The finding of a measurable fluid-filled space at the back of the fetal neck between 11 and 14 weeks gestation, and the association between an abnormal measurement and fetal malformation, is, to my mind, one of the currently most intriguing mysteries in medicine. The story began with the publication from Benacerraf et al.1 in 1988 of the association between the measurement of the nuchal fold and Down's syndrome. Benacerraf et al., in a series of 5500 fetuses examined after 16 weeks of gestation, found that combining the measurement of the nuchal fold with the femoral length identified three-quarters of cases of Down's syndrome. About this time, particularly in the United Kingdom, there was an increasing demand from women over 35 years of age for sampling of the chorion villus in the first trimester, in order to obtain an earlier diagnosis of Down's syndrome, instead of waiting until 16 weeks for amniocentesis. When sampling the placenta in this group of patients, Nicolaides et al.2 noticed that some fetuses were hydropic, and showed increased nuchal fluid or nuchal oedema. Subsequently, the relationship of this finding to an eventual diagnosis of trisomy 21 was recognised. Nicolaides et al.2 had observed that, of 827 fetuses examined between 10 and 14 weeks, the incidence of chromosomal abnormalities overall was 3%, whereas, if the nuchal measurement was greater than or equal to 3 millimetres, the incidence was 35%. Subsequently, nuchal translucency became the preferred term used to describe this measurement. Although there is a slight variation with the length from crown to rump, in the fetus between 11 and 14 weeks of gestation, the 95th centile of the normal measurement is around 2.5 millimetres, with the 99th centile at 3.5 millimetres (Fig. 1).3 Intrigued by this finding, subsequent review of the early literature on trisomy 21 revealed the observation by Langdon Down, when describing this particular group of patients in 1866, who said that “The skin is deficient in elasticity giving the appearance of being too large for the body. The face is flat and the nose is small.” Now, 140 years later, all these features can be used in the ultrasonic detection of the syndrome in early fetal life. A point of additional interest is that hydrops, at a comparable gestational age, is a feature of the mouse with trisomy 16. This animal, although not an ideal model for humans with trisomy 21, shares many similar characteristics, and has been used extensively in embryological research into trisomy 21.

