff, Schiffner, Haase, Rupprecht, Kiehntopf and Schwab2015, Reference Rakers, Rupprecht, Dreiling, Bergmeier, Witte and Schwab2017). In a sheep model, the fetus has been found to be very sensitive to decreases in blood flow to the uterus and to react to even small changes by increasing the production of catecholamines (Rakers et al., Reference Rakers, Bischoff, Schiffner, Haase, Rupprecht, Kiehntopf and Schwab2015). These fetal catecholamines may directly further affect intrauterine development.
Sleep disturbances in pregnancy have also been associated with increased pro-inflammatory activity of the immune system (Okun & Coussons-Read, Reference Okun and Coussons-Read2007; Okun, Hall, & Coussons-Read, Reference Okun, Hall and Coussons-Read2007). Sleep disturbances in the three trimesters may have different effects on the developing fetus, depending on the specific (neuro)developmental phase. Early pregnancy sleep problems have been proposed to have a greater impact on maternal physiology and to lead to adverse outcomes, such as preeclampsia, intrauterine growth retardation, and preterm birth, that have often been associated to early pregnancy factors such as inflammation and high norepinephrine levels (Okun et al., Reference Okun, Roberts, Marsland and Hall2009, Reference Okun, Kiewra, Luther, Wisniewski and Wisner2011). One way in which inflammation may lead to adverse outcomes is through interference with normal trophoblast invasion (Okun et al., Reference Okun, Roberts, Marsland and Hall2009). Trophoblasts are placental cells that originate in early pregnancy and play an important role in embryonic implantation and nutrition (Beijers et al., Reference Beijers, Buitelaar and de Weerth2014). In vitro experiments have shown that while some inflammatory markers promote trophoblast invasion, others interfere with trophoblast implantation (Salamonsen, Hannan, & Dimitriadis, Reference Salamonsen, Hannan and Dimitriadis2007; Sharma, Godbole, & Modi, Reference Sharma, Godbole and Modi2016).
High concentrations of cytokines may also affect the neurodevelopment of the fetal brain by directly acting on fetal glial cells (Ratnayake, Quinn, Walker, & Dickinson, Reference Ratnayake, Quinn, Walker and Dickinson2013). Finally, pro-inflammatory cytokines (e.g., TNF-α, IL-1, and IL-6) stimulate HPA axis activity and production of glucocorticoids (Silverman & Sternberg, Reference Silverman and Sternberg2012), which in turn can affect fetal brain development. In experimental rat studies, increases in pro-inflammatory cytokines have been causally associated with structural hippocampus brain changes and decreased learning (Samuelsson, Jennische, Hansson, & Holmäng, Reference Samuelsson, Jennische, Hansson and Holmäng2006), as well as with a hyperactive phenotype in male offspring (Bronson & Bale, Reference Bronson and Bale2014).
In support of the above, the systematic review by Palagini et al. (Reference Palagini, Gemignani, Banti, Manconi, Mauri and Riemann2014) concluded that maternal chronic sleep loss is related to negative pregnancy outcomes, and that this relation is mediated by HPA axis activation and immune/inflammatory reactions. Although none of the reviewed studies investigated the whole mediation model, according to the authors, chronic sleep loss can be both a trigger for prenatal psychological stress and the result of prenatal psychological stress. Stress and sleep would influence each other bidirectionally and result in chronic inflammation and HPA axis activation, in turn leading to adverse pregnancy outcomes.
PNS and Diet
Diet: Evidence of associations
The relevance of diet to mental health conditions such as depression and anxiety is beginning to be recognized, as exemplified by the emerging field of nutritional psychiatry research (Logan & Jacka, Reference Logan and Jacka2014). During pregnancy, links between maternal psychological well-being and nutrition may play a prominent role in explaining the mechanisms of fetal programming of future health and disease. A mother's metabolic needs increase throughout pregnancy, and in healthy pregnancies with a recommended gestational weight gain of 12 kg/26 lbs., mothers need to consume an additional 90, 287, and 466 kcal/day during the first, second, and third trimester, respectively (Bø et al., Reference Bø, Artal, Barakat, Brown, Davies, Dooley and Khan2016; Butte & King, Reference Butte and King2005). Quality and quantity issues of maternal diet are of high importance for both maternal and fetal health. For the woman, weight gain and an unhealthy diet during pregnancy may mark the start of postpartum health problems such as obesity and depression (Amorim Adegboye & Linne, Reference Amorim Adegboye and Linne2013; Chatzi et al., Reference Chatzi, Melaki, Sarri, Apostolaki, Roumeliotaki, Georgiou and Kogevinas2011; Sarwer, Allison, Gibbons, Markowitz, & Nelson, Reference Sarwer, Allison, Gibbons, Markowitz and Nelson2006). For the fetus, nutrients, including folate, iron, and certain fats, can have substantial effects on brain development impacting later cognitive development (Georgieff, Brunette, & Tran, Reference Georgieff, Brunette and Tran2015). In addition, research has shown that undernourishment or overnourishment of the mother can program obesity and metabolic syndrome in the offspring (Alfaradhi & Ozanne, Reference Alfaradhi and Ozanne2011; Fall, Reference Fall2011; Lakshmy, Reference Lakshmy2013; Palatianou, Simos, Andronikou, & Kiortsis, Reference Palatianou, Simos, Andronikou and Kiortsis2014; Sen et al., Reference Sen, Carpenter, Hochstadt, Huddleston, Kustanovich, Reynolds and Roberts2012).
In the nonpregnant population, strong links are found between eating patterns and both psychosocial stress and sleep problems. High levels of stress have been related to both lower and higher intake of food (van Strien, Herman, Anschutz, Engels, & de Weerth, Reference van Strien, Herman, Anschutz, Engels and de Weerth2012; van Strien, Ouwens, Engel, & de Weerth, Reference van Strien, Ouwens, Engel and de Weerth2014; van Strien, Roelofs, & de Weerth, Reference van Strien, Roelofs and de Weerth2013). Nevertheless, these relations cannot be assumed to be the same in pregnancy, a state accompanied by physical, hormonal, and behavioral changes. For example, cravings for specific foods can appear, and nausea or heartburn can affect hunger and diet (Fowles & Fowles, Reference Fowles and Fowles2008). Pregnant women also know that their food intake can affect their unborn child (Fowles & Fowles, Reference Fowles and Fowles2008), and may adapt their diet accordingly. However, although women are generally conscious that their diet can affect the fetus, many pregnant women do not succeed in making healthy food choices (Sarwer et al., Reference Sarwer, Allison, Gibbons, Markowitz and Nelson2006).
