Human survival, economic activities and the exploitation and utilization of mineral resources all depend on the supply of energy. Currently, the primary energy production requirements are met using hydrocarbon resources, with crude oil still dominating global energy production. Because of the increase in consumption, the demand for fossil fuels is increasing continuously. Drilling in unconventional reservoirs can help to meet the world's energy demand.
Drilling fluids, also known as drilling muds, are generally recognized as the ‘blood’ in oil and gas well-drilling engineering and architectural engineering (Ma et al., Reference Ma, Chen and Qi2014). Drilling fluids are mainly composed of a liquid phase, a solid phase and additives (Fig. 1). The liquid phase is usually water (fresh water, brine), oil (crude oil, diesel oil) or emulsion (mixed-oil emulsion, reverse-phase emulsion). Drilling fluids with water as a continuous phase are called water-based muds (WBMs), and those with oil as a continuous phase are called oil-based muds (OBMs). In most drilling processes, WBMs are used, owing to the simplicity of their preparation low cost, environmental friendliness, cutting-based removal and fast formation/breaking-down rate. Oil-based muds are used in specific cases, such as high-temperature deep wells, high-angle directional wells and horizontal wells. The solid phase mainly includes clay minerals, cuttings and weighting agents. The additives are usually inorganic, organic or polymer compounds (Ma et al., Reference Ma, Chen and Qi2014).
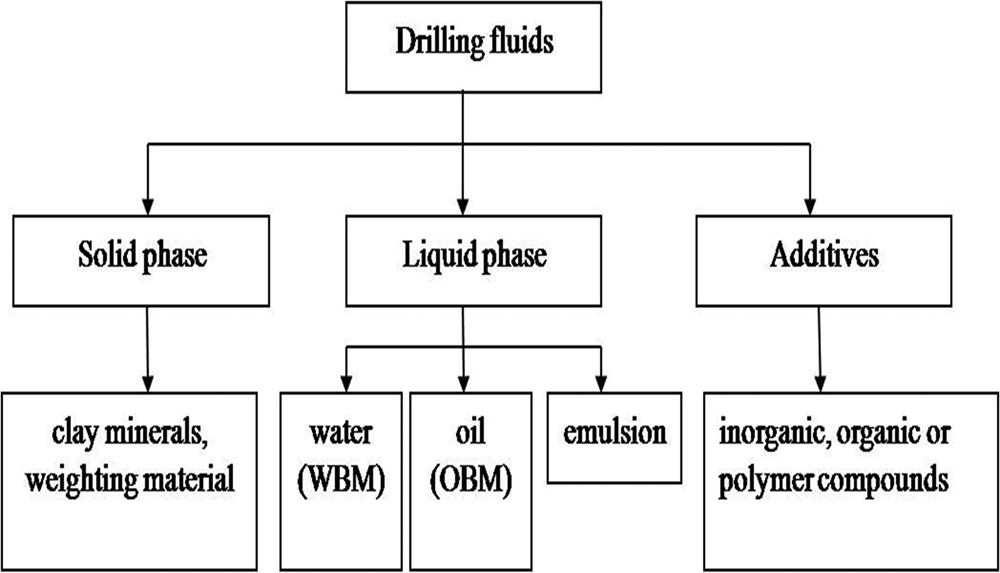
Fig. 1. Main components of drilling fluids.
Clay minerals are the basic constituents of the drilling fluids. They can build up and maintain a suitable viscosity at low solid levels and control the fluid loss throughout the drilling process (Omole et al., Reference Omole, Adeleye, Falode, Malomo and Oyedeji2013; Benslimane et al., Reference Benslimane, Bahlouli, Bekkour and Hammiche2016). For successful drilling, the selection and quantity of clay minerals are critical factors for achieving the required fluid properties (Al-Zubaidi et al., Reference Al-Zubaidi, Alwasiti and Mahmood2016). The clays used in drilling fluids are mainly divided into two types. The first type is bentonite, which consists mainly of montmorillonite (Mt). Sodium-bentonite contains 70–90 wt.% sodium-Mt and it has a high swelling capacity. Potassium ions are often used in these types of drilling formulations owing to their roles as swelling inhibitors (Li et al., Reference Li, Wu, Song, Lee, Jin and Ren2015; Barast et al., Reference Barast, Razakamanantsoa, Djeran-Maigre, Nicholson and Williams2017). The second type is the sepiolite/palygorskite (Pal) group (Wang & Wang, Reference Wang and Wang2016).
The properties of drilling fluids, namely the plastic viscosity, apparent viscosity, yield point, mud density, fluid loss, pH and gel strength, have been studied extensively. Rheological characterization is of great importance for clay minerals in drilling fluids, as they are often used in a variety of changing environmental conditions, such as changing pressure and temperature, and inflow through various geometrical shapes of a conduit (e.g. in pipes and strange annuli for oil and gas wells and in rivers and hills). Therefore, most studies focus on the rheological properties of the drilling fluids. The temperature, types of electrolytes, pH and concentration of clay minerals are important factors influencing the rheological properties of the drilling fluids (Afolabi et al., Reference Afolabi, Orodu and Efeovbokhan2017).
This work reviews comprehensively the factors that influence the rheological properties of drilling fluids containing clay minerals. It summarizes the application and research progress on the clay minerals used in drilling fluids and discusses the future prospects regarding the use of clay minerals in drilling fluids.
Bentonite-based drilling fluids
Bentonite is a type of clay consisting predominantly of Mt. The general structural formula of Mt in bentonite clays is (1/2Ca, Na)x(Al2–x, Mgx)(Si4O10)(OH)2nH2O (Afolabi et al., Reference Afolabi, Orodu and Efeovbokhan2017; Zhuang et al., Reference Zhuang, Zhang, Jaber, Gao and Peng2017c). Owing to their exceptional rheological properties, bentonite-based clays are usually the main solid components of drilling fluids, and they also function as viscosifiers for reducing water losses (Falode et al., Reference Falode, Ehinola and Nebeife2008).