Figure 1. (a) A fetal specimen is seen after interruption of pregnancy with the membranes intact. The translucent fluid-filled area at the back of the fetal neck can be clearly seen. (b) A 12 week fetus is seen on ultrasound. The measurement of the nuchal translucency is markedly increased.
Further experience with the phenomenon of increased nuchal translucency, demonstrated that when the chromosomes were normal, there was an increased incidence of other fetal malformations, in particular congenital cardiac disease, but also apparently unrelated defects, such as skeletal anomalies, diaphragmatic hernia, and an ever-increasing list of genetic syndromes.4 At first sight, it appears difficult to understand any possible association between such unrelated defects as cardiac and skeletal malformations. A study of research in the animal model, however, suggests that this may be explicable. Over 60 years ago, diaphragmatic hernia was found in the offspring of rats who were fed a diet deficient in vitamin A during pregnancy.5 Subsequently, congenital cardiac malformations were detected by Wilson and Warkany6 in a similarly deprived group of rats. Later experimentation on pregnant rats using the poison nitrofen, at a critical period of embryological development, led to the induction of diaphragmatic hernia or congenital cardiac disease7 in one study, and skeletal anomalies in another.8 Bisdiamine, another poison extensively investigated in particular by Japanese workers, is known to induce anomalies of the ventricular outflow tracts in rats.9 The mode of action of both these poisons is through the inhibition of retinal dehydrogenase-2, the enzyme which forms retinoic acid from retinaldehyde, or vitamin A.10 A recent paper demonstrated a low level of retinol at birth in neonates with diaphragmatic hernia.11 It might be of interest to test levels of retinol in neonates with congenital cardiac malformations. In the rat model at least, nonetheless, the same insult can induce disparate malformations in different systems, with the type of malformation depending on the timing and intensity of the insult, or dose of the poison, and the genetic background. It also appears to be true that, in the animal model at least, different insults can produce the same anomaly.
Nuchal translucency and congenital cardiac malformations
The strength of the association of congenital cardiac disease with increased nuchal translucency was reported by Hyett et al. in 1999,12 when these workers found that over half of the mothers producing offspring with major congenital cardiac malformations in a cohort of over 29,000 pregnancies was associated with an increased nuchal translucency. The ascertainment of the congenital cardiac malformations in the population studied was only 1.7 per 1000 livebirths, suggesting that many cases of congenital heart disease were not detected in the group studied. Similar later studies, which were based on the local population of a single hospital, found rates of 1713 and 36%,14 respectively. The ascertainment of congenital heart disease in the first of these studies13 was about expected, at 3.5 per 1000, whereas it was only 1.7 in the latter study. Although these two studies appear to be adequate in terms of numbers, with over 7000 and 6000 patients respectively, the number of cases of congenital cardiac disease is insufficient to be reliable. The correct figure for the proportion of major congenital cardiac anomalies associated with increased nuchal translucency may lie somewhere in between, at about 25%.15
The rate of congenital cardiac disease increases concomitant with the increase in nuchal translucency. When the nuchal translucency is between 2.5millimetres and 3.5 millimetres, the rate is 2%, over twice the normal rate. Between 3.5 millimetres and 4.5 millimetres, it is 4%, between 4.5 millimetres and 5.5 millimetres, 6.5%, between 5.5 millimetres and 6.5 millimetres, 14%, and over 6.5 millimetres, it rises to 26%.16 Those patients with an increased nuchal translucency now constitute the most important group of our current referrals for fetal echocardiography deemed to be at high risk, yielding one-third of our fetal cardiac anomalies. Our policy is to scan the fetus at the time of detection of a nuchal translucency above 3.5 millimetres, therefore between 11 and 14 weeks of gestation. For those patients with a nuchal translucency between 2.5 millimetres and 3.5 millimetres, detailed fetal echocardiography is scheduled instead for 20 weeks. In a series of 1210 fetuses seen between 11 and 14 weeks since 2000, 980 had a nuchal translucency over 3.5 millimetres, the rest forming a normal control group. Diagnostic images were obtained abdominally in nine-tenths of cases. Of the 980, one-quarter (252) had abnormal cardiac findings, of whom 85% had karyotypic abnormalities. It is important to realise that detailed fetal cardiac evaluation is difficult at 12 weeks, and becomes progressively easier with every day between 12 and 14 weeks. The mean size of the aorta and pulmonary arteries at this gestation is about 1 millimetre, whereas by 14 weeks they are about 1.7 millimetres, and 3.5 millimetres by 20 weeks, so the heart more than triples in size between 12 and 20 weeks. Despite this, with experience and practice, it is usually possible to display clearly two atriums and two ventricles of equal size, two great arteries in spiralling relationship, the arch, and the arterial duct (Fig. 2). Use of colour flow mapping in these tiny chambers and vessels allows confirmation of flow throughout the heart in the expected antegrade fashion. Doppler sampling of the atrioventricular and arterial valves is usually possible. This being true, the failure to demonstrate normal findings is suspicious, and the subsequent recognition of anomalies such as the hypoplastic left heart syndrome, atrioventricular septal defect, pulmonary atresia with intact septum, and discordant ventriculo-arterial connections have all reliably been made in this setting (Figs 3–7). Sometimes during the nuchal scan, the sonographer recognises a cardiac malformation even when the nuchal translucency is normal (Fig. 8), so this screening programme in itself often leads to early referrals. Many fetuses with a diagnosis of congenital cardiac disease, which is associated with a chromosomal defect, proceed to termination of pregnancy. There is often no confirmation of the diagnosis by autopsy. In a fairly large series of patients, however, we have been able to obtain follow-up either by autopsy, in 32 cases, or by later fetal scan, in 218 cases. Of these 250, confirmation of the findings was complete in 243, with only minor revisions or additions to diagnosis made in the remainder.
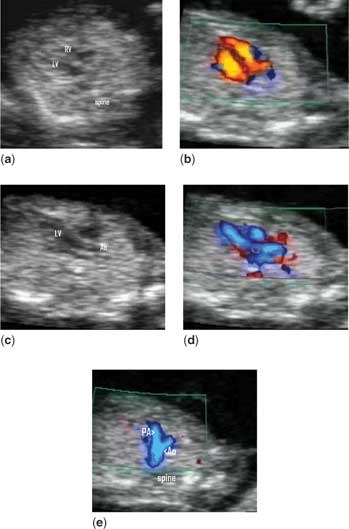
Figure 2. A normal heart (a) is seen at 12 weeks in the four-chamber view. Two equal atriums and ventricles can be seen, which fill on colour flow mapping (b) across patent atrioventricular valves. The origin of the aorta from the left ventricle can be seen (c), and is confirmed on colour flow mapping (d). In the plane immediately cranial to that shown in (d), the pulmonary trunk can be seen crossing over the aortic origin (e), confirming the normal spiral arrangement of the great arteries and that the vessels are of approximately equal size.