Psychosocial stress may be a significant factor preventing women from adopting or continuing a healthy diet during pregnancy. Studies on stress and food choices during pregnancy show that pregnant women reporting higher levels of general or pregnancy-specific stress ate more unhealthy foods and more food overall (Barker et al., Reference Barker, Kirkham, Ng and Jensen2013; Hurley, Caulfield, Sacco, Costigan, & Dipietro, Reference Hurley, Caulfield, Sacco, Costigan and Dipietro2005; Lobel et al., Reference Lobel, Cannella, Graham, DeVincent, Schneider and Meyer2008) as well as less folate (Hurley et al., Reference Hurley, Caulfield, Sacco, Costigan and Dipietro2005) and vitamins (Lobel et al., Reference Lobel, Cannella, Graham, DeVincent, Schneider and Meyer2008). Pregnant women with more psychological distress also had lower levels of an omega-3 fatty acid, specifically eicosapentaenoic acid or EPA, in serum (Hamazaki, Harauma, Otaka, Moriguchi, & Inadera, Reference Hamazaki, Harauma, Otaka, Moriguchi and Inadera2016).
With respect to depression, poorer diet quality was found in pregnant women who had more symptoms of depression (Fowles, Stang, Bryant, & Kim, Reference Fowles, Stang, Bryant and Kim2012; Huddy et al., Reference Huddy, Torres, Milte, McNaughton, Teychenne and Campbell2016). Maternal depression has also been positively related to fat intake in the first trimester (Chang et al., Reference Chang, Brown, Nitzke, Smith and Eghtedary2015; Fowles, Timmerman, Bryant, & Kim, Reference Fowles, Timmerman, Bryant and Kim2011) and to fat concentrations in serum over pregnancy (HDL cholesterol; Teofilo et al., Reference Teofilo, Farias, Pinto, Vilela, Vaz, Nardi and Kac2014). Maternal depressive and anxiety symptoms during pregnancy were associated with decreased vitamin intake (Newport et al., Reference Newport, Ji, Long, Knight, Zach, Smith and Stowe2012) and decreased fish intake, with fish being considered to positively impact fetal neurodevelopment (Emmett, Jones, & Golding, Reference Emmett, Jones and Golding2015).
Pregnant women with higher general or pregnancy-specific anxiety were more likely to gain too much weight during pregnancy (Hartley, McPhie, Fuller-Tyszkiewicz, Hill, & Skouteris, Reference Hartley, McPhie, Fuller-Tyszkiewicz, Hill and Skouteris2016; Westerneng et al., Reference Westerneng, Witteveen, Warmelink, Spelten, Honig and de Cock2017), to have a poorer diet quality (Nash, Gilliland, Evers, Wilk, & Campbell, Reference Nash, Gilliland, Evers, Wilk and Campbell2013; Vaz et al., Reference Vaz, Kac, Emmett, Davis, Golding and Hibbeln2013; Vilela et al., Reference Vilela, Pinto, Rebelo, Benaim, Lepsch, Dias-Silva and Kac2015), and to have lower levels of an omega-3 fatty acid, more specifically docosahexaenoic acid or DHA, in serum (Verly-Miguel et al., Reference Verly-Miguel, Farias, Pinto, Lepsch, Nardi and Kac2015). In late pregnancy, women who were fatigued, stressed, and anxious consumed more food overall and appeared to decrease the intake of some micronutrients (Hurley et al., Reference Hurley, Caulfield, Sacco, Costigan and Dipietro2005). In contrast, lower mood and anxiety disorder symptoms in second and third trimester pregnant Japanese women was not related to an hypothesized higher consumption of healthy fermented food (Takahashi et al., Reference Takahashi, Nishigori, Nishigori, Mizuno, Obara and Metoki2016).
Finally, Fowles et al. (Reference Fowles, Timmerman, Bryant and Kim2011) found that frequently consuming fast food in early pregnancy contributed to a poor quality diet and excessive caloric intake, possibly constituting the starting point for excessive gestational weight gain. These authors found that the combination of maternal depression, stress, and anxiety scores explained 45% of the variance in quality of diet.
The findings reported above are in line with those of a recent systematic review that concludes that prenatal depressive and stress symptoms are positively associated with poor quality and unhealthy diets, and that depressive and anxiety symptoms are negatively associated with healthy diets (Baskin, Hill, Jacka, O'Neil, & Skouteris, Reference Baskin, Hill, Jacka, O'Neil and Skouteris2015).
In sum, it is possible to cautiously conclude that PNS is related to less healthy pregnancy diets, greater food intake, and greater gestational weight gain. These findings, often based on self-report, have also been supported by measures of different nutrients in maternal serum. Hence, maternal diet may constitute a mediating factor between PNS and fetal programming. Nonetheless, despite the fact that these last years have seen an increase in research in this field, there is still a paucity of studies on the associations between PNS and diet, stressing the urgent need for more, sufficiently powered, studies on the subject (Baskin et al., Reference Baskin, Hill, Jacka, O'Neil and Skouteris2015; Monk et al., Reference Monk, Georgieff and Osterholm2013).
Diet: Specific patterns of associations
Based on the evidence presented above, we can conclude that stress, anxiety, and depression appear to be associated in similar manners and strength to a poor quality maternal diet. This means that, in answer to our second research question, there does not appear to be a stronger relation of one of these specific PNS factors with maternal pregnancy diet.