A good-quality bentonite for drilling fluids should contain mainly Mt. Nevertheless, in practice, bentonites often contain other clay minerals (illite and kaolinite) and non-clay minerals such as quartz and feldspars, which have an adverse effect on bentonite quality (swelling capacity) (Abdou et al., Reference Abdou, Al-sabagh and Dardir2013). The main types of bentonite are Na-bentonite, which has a high swelling capacity that contains sodium-Mt at 70–90 wt.%, and Ca-bentonite, which will quickly generate colloids in water and is considered to be a non-swelling clay (Dill, Reference Dill2016; Yan et al., Reference Yan, Wang, Sun and Zhu2016).
Rheological models
Rheological models are important tools for investigating the rheological properties of dispersions. Previous works have studied the rheological behaviour (plastic viscosity, apparent viscosity, gel strength and yield stress) of bentonite-based drilling fluids. Two models are commonly used to explain the rheological behaviour of a clay dispersion: the Herschel–Bulkley model and the Bingham model.
The Herschel–Bulkley model is described in Eq. (1):

where τ is the shear stress (Pa), τ0 is the yield stress (Pa) and represents the magnitude of the shear stress needed to initiate flow, k is the consistency index (Pa) and represents the viscosity of a material at one reciprocal second, γ is the shear rate (s–1) and n is the flow index and represents the degree to which a material exhibits non-Newtonian flow behaviour.
In the Herschel–Bulkley model, n < 1 indicates that the apparent viscosity of the material decreases as the shear rate increases owing to shear thinning or pseudo-plastic behaviour. In addition, the shear thinning will increase as n approaches 0. When n > 1, the apparent viscosity increases as the shear rate increases; this is caused by shear-thickening or dilatant behaviour.
The Bingham model is described by Eq. (2):

where τ is the shear stress (Pa), τ0 is the yield stress (Pa), η is the plastic viscosity (Pa) and D is the shear rate (s–1).
The Bingham model also shows the yield stress (i.e. the amount of shear stress required to initiate flow); the value is similar to that in the Herschel–Bulkley model. It also provides the plastic viscosity, which is the viscosity of a material after yielding (Ko & Kang, Reference Ko and Kang2015). Baravian et al. (Reference Baravian, Vantelon and Thomas2003) effectively assessed the viscosity of these dispersions through the definition of an effective volume fraction. Using such an approach, they transformed the relationship between the viscosity and volume fraction (ηr = f(ϕ)) into a relationship that links the viscosity with both the flow and volume fraction (ηr = f(ϕ, Pe)) (Baravian et al., Reference Baravian, Vantelon and Thomas2003).
Some modes have been proposed for interactions between clay particles in drilling fluids. Van Olphen (Reference van Olphen1951, Reference van Olphen1956) suggested the formation of a three-dimensional ‘house of cards’ network, including three possible modes for associations of the Mt particles in aqueous bentonite dispersions: face-to-face (F–F), edge-to-edge (E–E) and edge-to-face (E–F). The E–F interactions are induced by electrostatic attractions between the opposite charges of the edges (+) and faces (–) of the particles and should dominate under acidic conditions. This has been confirmed by various rheological measurements (Ramos-Tejada et al., Reference Ramos-Tejada, Arrovo, Peren and Duran2001). However, it has also been clearly demonstrated that the system is repulsive, and that the house of cards model could not account for the behaviour of clay gels at low ionic strength. As the gel structure could be stabilized by the repulsive forces between the interacting electrical double layers of the platelets, a completely different view was proposed supported by measurements of interparticle forces and rheological properties (Norrish, Reference Norrish1954). Fractal or cluster associations have also been suggested as possible mechanisms for explaining the macroscopic structure of the gel based on various scattering (light, X-ray and neutron) and nuclear magnetic resonance experiments (Shalkevich et al., Reference Shalkevich, Stradner, Bhat, Muller and Schurtenberger2007; Benna-Zayani et al., Reference Benna-Zayani, Mgaidi, Stambouli, Kbir-Ariguib, Trabelsi-Ayadi and Grossiord2009; Paineau et al., Reference Paineau, Bihannic, Baravian, Philippe, Davidson, Levitz, Funari, Rochas and Michot2011a).
The first mode (F–F) results in thicker and larger particles in bentonite dispersions, and the E–E and E–F associations form a three-dimensional network similar to a house of cards structure (Benna et al., Reference Benna, Kbir-Ariguib, Magnin and Bergaya1999). Two possible mechanisms account for the gel formation of Mt: (1) the strong electrostatic attraction between the negatively charged faces and the positively charged edges (Heller & Keren, Reference Heller and Keren2001); and (2) the E–E and F–F interactions via long-range electrostatic double-layer repulsion (Callaghan & Ottewill, Reference Callaghan and Ottewill1974). These associations may account for the customizing of the rheological properties of bentonite dispersions, and they are affected significantly by the temperature, types of electrolytes, pH and smectite content in the bentonite. Finally, the rheological properties of bentonite dispersions also seem to depend on the magnitude and localization of the layer charges of smectites. Smectites with a high-layer charge and/or abundant tetrahedral charges (e.g. beidellites or nontronites) tend to form dispersions with inferior rheological properties, as compared to their counterparts with low-layer charges located in octahedral sites (Christidis et al., Reference Christidis, Blum and Eberl2006).