Figure 3. This fetus has an atrioventricular septal defect. The four-chamber view is seen in systole (a), when the defect at the lower end of the atrial septum is seen. In the diastolic frame (b), the common valve is seen to open across the crest of the ventricular septum.

Figure 4. In the four-chamber view (a), the smallness of the left side of the heart can be appreciated. Aortic atresia is confirmed by the demonstration of reverse flow in the aortic arch (b). The arterial duct lies below the arch, is much larger and shows forward flow.

Figure 5. In a long-axis view of the left ventricle (a), the aorta is seen to arise astride the crest of the ventricular septum. The pulmonary trunk crosses the aortic origin in the normal way, but is appreciably smaller than the aorta (b). This is tetralogy of Fallot.

Figure 6. The cavity of the left ventricle is readily seen (a), whereas the cavity of the right is small and the right ventricular walls appear thick. On colour flow mapping (b), the left ventricle fills whereas the right does not. This is a typical appearance of pulmonary atresia with intact ventricular septum.

Figure 7. The great arteries arise in parallel orientation, and do not show their normal spiral arrangement. This is discordant ventriculo-arterial connections.

Figure 8. Although the nuchal translucency measurement was normal in this fetus, the sonographer noticed the cardiac enlargement during the scan. This was an early example of Ebstein's malformation of the tricuspid valve.
An unexpected finding that has proved interesting has been the detection of functional cardiac abnormalities in the early scan, detected on pulsed Doppler. Tricuspid regurgitation has been found in over half of the fetuses with congenital cardiac disease. Isolated tricuspid regurgitation, on the other hand, is found in almost half of those fetuses who later prove to have a karyotypic anomaly, particularly trisomy 21, in contrast to only one-tenth of those with an increased nuchal translucency and a normal karyotype.17 Of this tithe, half proved to have other genetic syndromes on later follow-up. Unusual patterns of flow, such as bi-directional flow in the aorta, pulmonary trunk or duct, or reversed E/A ratio across the atrioventricular valves, tend to be strongly associated with trisomy 18.
Although increased nuchal translucency can persist in some cases, usually in genetic syndromes, such as Noonan's syndrome, it is more frequently transient, even in the chromosomally abnormal fetus. It usually has resolved, or is resolving, by 14 weeks. This is also true of isolated tricuspid regurgitation, even in trisomy 21. Makikaillo et al.18 found atrioventricular valvar regurgitation in up to half of normal fetuses at 10 weeks gestation, in contrast to our finding of 2–4% of normals with normal nuchal translucency at 12 weeks. A possible explanation of this is that the tricuspid valve is still in the process of delamination at 10 weeks, a process which is completed between 9 and 11 weeks.19 It may be that fetuses with trisomies 21 and 18 have delay in this normal maturation process, resulting in tricuspid regurgitation being still present at 12 weeks, or it may be that the crux of the heart is simply different from normal at this stage in the chromosomally abnormal fetus.
The mechanisms producing nuchal translucency
There have been several theories put forward as to the underlying mechanism to increased nuchal translucency, most commonly, especially in the obstetric literature, that of “cardiac failure” or “cardiac dysfunction”. This is based on the idea that oedema in the fetus must, of necessity, be caused by cardiac failure, despite the fact that there are many other established causes of hydrops in the later fetus. Alternatively, the idea that a structural cardiac malformation, such as a ventricular septal defect, could account for oedema is proposed. Clearly, it is untenable for a ventricular septal defect to cause cardiac failure in fetal life. Support for the cardiac failure theory came from a paper by Hyett et al.,20 where they found increased levels of atrial and brain naturietic peptides in 15 fetuses with increased nuchal translucency compared to 30 controls. It is known that the secretion of these enzymes is developmentally regulated, yet nothing is known of the pattern of secretion in fetuses with trisomies, which all 15 had. Additionally, it is not unlikely that retention