Diet: Potential underlying mechanisms
Researchers of prenatal maternal stress have recently begun to discuss the intricate associations between maternal PNS and diet and fetal programming phenomena (Entringer & Wadhwa, Reference Entringer and Wadhwa2013; Marques et al., Reference Marques, Bjørke-Monsen, Teixeira and Silverman2015; Monk et al., Reference Monk, Georgieff and Osterholm2013). Although to date there are no empirical studies on diet as a mediator between PNS and child outcome, several mechanisms have been put forward as to how diet may impact maternal physiology and in turn program fetal development. Whether due to undernutrition or selective dietary choices, one of the most important factors of a poor diet is a lack of essential nutrients (Marques et al., Reference Marques, Bjørke-Monsen, Teixeira and Silverman2015). Deficits in maternal dietary nutrients in early pregnancy will have a larger effect on neuronal proliferation, and hence on cell numbers, while nutrient deficits in later pregnancy will have a greater impact on the cell differentiation, that in the case of neurons includes dendritic arborization and synaptogenesis (Monk et al., Reference Monk, Georgieff and Osterholm2013). This is in line with the fact that fetal brain development goes through sensitive and critical periods in which different nutrients are necessary for different aspects of neurodevelopment (see review by Georgieff, Brunette, & Tran, Reference Georgieff, Brunette and Tran2015). Important nutrients for child neurodevelopment include folate, iron, B vitamins, iodine, proteins, and LC-PUFAs (long chain polyunsaturated fatty acids; present in fish, oils, and nuts). For example, LC-PUFAs are important for synaptogenesis and myelin, and a shortage of LC-PUFAs in maternal diet is, similarly to what happens with shortages of iron and zinc, associated with worse offspring cognitive performance. Protein restriction in the fetus is related to intrauterine growth restriction, which has been linked to impaired brain growth (Monk et al., Reference Monk, Georgieff and Osterholm2013). Restrictions in these essential nutrients have also been associated with effects on the immune system development and functioning (for a review, see Marques et al., Reference Marques, Bjørke-Monsen, Teixeira and Silverman2015). Furthermore, reduced maternal intake of many of these nutrients has been associated with long-term effects in the offspring that show overlap with those of PNS, such as effects on memory, learning, and behavior (Georgieff et al., Reference Georgieff, Brunette and Tran2015).
On the other side of the spectrum, and possibly a much greater problem in many developed countries nowadays, is poor dietary quality due to an excess in sugars and saturated fats. While LC-PUFAs may benefit fetal brain development, diets rich in saturated fats appear to have deleterious effects on the fetal brain. Evidence, mostly originating from animal models, shows that a high-fat diet is related to increases in maternal leptin, insulin, glucose, triglycerides, and pro-inflammatory cytokines (Marques et al., Reference Marques, Bjørke-Monsen, Teixeira and Silverman2015; Sullivan, Riper, Lockard, & Valleau, Reference Sullivan, Riper, Lockard and Valleau2015). In turn, this can lead to placental dysfunction and altered fetal neuroendocrine development, due to inflammation in the brain and alterations to the serotonergic and dopaminergic systems, as well as the HPA axis. These heightened maternal systemic levels of inflammatory markers due to high-fat diets are independent from the effects of maternal obesity (Bolton & Bilbo, Reference Bolton and Bilbo2014).
A dietary product that deserves special mention with respect to maternal prenatal dietary programming mechanisms is licorice. A primary regulator of fetal glucocorticoid exposure is the activity of placental “barrier” enzyme 11β-hydroxysteroid dehydrogenase type 2 (HSD2), which protects the fetus from exposure to excessive cortisol by converting active cortisol to inactive cortisone (Reynolds, Reference Reynolds2016). Maternal consumption of licorice products may result in increased passage of maternal cortisol to the fetal compartment as licorice contains glycyrrhizin, which is a potent inhibitor of placental HSD2 (Räikkönen et al., Reference Räikkönen, Seckl, Pesonen, Simons and van den Bergh2011, Reference Räikkönen, Martikainen, Pesonen, Lahti, Heinonen, Pyhälä and Kajantie2017). The relations between high pregnancy licorice consumption, which is common in many North European countries, and offspring outcomes, mimics those of PNS (Räikkönen et al., Reference Räikkönen, Seckl, Pesonen, Simons and van den Bergh2011, Reference Räikkönen, Martikainen, Pesonen, Lahti, Heinonen, Pyhälä and Kajantie2017). It is as yet not known whether PNS is associated with maternal consumption of licorice confectionary, but if this were the case, this may constitute an additional physiological mechanism through which potential effects of PNS on the fetus may be exacerbated through dietary choices.
As stated above, on a cellular level, epigenetic mechanisms may explain how maternal diet impacts fetal neurodevelopment. Next to evidence from rodent studies, there are accumulating human studies showing that poor prenatal diet can also have long-term effects on the human epigenome, predisposing the offspring for deleterious effects on the brain and mental health (Kofink et al., Reference Kofink, Boks, Timmers and Kas2013). For example, individuals from the Dutch Hunger Winter cohort who were exposed to nutrient deficiency periconceptionally showed decreased methylation of the insulin-like growth factor 2 (IGF-2) gene at the age of 60, compared to their unexposed same-sex siblings (Heijmans et al., Reference Heijmans, Tobi, Stein, Putter, Blauw, Susser and Lumey2008).
Finally, and in the context of this discussion about mechanisms, it is relevant to note that what the mother consumes in her diet cannot be equated to what the fetal brain is exposed to. Many factors and processes influence the potential effects on the fetal brain, such as the composition of the maternal intestinal microbiota (de Weerth, Reference de Weerth2017), maternal absorption of nutrients, metabolism and transport within the maternal body, transport across the placenta, transport within the fetal body, and the fetal blood–brain barrier (Monk et al., Reference Monk, Georgieff and Osterholm2013). Hence, the food consumed by the mother is only part of the equation regulating fetal neurodevelopment.