The role of temperature
Temperature has a significant influence on the rheological behaviour of bentonite suspensions through various mechanisms (Darley & Gray, Reference Darley and Gray1980): (1) physically, as an increase in temperature will decrease the viscosity of the liquid phase; (2) chemically, as hydroxides such as sodium hydroxide, calcium hydroxide or other additives may react with clay minerals above 90°C (although this has little influence on the rheological properties at low alkalinity, it may be important at high alkalinity); and (3) electrochemically, as an increase in temperature will increase both the ionic activity of the electrolyte and the solubility of any partially soluble salts present in the suspension. The resulting changes will substantially affect the degree of aggregation and/or the suspension of the bentonite particles in the medium. Most studies on the rheology of bentonite suspensions have been performed at room temperature. However, there also exists a limited number of studies that have investigated the effects of temperature on the rheological properties of bentonite suspensions. These studies are important to the drilling industry because of the extreme conditions that exist in environments such as high-temperature reservoirs and deep wells in which drilling fluids are used. Moreover, they contribute to our understanding of the behaviour of drilling fluids that undergo temperature changes during geothermal well-drilling operations and provide a database for improving the development of numerical wellbore simulators (Table 1).
Table 1. Research on the effects of temperature on the rheological behaviour of bentonite dispersions.

Hiller (Reference Hiller1963) examined the rheological behaviour of 4 mass% Na-bentonite dispersions (using Wyoming bentonite converted to Na-bentonite as a raw material) at high temperatures and observed that both the plastic viscosity and yield stress decreased with increasing temperature (up to 176°C) at low electrolyte concentrations. In addition, an increase in yield stress and a decrease in plastic viscosity were reported with increasing temperature at high electrolyte concentrations. Briscoe et al. (Reference Briscoe, Luckham and Ren1994) observed that the yield stress significantly increased with temperature, but the plastic viscosity decreased significantly at temperature up to 140°C for 7–10 mass% water-based Na-bentonite dispersions. Rossi et al. (Reference Rossi, Luckham, Zhu and Briscoe1999) investigated the effect of temperature on the rheology of sodium-Mt dispersions under various electrolyte conditions and reported that the yield stress increased with temperature, which was caused by the thermally induced swelling. Santoyo et al. (Reference Santoyo, Santoyo-Gutierrez, Garcia, Espinosa and Moya2001) evaluated the rheological behaviour of drilling fluids of four different (mainly sodium-Mt) bentonites. A small decrease in apparent viscosity was observed at temperatures up to 80–120°C and a drastic increase was observed at temperatures of 125–175°C (Santoyo et al., Reference Santoyo, Santoyo-Gutierrez, Garcia, Espinosa and Moya2001).
In drilling activities in deep-sea beds, where the ambient temperature is significantly lower than at the sea surface (i.e. only a few degrees centigrade), it is necessary to study the effects of temperature on the behaviour of clay suspensions. The yield stresses and dynamic moduli of commercial bentonite water suspensions at 3°C and 25°C were reported to increase with temperature, and the temperature-dependent behaviour became more evident as the concentration of clay increased (Lin et al., Reference Lin, Cheah, Phan-Thiena and Khoo2016).
As temperature is known to adversely affect the rheology of water-based bentonite suspensions, several additives are used to counteract these adverse effects. However, the exact mechanism by which higher temperatures lead to enhanced flocculation of Mt platelets is unclear. Vryzas et al. (Reference Vryzas, Kelessidis, Nalbantian, Zaspalis, Gerogiorgis and Wubulikasimu2017) performed a systematic study of the rheological properties of 7 mass% bentonite suspensions at temperatures of 25–80°C under atmospheric pressure. As the temperature increased, the shear stresses at low shear rates increased. The effect of temperature was much smaller at higher shear rates, and the Herschel–Bulkley rheological model fitted all of the data well. A standard methodology was proposed to complement the American Petroleum Institute (API) standards, and it provided consistent results (Vryzas et al., Reference Vryzas, Kelessidis, Nalbantian, Zaspalis, Gerogiorgis and Wubulikasimu2017).
The properties of drilling fluids that use bentonite for rheology control and water as the fluid are greatly and adversely influenced by temperature, and the degree of influence should be known with accuracy. However, as several variables are involved, the rheological behaviour of bentonite suspensions is unpredictable and incompletely understood at elevated temperatures. Most studies in the past used oil as the main liquid phase, as it was more stable at high temperatures. However, in view of the emerging need for environmental protection, and because of logistics and cost considerations, the best choices for deep-drilling applications are high-temperature-resistant WBMs. The conventional approach is to use additives to counteract the adverse effects of temperature on the rheological properties of the bentonite suspensions. The commonly used additives are anionic polyelectrolytes, which are bound on the surfaces of bentonite particles, thereby prohibiting the agglomeration of the clay particles and keeping the suspensions stable. Such anionic polyelectrolytes are lignosulfonate complexes with various types of metals, tannins, humic acids, lignites and synthetic polymers (Zhang & Yin, Reference Zhang and Yin2002; Luo et al., Reference Luo, Wang, Pei, Yu and Xi2018). However, the aforementioned additives have inherent shortcomings, as they can undergo thermal degradation at high temperatures (Wan et al., Reference Wan, Yao, Zishun, Li and Juan2011; Oyewole et al., Reference Oyewole, Salami and Plank2013; Dias et al., Reference Dias, Souza and Lucas2015).