of fluid in itself is a stimulus that could increase levels of the peptides, when the myocardium was responding in an appropriate fashion to this stimulus. Nothing is known of the mechanism, or degree of stability, of regulation of fluid balance prior to about 11 weeks gestation, when renal function starts to come into play, this process taking over regulation completely by about 14 weeks. This coincides with the usual timing of resolution of nuchal translucency, even in the abnormal fetus. Against the idea of cardiac failure is the fact that the heart is not enlarged, and has normal systolic function. Further evidence of normal cardiac function was found in a series of 638 fetuses, where the Tei index, and the E/A ratio of the atrioventricular valves, was measured between 11 and 14 weeks.21 Of these, 159 were normal controls, 199 proved to be normal on follow-up but had a nuchal translucency over 4 millimetres, 24 had isolated congenital cardiac disease, and the remaining 256 had chromosomal anomalies. Of these 256, there were 142 cases of trisomy 21, 58 of trisomy 18, 19 of trisomy 13, and 37 of Turner's syndrome. In this study, no important differences in the indexes of cardiac function were found between any of the study groups and the controls.
A further mechanistic theory was put forward by Bekker et al.,22 who found abnormal lymphatic vessels in the neck region in fetuses with increased nuchal translucency. They suggested that there was a primary disorder of lymphangiogenesis, or endothelial function, which could cause both collections of nuchal fluid and also cardiac malformations. Abnormalities in the lymphatic vessels, however, may be induced by the excess nuchal fluid itself.
It appears more likely that some form of disturbance of haemodynamics or flow may be responsible for inducing the cardiac malformation and causing distension of the tissues. Experiments in the chick embryo show that alterations in cardiac inflow, either by central or peripheral manipulation of streams of flow, induce structural malformations.23, 24 Normal patterns of flow affect expression of the normal sequence of growth factors, without which the chambers do not develop in the usual way.25 It is well known that ablation of the neural crest is associated with the development of cardiac abnormalities, especially of the ventricular outflow tracts. This was attributed to the failure of neural crest cells to migrate to the outlet component of the heart in the normal fashion during development. More recent work suggests that disturbance of flow after ablation of the neural crest occurs prior to the development of cardiac malformations, and this may be the mechanism, rather than lack of migration of cells.
Flow across the venous duct
The Doppler flow profile in the venous duct has received much attention in recent years as an indicator of cardiac function. The venous duct connects the umbilical vein to the inferior caval vein, and acts as a point of acceleration of flow (Fig. 9). An abnormal flow profile can be associated with increased nuchal translucency, cardiac malformations, and chromosomal anomalies.26, 27 In the normal fetus, there is a characteristic pattern of forward flow throughout systole and diastole in this vessel (Fig. 10a). The jet from the duct travels via the inferior caval vein as a column of blood directly into the left atrium through the oval foramen. Absent or reversed flow in diastole has been suggested as reflecting the central venous pressure later in pregnancy (Figs 10b,c). The Doppler signal in the venous duct, however, can be unobtainable in some early fetuses, and overlap from adjacent vessels can cause difficulty in interpretation of the flow profile. Martinez et al.28 examined the pulsatility index in the jugular vein in 179 first trimester fetuses, 22 of whom had increased nuchal translucency, and found that there was no correlation between the index in the jugular vein and the venous duct. This suggests that there must be other explanations than an increase in central venous pressure for an abnormal flow profile across the venous duct in this setting. Other causes of an abnormal flow profile which have been described include dilation of the venous duct in hypoxia, increased adrenergic drive, a relative restriction of the oval foramen, which is known to occur in fetal right heart disease, and an increase in the end-diastolic pressure in the left ventricle.