PNS and Exercise
Exercise: Evidence of associations
In nonpregnant adult populations, exercising regularly has been associated with better physical health and reduced risk for developing diseases, as well as lower levels of depression, anxiety, and cognitive problems (Marques et al., Reference Marques, Bjørke-Monsen, Teixeira and Silverman2015). Pregnancy comes with many bodily and physiological changes that include metabolic adaptations mostly aimed at meeting the high metabolic demands of the mother and fetus (Butte & King, Reference Butte and King2005; Melzer, Schutz, Boulvain, & Kayser, Reference Melzer, Schutz, Boulvain and Kayser2010). Despite these heightened metabolic needs, pregnant women benefit from regular physical activity in the same way that the nonpregnant population benefits from exercise. While women with high levels of occupational physical activity have a higher risk of giving birth preterm and having a child that is small for gestational age (Lee et al., Reference Lee, Symanski, Lupo, Tinker, Razzaghi and Chan2017), both the mother and the fetus benefit from regular moderate exercise during pregnancy (Melzer et al., Reference Melzer, Schutz, Boulvain and Kayser2010). Moreover, in healthy low-risk pregnancies, maternal, placental, and fetal adaptations are in place to protect the fetus from potential negative effects of maternal physical activity, such as hypoglycemia, decreased uterine blood flow, and increased temperature (for a discussion on these mechanisms, see Bø et al., Reference Bø, Artal, Barakat, Brown, Davies, Dooley and Khan2016). Positive effects of regular moderate exercise for the mother include decreased risk for obesity, gestational diabetes mellitus, and other pregnancy-related diseases; improved cardiovascular function; improved mood; decreased physical discomfort; decreased muscular cramps and lower-limb edema; shorter duration of labor; and decreased incidence of cesarean section (Bø et al., Reference Bø, Artal, Barakat, Brown, Davies, Dooley and Khan2016; Melzer et al., Reference Melzer, Schutz, Boulvain and Kayser2010; Sun, Hung, Chang, & Kuo, Reference Sun, Hung, Chang and Kuo2010). For the fetus, moderate maternal physical activity during pregnancy is associated with advanced neurobehavioral maturation, decreased fat mass, improved stress tolerance, and improved metabolic programming with lower risk for childhood obesity (Mathias et al., Reference Mathias, Elmhiri, de Oliveira, Delayre-Orthez, Barella, Tófolo and Abdennebi-Najar2014; Melzer et al., Reference Melzer, Schutz, Boulvain and Kayser2010; Mourtakos et al., Reference Mourtakos, Tambalis, Panagiotakos, Antonogeorgos, Arnaoutis, Karteroliotis and Sidossis2015).
Despite these findings, many women who regularly exercise stop exercising when pregnant or as pregnancy advances, and few nonexercising women take up exercising during pregnancy (Bø et al., Reference Bø, Artal, Barakat, Brown, Davies, Dooley and Khan2016; Melzer et al., Reference Melzer, Schutz, Boulvain and Kayser2010). A decrease in activity-related energy expenditure during pregnancy can be seen in both developing and developed countries, and few pregnant women comply with the recommended health guidelines for exercise during pregnancy, namely, ≥30 min of moderate physical activity on most, if not all, days of the week (Artal & O'Toole, Reference Artal and O'Toole2003; Melzer et al., Reference Melzer, Schutz, Boulvain and Kayser2010). While some socioeconomic and cultural factors appear to influence maternal choices about exercising during pregnancy, we unfortunately still know very little about what affects pregnant women's decisions to engage in health-promoting behaviors such as exercising (Auerbach et al., Reference Auerbach, Lobel and Cannella2014; Melzer et al., Reference Melzer, Schutz, Boulvain and Kayser2010).
The literature on relations between stress, depression and anxiety, and physical activity during pregnancy is fairly scarce. In one study, higher depressive symptoms were associated with having low and very low levels of activity during pregnancy (Teofilo et al., Reference Teofilo, Farias, Pinto, Vilela, Vaz, Nardi and Kac2014), while in another study, having higher levels of pregnancy-specific stress and anxiety was associated with less exercise during pregnancy (Lobel et al., Reference Lobel, Cannella, Graham, DeVincent, Schneider and Meyer2008). Other researchers found that pregnant women who exercised reported significantly less depressed mood, daily hassles, state-anxiety, and pregnancy-specific stress in the first and second trimesters, and less state-anxiety in the third trimester, compared to nonexercisers (Da Costa, Rippen, Dritsa, & Ring, Reference Da Costa, Rippen, Dritsa and Ring2003).
In addition, a few published studies have investigated how exercise programs affect pregnant women's mental well-being. In a recent randomized control trial (RCT) in which the control group was sedentary and the treatment group received at least 12 weeks of general fitness classes twice a week, pregnant women who exercised reported lower anxiety, sadness, and hopelessness, but also lower life enjoyment, than the control group (Haakstad, Torset, & Bø, Reference Haakstad, Torset and Bø2016). In another study, pregnant women attending exercise classes showed a larger reduction in pregnancy-specific anxiety for childbirth than women attending traditional childbirth classes (Guszkowska, Reference Guszkowska2014). Finally, a study in which previously sedentary pregnant women were offered a 4-week exercise program and given an accelerometer to monitor their physical activity rendered interesting results. The women who reached the Canadian guidelines of exercise during pregnancy at Week 4 of the intervention showed significantly greater reductions in depression and anxiety as well as in anger, tension, and fatigue than those who did not (Gaston & Prapavessis, Reference Gaston and Prapavessis2013). These intervention studies illustrate how increasing physical activity and exercise may result in positive changes in PNS, hence tentatively pointing at possible causal relations.