Ionic liquids (ILs) are liquid organic salts at room temperature composed of positive and negative ions. They are considered to be ‘green chemicals’ because of their diversity, high thermal stability, selective dissolution and other advantages. They have attracted interest owing to their excellent properties, such as high thermal stability, ‘zero’ vapour pressure and compatibility with many types of materials (André et al., Reference André, Marsh, Shusheng and Staiger2009; Macfarlane et al., Reference Macfarlane, Tachikawa, Forsyth, Pringle and Howlett2014; Singh et al., Reference Singh, Singh and Chandra2014). Some studies have used ILs to improve the thermal stability of Mt-based drilling fluids, and the early studies mainly addressed the practical applications of IL-modified Mt (Kim et al., Reference Kim, Malhotra and Xanthos2006; Takahashi et al., Reference Takahashi, Takashi and Masayoshi2012). Luo et al. (Reference Luo, Pei, Wang, Yu and Chen2017) thoroughly investigated the influence of a water-soluble IL on the rheological and filtration properties of water-based drilling fluids under various experimental conditions. The rheological behaviour of the water-based drilling fluids was improved at high temperatures (up to 240°C) and low concentrations of IL (as low as 0.05 mass%). Moreover, the filtration volume at high temperatures and high pressures was reduced by as much as 52%. This demonstrated that the excellent rheological and filtration properties of water-based drilling fluids at high temperatures are caused by the high thermal stability and cation-exchange capacity of the ILs (Luo et al., Reference Luo, Pei, Wang, Yu and Chen2017). Ofei et al. (Reference Ofei, Bavoh and Rashidi2017) studied the effects of a 1-butyl-3-methylimidazolium chloride IL (BMIM-Cl) on the rheological and filtration properties of a WBM system in the temperature range of 25–200°C at a pressure of 1000 psi. The viscosity, solid suspension capacity and filtration behaviour of the drilling fluid were remarkably stabilized in the presence of BMIM-Cl, even at temperatures up to 180°C (Ofei et al., Reference Ofei, Bavoh and Rashidi2017). The inherent superior performances of ILs render them ideal for use as additives to drilling mud. However, additional insights regarding the detailed mechanisms thereof are necessary to better understand the influence of ILs on drilling muds.
The role of pH
Montmorillonite particles, which are the major components of bentonite, have negatively charged faces and edges, with the latter generally corresponding to <5% of the total surface area. The edges are positively charged at pH values below the isoelectric point and negatively charged at pH values above the isoelectric point of the edges (Delgado et al., Reference Delgado, Gonzalez-Caballero and Bruque1986). The isoelectric point of the smectite particles in the drilling fluids is the pH at which the smectite particles have zero charge (van Olphen, Reference van Olphen1977). The pH of the drilling fluids determines the type of Mt particle associations, endowing the system with viscosity and yield stress (Lagaly et al., Reference Lagaly and Ziesmer2003). Therefore, the effects of pH on the rheological behaviour of drilling fluids is a topic of considerable interest (Duran et al., Reference Duran, Ramos-Tejada, Arroyo and Gonzalez-Caballero2000; Ramos-Tejada et al., Reference Ramos-Tejada, Arrovo, Peren and Duran2001; Tombácz & Szekeres, Reference Tombácz and Szekeres2004; Laribi et al., Reference Laribi, Fleureua, Grossiord and Kbir-Ariguib2006).
Numerous studies have shown that the isoelectric point of the edges of Mt-based drilling fluids is in the range of 5.0–8.0 (Kelessidis et al., Reference Kelessidis, Tsamantaki and Dalamarinis2007). According to Heath & Tadros (Reference Heath and Tadros1983), the isoelectric point was estimated to be at a pH value of ~7, at which the system exhibited a minimum yield value and the residual viscosity was determined (Heath & Tadros, Reference Heath and Tadros1983). Benna et al. (Reference Benna, Kbir-Ariguib, Magnin and Bergaya1999) studied the effect of pH on the rheological behaviour of Na-bentonite suspensions. The yield stress decreased at a natural pH and it reached a minimum in a basic medium, whereas it increased and reached a maximum when the pH decreased (Benna et al., Reference Benna, Kbir-Ariguib, Magnin and Bergaya1999).
Although the effects of pH on the rheological behaviour of bentonite suspensions is of prime interest and many studies have been conducted thereon, a complete understanding of the effects of pH on the particle association and macroscopic structure of these suspensions is still elusive, especially for concentrated suspensions. Kelessidis et al. (Reference Kelessidis, Tsamantaki and Dalamarinis2007) investigated the influence of pH values in the range of 7.5–10.5 for 5.00% and 6.42% aqueous Wyoming bentonite suspensions, and they reported that the pH affected the type of Mt particle association. Moreover, the maximum yield stress, flow consistency index and apparent viscosity were determined at the natural pH of the suspensions. The shear stress showed an increasing trend with increasing pH values up to 9.1, and then decreased at higher pH values (Kelessidis et al., Reference Kelessidis, Tsamantaki and Dalamarinis2007). Choo & Bai (Reference Choo and Bai2015) also studied the effects of solution pH on the rheological properties and long-term stability of bentonite suspensions (Fig. 2). The absolute value of the ζ-potential decreased, and an initial stress value was observed at the initial shear rate value as an amount of 1 M HCl was added to the fresh suspension. However, the pH increased and the viscosity decreased after the suspensions were stored for 72 h with large amounts of added acid. The shear stress increased with the addition of up to 5 mL 1 M HCl (pH 7.3) and decreased with the addition of greater amounts of 1 M HCl (Choo & Bai, Reference Choo and Bai2015). Huang et al. (Reference Huang, Leong, Chen, Au, Liu and Qiu2016) evaluated the effects of pH on a mixed bentonite suspension consisting of 40.4% sodium-Mt and 16.0% sepiolite. The gel displayed very low or no yield stress at a pH value of 5.2 and a maximum yield stress at a pH value of 8.5. The ageing and/or structural recovery behaviours of the gels were most pronounced at high pH values (Huang et al., Reference Huang, Leong, Chen, Au, Liu and Qiu2016).

Fig. 2. Flow curves of the bentonite dispersions in HCl solutions (reprinted with permission from Choo et al. (2015), copyright Elsevier B.V.).
In the aforementioned studies, the effects of pH on Mt particle association and the resulting macroscopic structure that causes the rheological behaviour of these dispersions remain elusive for concentrated suspensions, which are the suspensions that meet industrial requirements. The view has been that, as long as the pH value of the mud is stabilized over a considerable range, the role of pH has not needed much attention; there has been a lack of in-depth research as a result.
