Measurement of cardiac output in paediatric cardiac surgery has become increasingly important. During the perioperative period, young children are at a higher risk of haemodynamic instability. Reference Nusmeier, van der Hoeven and Lemson1 Moreover, early recognition and correction of instability can reduce poor outcomes relating to organ morbidity. Reference Nusmeier, van der Hoeven and Lemson1 Blood pressure monitoring techniques allow for the detection of arterial hypotension, but ultimately do not inform the clinician of the cause for the blood pressure drop. 2 Therefore, the clinician is unaware of whether the drop in pressure results from a change in systemic vascular resistance or changes in cardiac output. Knowing the pathophysiology behind the systemic drop in blood pressure would determine the treatment options available to help support the patient. This would include knowing cardiac output measurements.
New technologies have been developed to monitor cardiac output over the previous few decades. Many of these methods are already being utilised in modern medicine for paediatric cardiac surgery. Haemodynamic stability is assessed invasively and non-invasively, with non-invasive techniques often used at the initial stage. Simple, non-invasive measures to monitor cardiac output or systemic vascular resistance are urine output, arterial pressure, toe-core temperature difference, and capillary refill time. Reference Purday3 More invasive techniques such as pulmonary artery catheter measurement and arterial and central venous pressure waveforms, have also been used in perioperative paediatric patients. Reference Trieu, Williams and Cannesson4 Newer non-invasive cardiac output monitoring modalities including electrical cardiometry, impedance cardiography, and bioreactance have been described in the literature and have been introduced to paediatric populations, however, the real-life implementation of these has been limited. It can be difficult for clinicians to decide which technique should be used for cardiac output monitoring, as each has advantages and limitations. The following review focuses on the currently available invasive and non-invasive monitoring devices for cardiac output measurement in children. An explanation of each modality will be described briefly to provide an overview. The overall aim of this review is to present the current options for cardiac output monitoring in paediatric patients and to outline which modalities may prove appropriate for use in clinical practice.
Methods
A comprehensive evidence search was conducted using online databases including Medline Ovid, National Institute for Health and Care Excellence Evidence, and Cochrane Library, which captured the available information for this review. Search terms used to conduct the search are shown in Table 1 and inclusion criteria are shown in Table 2. The population, intervention, comparator and outcome decided on to devise the literature search can be seen in Table 3. Any relevant evidence found within the search was title screened. The evidence remaining was then abstract screened. Finally, a full-text screen was conducted. The literature’s title, abstract, and full text were screened by two independent reviewers. All guidelines included were appraised using the AGREE II tool and systematic reviews and primary research were appraised using the appropriate critical appraisal skills programme tools. 5,6 The patient characteristics for each reviewed study can be viewed in Table 4.
Table 1. Databases and search terms used to generate guideline, systematic review, and primary research literature

Table 2. Inclusion criteria used in the literature search for guidelines, systematic reviews, and primary research studies

CI = cardiac index; CO = cardiac output; HR = heart rate; RCT = randomised control trial; SV = stroke volume; TPRI = total peripheral resistance.
Table 3. PICO framework used for literature search for review title

CI = cardiac index; CO = cardiac output; HR = heart rate; SV = stroke volume; TPRI =, total peripheral resistance.
Table 4. Patient characteristics of reviewed studies

ASA = American Society of Anaesthesiologists; CO = cardiac output; GA = general anaesthesia; NA = not available; NHA = normal heart anatomy; NICOM = non-invasive cardiac output monitoring; OR = operating room; PAC = pulmonary artery catheter; SD = standard deviation; TFC = thoracic fluid content; VSD = ventral septal defect.
Technologies for cardiac output measurement
Cardiac output is an important measure for clinicians to monitor, especially throughout high-risk surgeries in paediatric patients. It indicates the current haemodynamic status and highlights when clinicians should provide intervention. The Royal College of Anaesthetists has published a guideline regarding anaesthetic provisions for cardiac and thoracic procedures. 7 The guideline states that cardiac output monitoring should be readily accessible for high-risk cardiac surgery cases and should even be considered for thoracic surgery. This guideline covers both adult and paediatric patients and, whilst it stresses the importance of monitoring, there is no mention of the method that should be chosen. This guideline scored highly using the AGREE II tool, showing the evidence was found using a comprehensive strategy. Multiple modalities of monitoring cardiac output have been developed using invasive and non-invasive methods. Each of the methods of cardiac output monitoring featured within the reviewed papers will be expanded upon individually. Table 5 details the advantages and disadvantages of each cardiac monitoring modality.