It is unclear why there are so few studies on how exercise programs in pregnancy affect women's mental and physical well-being. Possibly, one of the underlying reasons is that researchers are hesitant to use interventions with physical activity because of fears of unintended negative consequences for participating mothers. Although general guidelines for exercising during pregnancy exist (Bø et al., Reference Bø, Artal, Barakat, Brown, Davies, Dooley and Khan2016), recommendations are not very comprehensive nor specific, and maternal and fetal safety issues for exercise programs are not always clear. Most exercise intervention studies by far have investigated how yoga, popularly accepted as safe during gestation, affects the pregnant woman. For example, a 12-week twice-a-week yoga intervention reduced depression and anxiety more than standard prenatal care (Field et al., Reference Field, Diego, Hernandez-Reif, Medina, Delgado and Hernandez2012). In addition, in prenatally depressed women randomized to a 12-week weekly group yoga or group social support session, both groups showed reductions in depression and anxiety at the end of the intervention, as well as reductions in cortisol from pre- to postsession on the first and last days (Field, Diego, Delgado, & Medina, Reference Field, Diego, Delgado and Medina2013b). When depressed pregnant women were randomized to a 12-week weekly tai chi/yoga group instead of a waitlist control group, women showed reductions in depression, anxiety, and negative affect, as well as lower sleep disturbances, at the end of the treatment (Field, Diego, Delgado, & Medina, Reference Field, Diego, Delgado and Medina2013a). RCTs with an 8-week yoga program or treatment-as-usual for pregnant women with symptoms of anxiety and depression showed that yoga reduced pregnancy-specific anxiety for childbirth and prevented increases in depression in one study (Newham, Wittkowski, Hurley, Aplin, & Westwood, Reference Newham, Wittkowski, Hurley, Aplin and Westwood2014), and in another, that although symptoms decreased in both groups, negative affect decreased significantly more in the yoga group (Davis, Goodman, Leiferman, Taylor, & Dimidjian, Reference Davis, Goodman, Leiferman, Taylor and Dimidjian2015). In addition, daily practicing of yoga combined with deep relaxation from the middle of pregnancy till Week 36 of gestation, reduced depression and anxiety during pregnancy significantly more than when women practiced standard antenatal exercises that included simple stretching exercises, with anxiety increasing in this last group over pregnancy (Satyapriya, Nagarathna, Padmalatha, & Nagendra, Reference Satyapriya, Nagarathna, Padmalatha and Nagendra2013).
Yoga typically consists of stretching exercises and postures in combination with deep breathing and meditation. It is important to note that the activity level and energy expenditure of practicing yoga in pregnancy will very much depend on the specific program and style. For example, hatha yoga is the most practiced in Western cultures, power yoga is vigorous, and Bikram yoga is performed in a heated room and is not recommended for pregnant women (Babbar & Shyken, Reference Babbar and Shyken2016). Pregnancy yoga programs are often adapted versions of traditional yoga programs, of shorter duration or including only postures (e.g., Field et al., Reference Field, Diego, Delgado and Medina2013b). In addition, yoga classes typically contain periods of deep relaxation that other prenatal exercise programs do not include, but that may be fundamental in explaining potential effects of yoga on pregnant women. During deep relaxation, muscles are relaxed and breathing is voluntarily manipulated, becoming slow, deliberate, and deep (Babbar & Shyken, Reference Babbar and Shyken2016). This activates the parasympathetic nervous system, leading to decreases in blood pressure, heart rate, metabolic functioning, and oxygen consumption. These physiological effects may be beneficial for pregnant women (Babbar & Shyken, Reference Babbar and Shyken2016). Summarizing, the variations in yoga styles and the inclusion of breathing techniques mean that care must be taken when comparing the results of yoga interventions with those of other moderate physical exercise programs.
In sum, although moderate physical exercise has positive effects on the physical and mental well-being of pregnant women, as well as positive effects on the fetus, many pregnant women are sedentary or do not reach recommended pregnancy levels of physical activity. The paucity of studies investigating relations between PNS and exercise does not permit drawing conclusions on whether women suffering from PNS tend to exercise less. Indirect evidence for associations between PNS and exercise comes from exercise intervention studies in healthy pregnant women and in women with elevated depressive and anxiety complaints. The results of these studies clearly point in the direction of physical exercise and yoga helping to reduce PNS. However, this is not the same as evidence showing that PNS leads to less physical activity. Future longitudinal studies starting before conception and following women throughout pregnancy are needed to begin to uncover possible causal relations between PNS and exercise, and to unravel whether maternal physical activity may mediate programming effects of PNS on the fetus.
Exercise: Specific patterns of associations
With respect to our second question, there were overall few studies looking at associations between PNS and physical activity in pregnant women, making it difficult to draw conclusions on specific patterns of associations. Most reviewed findings showed that exercise reduced depression and anxiety, but few of the studies looked specifically at stress. Worthy of notice is the fact that two independent studies reported reductions in pregnancy-specific anxiety for childbirth in relation to increases in physical activity (Guszkowska, Reference Guszkowska2014; Newham et al., Reference Newham, Wittkowski, Hurley, Aplin and Westwood2014). On a speculative note, perhaps pregnant women increasing their physical activity feel stronger and become more confident that their bodies can physically handle childbirth, and this leads to the observed reductions in anxiety about childbirth.
Exercise: Potential underlying mechanisms
Research to date has mainly investigated the effects of physical activity, and not of inactivity, on body and mind. Consequently, in the current section, we will limit the discussion to trying to pinpoint the mechanisms behind the positive effects of engaging in pregnancy physical activity and exercise, on fetal and later child development. An important caveat to this discussion is that although physical inactivity and activity may be seen as two sides of the same coin, it would be unwise to assume that the mechanisms of how an inactive or sedentary pregnancy affect fetal development are simply the opposite of those behind the effects of physical activity. In other words, extreme inactivity during pregnancy may be paired with idiosyncratic physiological effects that cannot solely be explained by the lack of the effects of physical activity.
As stated before, physical activity during gestation appears to have many benefits for mother and fetus, including reduced gestational weight gain, risk of cardiovascular disease, risk of impaired glucose tolerance, and risk of preeclampsia, together with the development of innate immunity that could help prevent detrimental metabolic programming in the offspring (Genest, Falcao, Gutkowska, & Lavoie, Reference Genest, Falcao, Gutkowska and Lavoie2012; Marques et al., Reference Marques, Bjørke-Monsen, Teixeira and Silverman2015; Mathias et al., Reference Mathias, Elmhiri, de Oliveira, Delayre-Orthez, Barella, Tófolo and Abdennebi-Najar2014; Padmanabhan et al., Reference Padmanabhan, Cardoso and Puttabyatappa2016; Prather, Spitznagle, & Hunt, Reference Prather, Spitznagle and Hunt2012). Due to the paucity of research on exercise in pregnancy (Marques et al., Reference Marques, Bjørke-Monsen, Teixeira and Silverman2015), much of what is known about mechanisms underlying effects of physical activity comes from nonpregnant human adults and, often male, animal models. This should be kept in mind when interpreting many of the described mechanisms. The improved health exhibited by people who exercise regularly may be due to several biological mechanisms, including optimized neuroendocrine stress responses, increased cardiovascular fitness, an anti-inflammatory state, and increased growth factor expression and neuroplasticity (see review by Marques et al., Reference Marques, Bjørke-Monsen, Teixeira and Silverman2015). One of the mechanisms studied in pregnant women, and that may contribute to the positive effects of exercise, are increases in serum neurotrophic factors that regulate neurogenesis (Vega et al., Reference Vega, Kleinert, Sulprizio, Hollmann, Bloch and Strüder2011). As shown by these authors, acute exercise in the third trimester leads to increases of growth factors such as BDNF and IGF-1, which may have beneficial effects on the maternal brain, including improving mood and cognition.