The role of electrolytes
The edges of Mt particles are usually irregular (Keren et al., Reference Keren, Shainberg and Klein1988; Zou & Pierre, Reference Zou and Pierre1992). The particles may remain isolated or form thin packets and may fail to agglomerate in a dilute clay dispersion owing to the interaction of diffuse double layers (Bergaya & Lagaly, Reference Bergaya and Lagaly2013). Nevertheless, they may flocculate in the presence of electrolytes. Hence, the influence of electrolytes on the association of Mt particles has also been a critical topic of research in drilling fluids.
The addition of inorganic salts (especially NaCl) may strongly affect the rheological properties of bentonite-based drilling fluids. Numerous studies have evaluated the effects of the concentration of NaCl on the rheological behaviour of bentonite dispersions (M'bodj et al., Reference M'bodj, Kbir Ariguib, Trabelsi Ayadi and Magnin2004; Tombácz & Szekeres, Reference Tombácz and Szekeres2004; Duman & Tunç, Reference Duman and Tunç2009; Liang et al., Reference Liang, Long, Zhang and Yang2010). In this respect, E–F heterocoagulation might occur in NaCl solutions with concentrations >25 mM at a pH of 4, where a screening electric double layer of positively charged edges may emerge. A greater concentration of salt (~50 mM of NaCl) will induce E–F interactions between poorly charged edges and negatively charged faces around the point of zero charge of the edges (pH value of ~6.5). A transient coagulation of F–F homotype interactions appears after adding ≥0.1 M NaCl (Tombácz & Szekeres, Reference Tombácz and Szekeres2004). Thus, the addition of NaCl salts affects concentrated bentonite dispersions. The yield stress, flow consistency index and apparent viscosity were reported to decrease monotonously with increasing salt concentrations (up to 1.0 M) (Fig. 3) (Kelessidis et al., Reference Kelessidis, Tsamantaki and Dalamarinis2007).

Fig. 3. Flow curves of 6.42 wt.% Wyoming bentonite dispersions in electrolyte solutions (reprinted with permission from Kelessidis et al. (2007), Elsevier B.V.).
Miyahara et al. (Reference Miyahara, Adachi and Nakaishi1998) examined the maximum sedimentation rate as a function of the volume fraction of solids at a pH value of 10, with NaCl concentrations ranging from 0.5 to 1.5 M. The equivalent hydrodynamic diameter of the floc (d f) and increment ratio of the effective volume fraction of the particles (α) increased with the salt concentration, and the value of α agreed qualitatively with the values obtained from the rheological data (Batchelor, Reference Batchelor1977; Miyahara et al., Reference Miyahara, Adachi and Nakaishi1998). The observed increase in d f was ascribed to F–F association, as the vicinal platelets approach and form flocs with more irregular shapes with increasing salt concentrations (Miyahara et al., Reference Miyahara, Ohtsubo, Nakaishi and Adachi2001).
The influence of other types of electrolytes (BaCl2, CaCl2, KCl and Na2SO4) on bentonite dispersions has not received the same amount of attention as the influence of NaCl. Mpofu et al. (Reference Mpofu, Addai-Mensah and Ralston2005) investigated the influence of hydrolysable metal ions, Mn(II) and Ca(II), on the interfacial chemistry, particle interactions and dewatering behaviour of Mt clay dispersions in the pH range of 3–12, and they observed that the metal ions had a profound influence on the colloid stability, rheology and dewatering behaviour of unflocculated and flocculated Mt dispersions (Mpofu et al., Reference Mpofu, Addai-Mensah and Ralston2005). The addition of 0.02–0.2 M of electrolytes reduced the apparent viscosity of the bentonite dispersions and modified their rheological behaviour from shear thinning to Newtonian and shear thickening (Abu-Jdayil, Reference Abu-Jdayil2011). The divalent cation salts were more effective at reducing the apparent viscosity and yield stress of dispersions than the monovalent cation salts (Fig. 4) (Abu-Jdayil, Reference Abu-Jdayil2011). Duman & Tunç (Reference Duman and Tunç2009) investigated the electrokinetic and rheological properties of Na-bentonite dispersions in the presence of various electrolyte solutions, including LiCl, NaCl, KCl, NH4Cl, NaClO4, CH3COONa, NaNO3, Na2SO4, Na3PO4, CuCl2, MnCl2, CaCl2, BaCl2, NiCl2 and AlCl3. The divalent cations (Cu2+, Mn2+, Ca2+, Ba2+ and Ni2+) and trivalent cation (Al3+) controlled the surface potential of the smectites in the Na-bentonite dispersions. Trivalent cations such as Al3+ changed the surface charge of the Na-bentonite from negative to positive (Duman & Tunç, Reference Duman and Tunç2009). Recent research has confirmed that the charge location might have a significant impact on the phase behaviour of swelling clay minerals. Paineau et al. (Reference Paineau, Bihannic, Baravian, Philippe, Davidson, Levitz, Funari, Rochas and Michot2011a) investigated the structures of clay platelet dispersions with various charge locations at various ionic strengths using small-angle X-ray scattering experiments and transmission electron microscopy (TEM), and they proposed that stronger electrostatic repulsions might improve the liquid-like positional local order. These authors also conducted a comprehensive investigation of the rheology behaviour of various natural colloidal clay minerals in aqueous dispersions under both dynamic and steady-state conditions. The viscoelastic properties were closely related to repulsive electrostatic interactions between the platelets and the charge localization of the clay structures in such systems. In addition, new insights were provided on sol–gel transitions, which depend on the size of clays (Paineau et al., Reference Paineau, Michot, Bihannic and Baravian2011b).

Fig. 4. (a) Effect of NaCl concentration on the flow curves of bentonite. (b) Comparison between the effects of monovalent and divalent electrolytes on the flow curves of bentonite (reprinted from Abu-Jdayil (2011), Elsevier B.V.).