Figure 9. The venous duct (arrowhead) is seen in a long-axis view of the fetal abdomen. It connects the umbilical vein to the inferior caval vein.

Figure 10. The Doppler sample volume is correctly positioned in the venous duct (a), and shows a normal flow profile, with forward flow throughout the cardiac cycle. In panel (b), there is absence of forward flow in diastole in the profile, while panel (c) shows reversal of flow in diastole.
Association with certain cardiac malformations
Does the type of congenital heart disease seen in association with increased nuchal translucency provide any clues to the mechanism? In our centre, of 207 fetuses with abnormal chromosomes, there was a scatter of diagnoses typical of chromosomal defects, with atrioventricular septal defect and left heart disease comprising the majority, with ventricular septal defect, tetralogy and double outlet right ventricle making up the rest. The relative under-representation of ventricular septal defect in the fetal series in comparison with postnatal series can be accounted for by the difficulty in detection of even moderate ventricular septal defects in fetal life. The diagnostic categories in 123 fetuses with normal chromosomes and an increased nuchal measurement are seen in Figure 11. There is a similar scatter of disease to that expected in neonates, with the possible exception of the rate of coarctation of the aorta and atrioventricular septal defect. Coarctation was more frequent than might be expected, but this can be explained by the fact that some of them may have become examples of the hypoplastic left heart by the end of pregnancy, and a further group of anticipated cases of coarctation may have proved normal after birth. Coarctation is one of the few diagnoses where a false positive diagnosis can occur in the fetus.29 The rate of atrioventricular septal defect, at about 12%, is close to the overall rate in infant series, but in our series the chromosomal defects have been taken out of this group. So this rate does appear to be higher, perhaps more than twice, the expected rate in infants. Whether this is of any significance needs further investigation.

Figure 11. The scatter of diagnostic categories in 123 cases of fetal congenital heart disease. All had increased nuchal translucency and normal chromosomes.
Conclusions
An exciting prospect to all this is that it seems likely that the ability to make the diagnosis of congenital cardiac disease so much closer to the timing of the insult which produces the cardiac malformation, might lead to the identification of the insult itself. My current understanding of the mechanism and chronology of increased nuchal translucency and congenital heart disease is seen in Figure 12. To summarise the current situation:
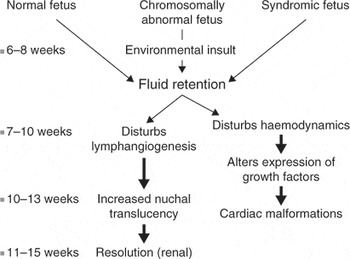
Figure 12. The cartoon shows the sequence of events which might produce increased nuchal translucency and cardiac malformations.
- Fetuses with an increased nuchal translucency are an important group at high-risk to be referred for detailed fetal echocardiography, with a greater risk of congenital cardiac disease than the traditional groups considered at increased risk.
- With practice and experience, major forms of congenital cardiac disease can reliably be diagnosed at 12 weeks gestation in the majority of fetuses examined at this time.
- Those fetuses with a nuchal translucency measurement over 3.5 millimetres should have a cardiac scan at least by 14 weeks, as they represent a group at particularly high risk who should not be made to wait until 20 weeks for reassurance.
- Any form of congenital cardiac disease may be associated with increased nuchal translucency.
- The underlying mechanism that produces increased nuchal translucency remains elusive.