Exercise-related improvements of maternal physical and psychological health may indirectly affect the fetus, with most of the positive physiological effects on the mother having positive effects on fetal development. For example, maternal complications such as preeclampsia can affect the fetus by producing fetal intrauterine growth restriction. Exercise can reduce such fetal complications through several maternal physiological pathways known to protect the fetus (Marques et al., Reference Marques, Bjørke-Monsen, Teixeira and Silverman2015). These include an anti-inflammatory state, characterized by low levels of pro-inflammatory cytokines and high levels of anti-inflammatory cytokines, and by improving endothelial function and diminishing vasoconstriction (Genest et al., Reference Genest, Falcao, Gutkowska and Lavoie2012). Maternal exercise has also been linked to improved viability of the placenta and reduced resting fetal heart rate, and increased gestational age at birth and lower birth weights (Prather et al., Reference Prather, Spitznagle and Hunt2012). With respect to the effects of maternal exercise on the offspring's neurodevelopment, one study found that neonates of exercising mothers had better 1-min Apgar scores, a measure of general infant health at birth (Haakstad & Bø, Reference Haakstad and Bø2011). Other studies report that the offspring of regularly exercising pregnant mothers have better neonatal orientation and self-soothing abilities, as well as higher 5-year-old general intelligence and oral language skills (Clapp, Reference Clapp1996; Clapp, Lopez, & Harcar-Sevcik, Reference Clapp, Lopez and Harcar-Sevcik1999). These early positive associations between maternal pregnancy exercising and offspring health and development may indicate a protective role of maternal exercise for offspring. However, research findings in this area are as yet too limited to be able to draw conclusions on potential effects of maternal prenatal exercise on offspring neurodevelopment (Marques et al., Reference Marques, Bjørke-Monsen, Teixeira and Silverman2015; Prather et al., Reference Prather, Spitznagle and Hunt2012).
An intriguing fact in this context is that physical activity such as aerobic exercise is known to lead to acute increases in cortisol, while chronic engagement in exercise leads to elevated basal cortisol levels in human adults and animals (Chen et al., Reference Chen, Nakagawa, An, Ito, Kitaichi and Kusumi2017). These elevations of cortisol with chronic exercise are similar to those of chronic stress and could be interpreted as a negative effect of exercise during pregnancy. However, while chronic cortisol elevations due to exercise are associated with decreases in depressive symptoms, increased memory and cognition, and improved brain neural plasticity, heightened cortisol due to chronic stress produces the opposite effects (Chen et al., Reference Chen, Nakagawa, An, Ito, Kitaichi and Kusumi2017). According to Chen et al., this apparent paradox could be explained by elevated cortisol due to different causes having different effects on the dopamine system, on stress reactivity to novel stressors, and on glucocorticoid and mineralocorticoid receptor regulation. For example, chronic cortisol elevations as a result of exercise lead to increases in dopamine, but those as a result of stress lead to decreases in dopamine, especially in the medial prefrontal cortex. Dopamine in this region of the brain is associated with active coping with stressors and with antidepressant effects. In addition, chronic stress is experienced as aversive, while chronic exercise is generally experienced as rewarding, and reward is known to acutely activate the midbrain dopamine system (see Chen et al., Reference Chen, Nakagawa, An, Ito, Kitaichi and Kusumi2017, for a further discussion). These and additional mechanisms may explain the positive effects of exercise on the mother and fetus described before. Note, however, that whether exercising regularly during pregnancy also produces chronically increased cortisol above the normal increases in cortisol throughout pregnancy (de Weerth & Buitelaar, Reference de Weerth and Buitelaar2005) is as yet unknown.
The possible positive effects of yoga exercise on the pregnant mother and fetus appear more straightforward, as studies to date report decreases in physiological stress as a result of engaging in yoga. For example, in pregnant women practicing yoga three times a week from pregnancy Week 20 until delivery, the acute effects of a yoga class at two time points in the third trimester were a significant reduction in salivary cortisol and alpha-amylase, a biomarker for sympathetic activity (Kusaka, Matsuzaki, Shiraishi, & Haruna, Reference Kusaka, Matsuzaki, Shiraishi and Haruna2016). In another study on women practicing prenatal hatha yoga, salivary cortisol was lower and positive affect higher on yoga compared to usual activity comparison days (Bershadsky, Trumpfheller, Kimble, Pipaloff, & Yim, Reference Bershadsky, Trumpfheller, Kimble, Pipaloff and Yim2014). Finally, in pregnant women with depressive symptoms, an acute physiological effect of a yoga session was a decrease in cortisol concentrations (Field et al., Reference Field, Diego, Delgado and Medina2013b). These studies are significant because they may be indicating that if PNS leads to a hypothesized decrease in physical activity and exercise, women may be missing regular opportunities to decrease daily cortisol concentrations, in what may constitute periods of recovery from daily stressors. However, the findings are limited to yoga exercises, which, as stated above, are known to include periods of deep relaxation that possibly lead to the observed decreases in cortisol. As discussed before, more vigorous physical activity leads to increases instead of decreases in cortisol. In addition, to date, studies examining exercise as a mediator of PNS and offspring outcomes are as yet unavailable.