The effects of salt concentrations on the sedimentation behaviour of Mt dispersions is still not well understood. Wu & Adachi (Reference Wu and Adachi2016) studied the sedimentation behaviour of semi-dilute dispersions of Mt in a flocculation regime as a function of ionic strength at pH values of 4.0 and 9.5. The maximum velocity of the boundary between the supernatant and sediment and the ultimate sediment height were strongly affected by the salt concentration (Wu & Adachi, Reference Wu and Adachi2016). In addition, a few authors have analysed the morphological aspects of Mt through various techniques (scattering, cryo-TEM, X-ray microscopy and others). Hence, Ahmad et al. (Reference Ahmad, Kamal and Al-Harthi2018) studied the effect of an electrolyte (0.1 M NaCl) on the rheological and morphological properties of a bentonite dispersion and noted that electrolyte-treated bentonite dispersions have inferior rheological properties as compared to the base bentonite dispersion. Moreover, the particle morphology was dominated by the aggregation of Mt platelets (Ahmad et al., Reference Ahmad, Kamal and Al-Harthi2018).
The effect of bentonite concentration
The fact that the bentonite concentration is important is evident from a rheological standpoint. Starting with the Einstein equation, it has long been known that the volume fraction affects viscosity. A high clay concentration would slowly lead to the formation of a continuous gel structure as the suspended particles orientate towards the positions of minimum free energy according to Brownian motion. Large clay contents in water-based drilling fluids may be detrimental to drilling operations, leading to problems such as a reduction of the drill bit penetration rate, precipitation of a thick filter cake, differential pipe sticking and formation damage. Therefore, the clay concentration is an important factor that may influence the length of time required for the gel to reach maximum strength (Luckham & Rossi, Reference Luckham and Rossi1999).
The bentonite content affects the rheology of drilling fluids in HTHP conditions; the greater the bentonite content, the more inferior the rheological properties of the drilling fluids, although the bentonite content does not affect the shear rate and shear stress (Shi et al., Reference Shi, Li, Zhang, Zhang and Liang2008). Abu-Jdayil (Reference Abu-Jdayil2011) investigated the rheological behaviour of three types of bentonites at various bentonite concentrations. The bentonite concentration and bentonite type had significant impacts on the Newtonian, Bingham plastic and shear thinning behaviour of bentonite dispersions (Abu-Jdayil, Reference Abu-Jdayil2011). In a similar study, the viscosity and shear stress of bentonite dispersions increased with increasing bentonite concentration, and a considerable initial stress was observed at the initial shear rate as the storage time increased (Choo & Bai, Reference Choo and Bai2015).
The aforementioned studies refer to the rheological behaviour of bentonite-based drilling fluids at various temperatures, pH values, electrolytes and concentrations. In contrast, there have been relatively few studies addressing the rheological behaviour of locally formulated bentonite-based drilling fluids in challenging environments, such as in deep offshore drilling and under HTHP conditions. In addition, the thermal stability of these clays after beneficiation has not been well documented; this is a major concern regarding clays used in drilling fluids. Previous studies have focused on the upgrading of Ca-bentonite, usually with chemical treatment by Na2CO3. A limited number of studies has been performed on the rheological properties of Ca-bentonite dispersions at various pH levels, salt contents and in the presence/absence of polymeric additives (Karagüzel et al., Reference Karagüzel, Çetinel, Boylu, Çinku and Çelik2010).
The structure of the clay (particle size and shape) is crucial for a correct understanding of the rheological features. Some studies have attempted to determine the links between the structure of the clay and the rheological properties of drilling fluids. The rheological behaviours of pure mineral-colloid systems, the clay sizes and shapes of which changed systematically, were studied by ten Brinke et al. (Reference ten Brinke, Bailey, Lekkerkerker and Maitland2007) and they provided a physical model for describing the observed behaviours. In another study, the rheological modifications in mixed anisometric colloid systems were investigated and the mixtures displayed a complex “yield space” transition (ten Brinke et al., Reference ten Brinke, Bailey, Lekkerkerker and Maitland2008). The physicochemical factors of the transition were discussed and a simple qualitative model was described. This study provided a quantitative model-system baseline for mixed colloidal dispersions (ten Brinke et al., Reference ten Brinke, Bailey, Lekkerkerker and Maitland2008). Philippe et al. (Reference Philippe, Baravian, Bezuglyy, Angilella, Meneau, Bihannic and Michot2013) investigated the rheological evolution of size-selected natural swelling clay minerals from isotropic liquids to weak nematic gels. An effective approach to assessing the viscosity of the dispersions was used, and the relationship between the viscosity and volume fraction (ηr = f(ϕ)) was transformed into a relationship that linked the viscosity with the flow and volume fraction (ηr = f(ϕ, Pe)) (Philippe et al., Reference Philippe, Baravian, Bezuglyy, Angilella, Meneau, Bihannic and Michot2013).
Palygorskite-based drilling fluids
Palygorskite is a hydrated magnesium aluminium silicate clay mineral with the theoretical formula of Si8Mg8O20(OH)2(H2O)4⋅4H2O (Giustetto & Chiari, Reference Giustetto and Chiari2004). It has fibrous habit with fibre lengths of 0.2–2.0 mm, widths of 10–30 nm and thicknesses of 5–10 nm (Fig. 5) (Zhuang et al., Reference Zhuang, Zhang, Jaber, Gao and Peng2017c). Palygorskite has excellent colloidal properties, including high-temperature resistance, salt and alkali endurance and high adsorbing capabilities (Galan & Singer, Reference Galan and Singer2011). Therefore, Pal is often used as a thixotropic agent and viscosity controller, and it may act as an inhibiting agent for circulation loss in oil-well drilling fluids (Darley & Gray, Reference Darley and Gray1988).

Fig. 5. (a) The structure and (b) an SEM image of palygorskite (Pal) (reprinted with permission from Zhang et al. (2017), Elsevier B.V.).