Table 5. Advantages and disadvantages of each CO monitoring technique
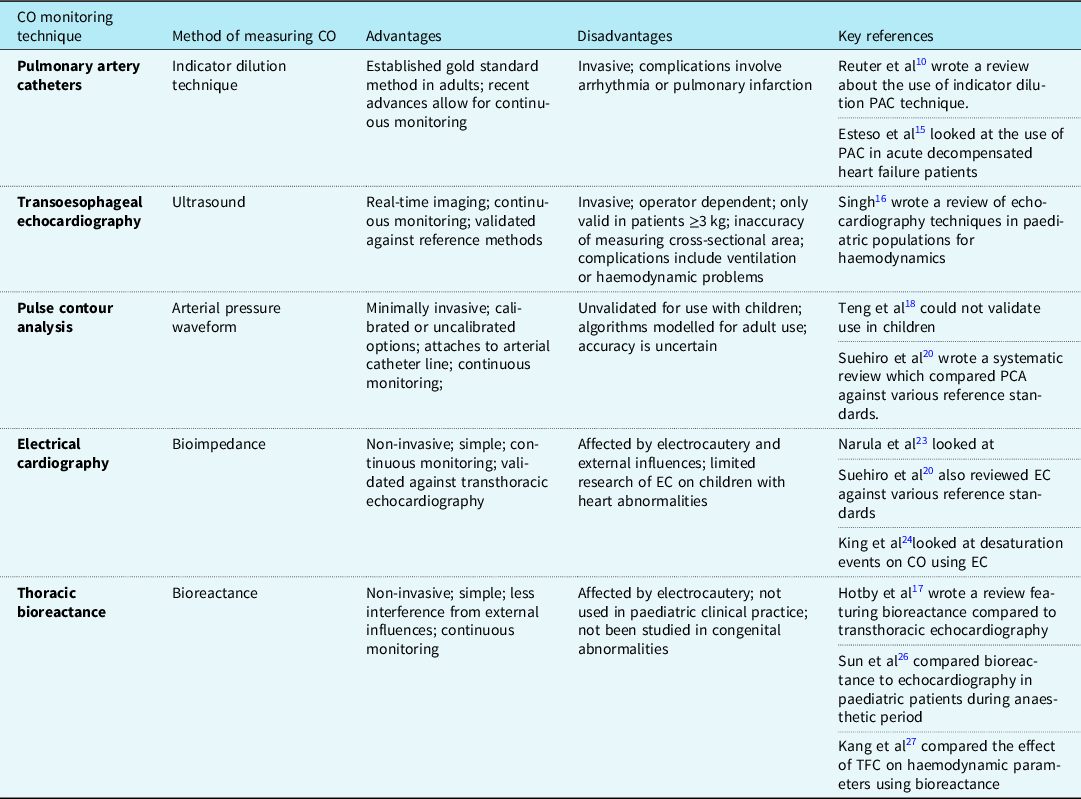
CO = cardiac output; EC = electrical cardiography; kg = kilograms; PCA = pulse contour analysis.
Invasive methods
Pulmonary artery catheter
Pulmonary artery catheter for the use of cardiac output monitoring in patients with CHD was first described in 1950 by Dexter. Reference Dexter8 In adults, this method has been validated and is considered to be the gold standard to monitor cardiac output using the thermodilution technique. Reference Mehta and Arora9 Pulmonary artery catheters use pulmonary blood flow (Qp) as a measure of cardiac output. Suggested gold standard methods for cardiac output monitoring in children are the indicator dilution technique or the Fick principle. Reference Reuter, Huang, Edrich, Shernan and Eltzschig10 There are, however, many variations to this technique including thermodilution and dye dilution. All use the theory of injecting a soluble, inert substance and then measuring the dilution of the substance downstream. The speed of the dilution relates to blood flow, and cardiac output is calculated by assessing the time taken for the change in either temperature or concentration of the measuring substance between two static points. Intermittent measurements are complex and involved, and so can be difficult to use when tracking rapid changes in cardiac output. However, recent technological advances have allowed for continuous cardiac output monitoring due to the inclusion of a rapid response probe coupled with a computer programme, allowing cardiac output to be calculated quickly.
The insertion of a pulmonary artery catheter is invasive and there are subsequent complications associated with its use. Difficulties can arise during the insertion due to potential complications such as difficulty placing the catheter or arrhythmias. Additionally, inaccuracies may occur due to the size variabilities of structures leading to mal-placement of catheter ports or presence of intracardiac shunts. Therefore, the risks and benefits of using a pulmonary artery catheter need to be carefully considered. Reference de Boode11–Reference Yartsev13
Guidelines written by the National Health Service Greater Glasgow and Clyde explain the insertion and care of a pulmonary artery catheter in paediatric patients and warn about the potential complications of this procedure, including arrhythmia, pulmonary infarction, and pulmonary artery rupture. 14 The AGREE II tool was used to evaluate this guideline. Using this tool, the guideline was shown to have a clear objective and targeted the correct audience. The literature used to develop this guideline was appropriate and clearly referenced. However, there was limited information regarding the process in selecting the references used to devise the guideline.
Esteso et al have conducted a quality improvement study into the use of pulmonary artery catheters in children as part of the management of acute decompensated heart failure. Reference Esteso, Blume and VanderPluym15 Their study had a small sample size of 11 patients. Two adverse events occurred, both of which were arrhythmias, and may have been related to the presence of the pulmonary artery catheter. This study demonstrates that whilst the use of a pulmonary artery catheter within children is feasible, more evidence is required to determine the accuracy and rates of potential risks associated with this method.