Conclusions
In this review we sought out to find evidence for a heuristic model in which the effects of PNS on maternal physiology and fetal and later child development are mediated by lifestyle behaviors, more specifically sleep, diet, and exercise. The literature provided clear evidence for associations between maternal PNS, depression, and anxiety, and sleep disturbances, unhealthy diets, and less physical activity during pregnancy. In general, PNS was associated with negative lifestyle behaviors throughout pregnancy and not in a specific period of gestation. Stress, depression, and anxiety were all three related to these changes, although because of the often limited body of research, it was not always possible to pinpoint differences in the strength of specific relations. Finally, a plethora of potential underlying mechanisms linking lifestyle factors to maternal physical changes and fetal development could be described. These mechanisms showed some overlap between the three investigated lifestyle factors (e.g., inflammation was reported in all three), and include immune, neural, metabolic, endocrine, and physical pathways. This last observation is in line with the fact that different prenatal insults can lead to the same physiological change; for example, in animal models, both maternal undernutrition and gestational stress have been seen to lead to alterations in pregnancy androgen levels (Padmanabhan et al., Reference Padmanabhan, Cardoso and Puttabyatappa2016).
Discussion and Future Research
With the exception of RCT studies, most of the studies reviewed in this paper do not provide causal evidence for links between PNS and lifestyle behaviors. We can therefore not rule out the possibility that changes in lifestyle lead to PNS. For example, a systematic review by Lancaster et al. (Reference Lancaster, Gold, Flynn, Yoo, Marcus and Davis2010) showed that risk factors for prenatal depression were stress, anxiety, and smoking, a lifestyle behavior. This study also illustrates how different types of PNS may be intricately and complexly related, making it difficult to disentangle causal relations. Moreover, different models explaining the relations between PNS, lifestyle factors, and fetal programming exist. For example, according to one model, maternal stress would be the mediator between dietary perturbations and programming of the fetus (Entringer & Wadhwa, Reference Entringer and Wadhwa2013). In principle, models with either PNS or lifestyle factors as mediators would be valid. They may apply to different situations, such as famine leading to stress, or pregnancy-related anxiety leading to poor diet. In other words, although the heuristic model of this paper makes a case for lifestyle factors as mediators between PNS and fetal programming (Figure 1), this by no means implies that the direction of effects may not change depending on specific situations.
At the same time, thinking of inverse causal associations between lifestyle behaviors and PNS leads to the question of whether interventions on lifestyle behaviors may help reduce PNS. These pathways for intervening on maternal pregnancy psychological health have been suggested earlier (Vaz et al., Reference Vaz, Kac, Emmett, Davis, Golding and Hibbeln2013), and as we have seen above, pregnancy interventions with exercise and yoga have already shown promising results. Moreover, lifestyle behavior interventions to improve mental health have been used before in nonpregnant populations. For example, a 12-week dietary intervention in adults with major depression significantly reduced depressive symptoms, and was much more effective than social support (Jacka et al., Reference Jacka, O'Neil, Opie, Itsiopoulos, Cotton, Mohebbi and Berk2017). Very recently, a large RCT using an online sleep intervention program significantly improved sleep and reduced anxiety and depression in a student population suffering from insomnia (Freeman et al., Reference Freeman, Sheaves, Goodwin, Yu, Nickless, Harrison and Espie2017).
In pregnant women, higher zinc intake in diet appears to buffer the effects of maternal stress on pregnancy depression (Roy, Evers, Avison, & Campbell, Reference Roy, Evers, Avison and Campbell2010). Keenan, Hipwell, Bortner, Hoffmann, and McAloon (Reference Keenan, Hipwell, Bortner, Hoffmann and McAloon2014) showed that fatty acid dietary supplementation in pregnant African American women living in low-income environments resulted in lower reported perceived stress and lower cortisol reactivity to a laboratory stressor at 30 weeks of pregnancy. In addition, prenatal exercise training programs may be one of the most prominent nonpharmacological tools for optimizing fetal metabolic programming and preventing the future development of obesity, type 2 diabetes, and cardiovascular diseases (Mathias et al., Reference Mathias, Elmhiri, de Oliveira, Delayre-Orthez, Barella, Tófolo and Abdennebi-Najar2014). In sum, these types of prenatal lifestyle interventions may constitute one of the best gateways for indirectly diminishing levels of maternal PNS, while at the same time positively impacting maternal health and fetal neurodevelopment. Such interventions may therefore also contribute to the prevention of offspring neurodevelopmental and psychiatric disorders (Marques et al., Reference Marques, Bjørke-Monsen, Teixeira and Silverman2015) and hence be regarded as attractive and efficient alternatives or add-ons to existing PNS reduction programs. Combined nutrition and exercise intervention programs may produce the most desirable effects on mother and child (Mathias et al., Reference Mathias, Elmhiri, de Oliveira, Delayre-Orthez, Barella, Tófolo and Abdennebi-Najar2014), especially as there are indications that exercise may also positively impact sleep in pregnancy (Beddoe, Lee, Weiss, Kennedy, & Yang, Reference Beddoe, Lee, Weiss, Kennedy and Yang2010; Goodwin, Astbury, & McMeeken, Reference Goodwin, Astbury and McMeeken2000; Kolu, Raitanen, & Luoto, Reference Kolu, Raitanen and Luoto2014).
Several factors potentially relevant to understanding the role of lifestyle factors in PNS were not touched upon in the present review, but deserve to be mentioned. First, no attention was given to the role of fetal sex when reviewing the literature. Despite the fact that most of the studies do not report on the potential role of fetal sex, emerging studies are showing that sex may play an important role in fetal programming processes. Males and females appear to have differential vulnerabilities to programming (e.g., Carpenter, Grecian, & Reynolds, Reference Carpenter, Grecian and Reynolds2017; Khashan et al., Reference Khashan, Everard, McCowan, Dekker, Moss-Morris, Baker and Kenny2014), as well as differential influences on maternal physiology (Giesbrecht, Campbell, Letourneau, & APrON Study Team, Reference Giesbrecht, Campbell and Letourneau2015). Fetal sex may interact with maternal lifestyle behaviors, producing effects on maternal physiology that depend on the sex of the fetus. This factor should thus receive more attention in future empirical studies in the field. Second, ethnicity and cultural differences have not been studied in this context, while there are papers reporting, for example, that pregnant women from different ethnicities, but living in similar socioeconomic situations, report different prenatal stressors and show significant differences in biological markers (Borders et al., Reference Borders, Wolfe, Qadir, Kim, Holl and Grobman2015; Harville et al., Reference Harville, Savitz, Dole, Herring and Thorp2009). This underlines the importance of extending studies on maternal PNS to non-Western cultures and diverse ethnicities.