The use of Pal in water-based drilling fluids and its gel structures and properties in aqueous systems have been studied. Neaman & Singer (Reference Neaman and Singer2000) investigated the rheology of aqueous suspensions of Pal with various particle morphologies as a function of clay concentration, adsorbed ions, pH and electrolyte concentration using a rotary viscometer. The rheological parameters (plastic viscosity, Bingham yield value and apparent viscosity) of Pal suspensions depend on the aspect ratio (length:width) of the Pal fibres, with the plastic viscosity increasing linearly with the clay concentration up to 5% w/v (Neaman & Singer, Reference Neaman and Singer2000). Both the morphology and the surface charge of the particles have major effects on the suitability of a drilling fluid; a rounded shape and a ζ-potential close to the isoelectric point favour particle aggregation, resulting in low-viscosity dispersions (Baltar et al., Reference Baltar, Luz, Baltar, Oliveira and Bezerra2009). In addition, with an increasing concentration of the dispersed phase in the Pal suspensions, the areas in the flow curves near the critical concentration (ϕcr; which indicate the onset of the rearrangement of the dispersion structure) are identified, and a sharp change occurs in the character of their flow in the immediate vicinity of ϕcr (Khil'Ko & Titov, Reference Khil'Ko and Titov2002). Finally, the rheological properties of Pal dispersions are not affected by the presence of electrolytes, in contrast to bentonites (Christidis et al., Reference Christidis, Katsiki, Pratikakis and Kacandes2010)
With the increasing importance of environmental protection and the development of petroleum exploration research, oil-based drilling fluids have attracted significant research and application interest owing to their excellent temperature resistance, good lubrication, shale inhibition, high reservoir protection and environmental friendliness. Organo-clay minerals may reduce the filtration loss and increase the viscosity and shear stress of drilling fluids, and their performance directly affects the rheology and filtration properties of drilling fluids. Hence, organo-clay minerals are important additives to oil-based drilling fluids. Organo-Mt is subject to thermal degradation and thermal thinning, permanently destroying its capacity to develop viscosity. Therefore, Pal has gained significant attention with regards to its use in oil-based drilling fluids owing to its high thermal stability and salt resistance (Farrow et al., Reference Farrow, Rasmussen, Menking and Durham2003; Dino & Thompson, Reference Dino and Thompson2013; Mainye & Teutsch, Reference Mainye and Teutsch2015).
Organo-Pal has exhibited excellent rheological properties and thermal stability in oil-based drilling fluids. The microstructures and properties of Pal in OBMs are influenced by the surface properties and organization of Pal. In addition, both Mt and Pal are suitable for use in oil-based drilling fluids, with the former providing excellent rheological properties and the latter providing good thermal stability (Zhuang et al., Reference Zhuang, Wu, Zhang, Zhang, Zhang and Liao2017a, Reference Zhuang, Zhang, Gao, Zhang and Liao2017b, Reference Zhuang, Zhang, Jaber, Gao and Peng2017c). However, the gel structure and gel formation of organic Pal in oil-based drilling fluids have not been studied extensively. It is suggested that investigating these issues may promote the application of Pal in oil-based drilling fluids and consequently improve drilling engineering.
Sepiolite-based drilling fluids
Sepiolite is a nanofibrous hydrous magnesium silicate, with an ideal unit-cell formula of Si12Mg8O30(OH)4(OH2)4(H2O)8 (Suárez & García-Romero, Reference Suárez and García-Romero2011). Owing to its fibrous morphology, intra-crystalline channels and small particle size, sepiolite has the largest specific surface area (up to 900 m2 g–1) among clay minerals. Moreover, its unique microporous channels (0.30–1.06 nm) lead to it having the strongest adsorption capacity among clay minerals. Therefore, sepiolite has been used frequently as a drug and catalyst carrier (Núñez et al., Reference Núñez, Gallego, Pastor and Merino2014), adsorbent (Rytwo et al., Reference Rytwo, Rettig and Gonen2011; Duman et al., Reference Duman, Tunç and Polat2015) and inorganic fibre, among other applications (Zheng et al., Reference Zheng, Zhang, Lan and Yu2011; Xu et al., Reference Xu, Wang and Wang2013). It is stable up to 260°C and in a saturated saline-water phase owing to its structure (Altun & Serpen, Reference Altun and Serpen2005). Sepiolite is also used in drilling fluids as a rheological additive, as it exhibits great thermal stability, excellent salt resistance and excellent rheological properties in water-based drilling fluids (Abdo et al., Reference Abdo, Al-Sharji and Hassan2016).
Similar to Pal, organo-sepiolite may potentially develop excellent rheological properties in oil-based drilling fluids. Hence, the preparation of organo-sepiolite has been reported and has attracted significant attention; however, the interest has mainly been focused on the preparation of sepiolite–polymer nanocomposites (Tartaglione et al., Reference Tartaglione, Tabuani and Camino2008; Garcia-Lopez et al., Reference Garcia-Lopez, Fernandez, Merino and Pastor2013; Fernandez-Barranco et al., Reference Fernandez-Barranco, Kozioł, Skrzypiec, Rawski, Drewniak and Yebra-Rodriguez2016), which do not meet the requirements of drilling fluids. Recently, the structures and properties of organo-sepiolite in oil-based drilling fluids were studied using X-ray diffraction, scanning electron microscopy, Fourier-transform infrared spectroscopy, thermal analysis and surface contact-angle analysis (Weng et al., Reference Weng, Gong, Liao, Lv and Tan2018; Zhuang et al., Reference Zhuang, Zhang and Chen2018a, Reference Zhuang, Zhang, Yang and Tan2018b). A sepiolite modified with surfactants had high thermal stability and exhibited excellent rheological properties in oil-based drilling fluids (Fig. 6). These studies strongly suggest that organo-sepiolite is a potential rheological additive in oil-based drilling fluids, with excellent rheological properties.

Fig. 6. The structure of sepiolite and the possible positions of organic surfactants; OSep = organo-sepiolite (reprinted with permission from Zhuang et al. (Reference Zhuang, Zhang and Chen2018a), Elsevier, Inc.).