Third, few longitudinal studies include the preconceptional period in their designs, most probably because of pragmatic and logistic reasons. Nonetheless, the preconceptional period is fundamental for understanding the development of pregnancy, as many mental health and lifestyle problems are preexistent upon conception and may predispose women to negative pregnancy pathways (Laraia, Siega-Riz, Dole, & London, Reference Laraia, Siega-Riz, Dole and London2009). Pregravid weight, for example, is positively associated with stress, depression, and anxiety in early pregnancy (Laraia et al., Reference Laraia, Siega-Riz, Dole and London2009), and contrarily, pregravid physical activity is associated with fewer signs of gestational diabetes in early pregnancy (Baptiste-Roberts, Ghosh, & Nicholson, Reference Baptiste-Roberts, Ghosh and Nicholson2011). By designing future studies starting in the preconceptional period, and monitoring PNS, sleep, diet, and exercise from then on till delivery, changes over pregnancy can be monitored and attempts can be made at modeling interactions and change, distinguishing prospective causal pathways.
Fourth and finally, in the current review, the relations between PNS and the three studied lifestyle factors were discussed separately. This is an oversimplification of reality, as these factors are very often closely related. For example, in one study, occupational physical activity was weakly related to sleep duration and quality in late pregnancy (Borodulin et al., Reference Borodulin, Evenson, Monda, Wen, Herring and Dole2010), and in another, nighttime sleep disturbances were positively related to fat intake in the second and third trimesters (Chang et al., Reference Chang, Brown, Nitzke, Smith and Eghtedary2015). Future studies would do well in including measures of all three lifestyle factors in their designs and inspecting interrelations between all.
The points made above lead to the challenges now faced by research in this field. Carrying out the recommended lengthy longitudinal studies in which many types of data on lifestyle, mental, and physical health are collected from mother and infant, through diverse methodologies, such as observations, tests, self-reports, and biological samples, implies a large burden on researchers and participants alike. In order to reduce this burden, while at the same time increasing the objectivity, quality, and amount of data collected, new research methodologies need to be developed. For example, for assessing sleep and physical activity, wearable wireless devices such as smart textiles equipped with microsensors would constitute comfortable, nonintrusive methodologies that could collect ecologically valid data over longer periods of time in pregnancy. For dietary assessments, digital tools using a large collection of food probes and pictures of individual foods of different portion sizes may improve the reliability of more traditional food frequency questionnaires (Monk et al., Reference Monk, Georgieff and Osterholm2013). Alternatively, sophisticated digital photographic methods such as the Remote Food Photography Method (Martin et al., Reference Martin, Nicklas, Gunturk, Correa, Allen and Champagne2014) may decrease the burden while at the same time increase the objectivity of dietary reports.
Collecting large amounts of digital data over longer periods of time leads to data analysis challenges. Prenatal factors exert their influences in the context of one another, but this is not reflected in most studies on fetal programming (Monk et al., Reference Monk, Georgieff and Osterholm2013). Most studies are correlational and include a subset of potentially relevant variables. However, with technological advances will come ever-increasing digital databases with large numbers of variables. To truly take advantage of the data and do justice to the complexity of human life and development, we need to use complex statistical analyses. In this manner, we will begin to distill how maternal PNS, characterized by observations, self-report, and biomarkers, moderated by personality, genetics, experiences, fetal sex, and so on, and mediated through lifestyle behaviors, such as sleep, diet, teratogens, and exercise, leads to physiological changes and effects on the offspring. These models will allow us to unravel mechanisms and open the floor for the creation of successful tailor-made interventions, targeted at specific types of PNS and lifestyles.
Such complex models with dozens of variables require sufficient statistical power to be run. The prospect of having to collect such large longitudinal data sets for longer periods of time and on large groups of participants will be daunting for most research groups. One solution to this problem is to do single-subject meta-analyses of pregnant women from different cohorts and countries. This approach could also help combine data from different countries, cultures, and ethnicities, making the results more robust and representative. However, systematic reviews and meta-analyses today encounter large problems due to diversity of study designs and measures (Kim et al., Reference Kim, Bale and Epperson2015). For example, although a recent review by Andersson et al. (Reference Andersson, Hansen, Larsen, Hougaard, Kolstad and Schlünssen2016) reported that maternal PNS could be associated with atopic disease in the offspring, the diversity in study quality and methodologies made it very difficult for the authors to reach strong conclusions.
For future prospective studies on PNS and developmental programming, international expert agreement on basic research protocols and measures, together with recommendations on the best instruments to assess them, would aid in making the results of research efforts around the globe more comparable. Such an effort would inform research teams setting up new studies and facilitate future sophisticated data analyses with large data sets. An international expert meeting could serve to set up this “basic pregnancy PNS protocol” with a set of questionnaires and biological markers to be collected whenever possible. In the last 20 years or so, there have been many studies on PNS and programming that have collected many types of data on PNS and offspring outcome. Perhaps now is the time for pruning and choosing the instruments that best predict offspring development with minimal burden for both researchers and participants. A basic, minimal set of open-access instruments should be chosen so that data can be collected easily, correctly, and more widely than only in Western populations. In the future, the data resulting from studies using the same standard measures of PNS could also be poured into an international databank. Over the years, this would provide enough statistical power to test complex models, deepen our knowledge of PNS, and begin to tease out issues of causality. This approach would be game changing and immediately remove many of the obstacles faced by many researchers wanting to collect PNS data together with lifestyle data: participant burden, need of added power, child outcomes, and complexity of study designs (Monk et al., Reference Monk, Georgieff and Osterholm2013).
To conclude, this theoretical review has shown that sleep, diet, and exercise during pregnancy, albeit largely understudied, may have fundamental roles as mediators between PNS and maternal pregnancy physiology. Future well-powered, longitudinal studies on these important lifestyle factors are needed to uncover the exact nature of their roles in programming mechanisms of PNS on fetal and child development. Research in this area is warranted, as lifestyle factors may constitute promising gateways for interventions, also of a preventive nature, on maternal physical and mental well-being during pregnancy.