Composite clay minerals in drilling fluids
All bentonite clays may develop a high-yield-stress gel at a low concentration (~5 wt.% solids), irrespective of the source. Nevertheless, bentonite-based drilling fluids (in Na or Li form) have limitations because of flocculation and instability problems at HTHP conditions. In this regard, Pal has excellent colloidal properties, such as high-temperature endurance, salt and alkali resistance and high adsorbing capacity (Lagaly, Reference Lagaly1989). In contrast, kaolin clays have a low swelling capacity, and their suspensions display significant yield stresses, and viscosity only at high concentrations (>40 wt.% solids; Teh et al., Reference Teh, Leong, Liu, Fourie and Fahey2009; Shanker et al., Reference Shanker, Teo, Leong, Fourie and Fahey2010). Hence, the mixing of the clay minerals may control and tailor the rheological properties for given applications, such as for use in high-density drilling muds or in impermeable slurry walls. Bailey et al. (Reference Bailey, Lekkerkerker and Maitland2015) summarized the current status of research in the area of mixed suspensions of clays, focusing on phase behaviour and rheological properties.
The addition of small amounts of bentonite to kaolin clay dispersions might drastically modify their rheological properties. Indeed, in the Hanford River Protection Program, bentonite was used to tailor the kaolin clay rheology to achieve a specific yield stress and plastic viscosity (Daniel & Eibling, Reference Daniel and Eibling2005). The mixed bentonite–kaolin drilling fluid had a greater viscosity at a pH value of 4 than at a pH value of 7 owing to positive–negative charge interactions between the various clay particles (Keren, Reference Keren1989). Kaolin clay may also be added to bentonite-based drilling fluids to increase the slurry density and control the rheological behaviour. Increasing the kaolin content reduces the slurry viscosity and increases its density (Adebayo & Ajayi, Reference Adebayo and Ajayi2011). Unfortunately, the pH of the slurries, which is expected to be important, was not measured in the aforementioned studies, and the microstructures formed in these mixed slurries that are responsible for the viscosity properties remain unclear. Moreover, the bentonite content determined the magnitude of the yield stress of the kaolin–bentonite composite dispersions, whereas the kaolin clay determined the pH value of the maximum yield stress (Au & Leong, Reference Au and Leong2013).
The properties of mixed Mt–Pal dispersions have also been studied. A significant improvement in rheology was observed when using Pal as an additive in small amounts with Mt and water. Palygorskite may tailor the properties of drilling fluids by reducing the particle size of the solid phase. Moreover, the addition of small amounts of Pal may impart to the drilling fluid considerable stability under HTHP conditions (Abdo & Haneef, Reference Abdo and Haneef2013). Chemeda et al. (Reference Chemeda, Christidis, Tauhid Khan, Koutsopoulou, Hatzistamou and Kelessidis2014) examined the effects of bentonite addition on the rheological behaviours of Pal and sepiolite. The proportion of the smectite in the mixture, the layer charge and the charge localization of the smectite influenced the apparent viscosity and yield point of the dispersions. Low-charge and high-charge smectites tended to improve and deteriorate the yield point and apparent viscosity of the dispersions, respectively (Chemeda et al., Reference Chemeda, Christidis, Tauhid Khan, Koutsopoulou, Hatzistamou and Kelessidis2014). Thus far, most of the studies on the use of mixed clays in drilling fluids have addressed specific aspects and have not produced a complete picture or understanding of their behaviour. As a result, the surface chemistry–microstructure–property relationships of mixed-clay dispersions require further study.
Conclusion
Drilling fluids provide a significant-market for bentonite, Pal and sepiolite. The important role of clays in drilling muds relates to their ability to achieve suitable viscosity at relatively low solid concentrations and to maintain the desired viscosity throughout a drilling operation.
Water-based drilling fluids are the most widely used, accounting for 90% of all such fluids at present. Bentonite has always been the basis of water-based drilling fluids. However, bentonite-based drilling fluids have limitations with regards to flocculation and instability under HTHP conditions. Owing to its swelling characteristics and intrinsic crystal-chemical heterogeneity, Mt is heterogeneous and causes flocculation problems. This results in insufficient and inconsistent rheological properties in drilling fluids.
Oil-based drilling fluids have broad application prospects in deep wells and complex drilling engineering. The excellent colloidal properties of Pal and sepiolite (e.g. specific dispersion features, high-temperature endurance, salt and alkali resistance and high adsorption capacity) impart to Pal and sepiolite unique physical and chemical properties, thus attracting the interest of the oil-based drilling industry.
There are numerous reports on clay mud rheology that, for a given clay, may enable the extraction of a relatively good qualitative model for explaining the rheological behaviour of the clay. However, there is still a need for an accurate predictive model based on clay-particle properties. Moreover, with the emergence of new drilling fluid products, it is necessary to define, judge and evaluate clays in accordance with the corresponding new standards (nano-scale and performance verifications of nano-drilling fluids, accurate quantitative determination methods of water-based drilling fluid film efficiencies, etc.). With the development of deep wells, complex wells, special technology wells and special reservoirs, as well as with the attention now being paid to environmental protection, the requirements for drilling fluids are increasing. Therefore, high-temperature resistance, high-pressure resistance, complex formations in deep wells and reservoir protection are still important research directions for drilling-fluid technology.
Financial support
This research was supported by the Zhejiang Provincial Natural Science Foundation of China (LQ18E030014, LQ18E030013 and LQ19F050004), the Zhejiang Provincial College Student Science and Technology Innovation Activity Plan (Xinmiao Talent Plan), the National Natural Science Foundation of China (41672033), the open fund from the Key Laboratory of Clay Minerals of the Ministry of Land and Resources of the People's Republic of China and the Engineering Research Center of Non-metallic Minerals of Zhejiang Province, Zhejiang Institute of Geology and Mineral Resource, China (ZD2018K04).