Transoesophageal echocardiography
Transoesophageal echocardiography is a method which utilises ultrasound and the doppler effect to measure blood flow velocity by the placement of an ultrasound probe into the oesophagus. The use of a spectral doppler displays the flow velocity over time as a graph which allows the velocity-time integral to be measured from the area under the curve. The velocity-time integral multiplied by the cross-sectional area of the aorta, as measured by planimetry, gives the stroke volume and the cardiac output is subsequently calculated by multiplying stroke volume and heart rate. Reference Mehta and Arora9
Transoesophageal echocardiography requires the insertion of the probe by a trained practitioner. This method’s accuracy has been validated against other reference methods such as Fick’s principle, pulmonary artery thermodilution, and dye dilution. Reference Holtby, Skowno and Kor16 In addition to allowing for cardiac output monitoring, the use of ultrasound allows for real-time imaging of the heart and surrounding vessels, which may be of use perioperatively, especially during cardiac heart defect surgery. Reference Teng, Kaufman and Pan17 However, this technique is limited to use in children whose weight is greater than 3 kg, as the risks of inserting the oesophageal probe increases in proportion to the patient’s size. Furthermore, inaccuracy in measurement of the cross-sectional area of the aorta, especially in neonates, can result in erroneous cardiac output measurements. Reference Singh18 Complications of using transoesophageal echocardiography include ventilation problems or cardiac compression causing true haemodynamic changes or vessel compression causing factitious haemodynamic changes. Reference Singh18 Additionally, the use of ultrasound means that there is a narrow directional range of view and so small movements can disturb the optimum view. This may limit its use if readjustment of the probe disrupts the cardiac surgery. Reference Holtby, Skowno and Kor16
Pulse contour analysis
Pulse contour analysis offers a minimally invasive method of measuring cardiac output through the attachment of a device to a peripheral arterial line. The arterial pressure waveforms are used to calculate various cardiac parameters, including cardiac output. These can either be calibrated or uncalibrated. Calibrated pulse contour analysis methods use an internal reference method in combination with pulse contour analysis to calibrate the algorithms to the individual patient. Reference Grensemann19 Calibration may be required to avoid incorrect cardiac output measurements in septic patients, where there are changes in the vascular impedance and compliance of vessels. Uncalibrated pulse contour analysis uses algorithms based upon the updated Otto Frank’s Windkessel model. Reference Grensemann19 Stroke volume is estimated from calculations using the arterial catheter pressure measurements and the inclusion of patient demographics increases accuracy within this approximation.
This system is advantageous as many paediatric patients will already have an arterial catheter placed, hence connection of the pulse contour analysis system is simple. Reference Holtby, Skowno and Kor16 Similar to other cardiac output monitoring methods, pulse contour analysis was designed for adult use before being introduced to paediatric practice and the algorithms used by these devices were modelled on the adult population. It is inappropriate to assume the same algorithms will apply to children as younger populations will accommodate different levels of vessel compliance which may affect the accuracy of cardiac output measurement.
Furthermore, the use of the pulse contour analysis in paediatric populations has not been approved. Teng et al found that they could not validate its use in children as there was great variability in the readings that were produced during its use. Reference Teng, Kaufman and Pan17 The authors suggest that this is due to the FloTrac algorithm only being validated in elderly patients with atherosclerotic vessels, compared to their population of children with more compliant vessels and higher heart rates, thus resulting in smaller stroke volumes.
Within the systematic review conducted by Suehiro et al, four studies were found to compare pulse contour analysis use in paediatric groups against various reference standards. Reference Suehiro, Joosten and Murphy20 Within these four studies, a range of pulse contour analysis devices were used (LiDCO, MostCare, FloTrac, and esCCO), which were investigated in a range of clinical scenarios including surgery. The mean bias for pulse contour analysis was 0.32, however, the 95% limit of agreement was wide. The percentage error was 33%, which is above the accepted limit of 30%. Therefore, even though pulse contour analysis may be attractive due to its ease of use, the accuracy of this method is uncertain. The accuracy of this modality worsens in uncalibrated methods.
Non-invasive methods
The methods described previously are all invasive to some degree and have associated risks, therefore non-invasive modalities appear desirable and have been developed to combat the disadvantages of the aforementioned techniques. Impedance cardiography was first developed in the 1960s for NASA to monitor astronaut’s cardiac output. Reference Miller and Horvath21 This method, also called thoracic electrical bioimpedance, involves electrode placement on the neck and lower thorax to measure the resistance between them. Reference Dexter8 This impedance change occurs due to the varying intrathoracic blood volume throughout the cardiac cycle. However, the measurements are dependent on correct electrode placement and can be affected by electrocautery. Since then, thoracic electrical bioimpedance has been modified into two separate methods: electrical cardiometry and thoracic bioreactance.
Electrical cardiometry
Electrical cardiometry was developed in 2001 and involves electrodes placed in the same position as thoracic electrical bioimpedance. This method, however, measures the change in conductivity due to the orientation of the red blood cells. Red cells are randomly orientated prior to the aortic valve opening and then align during early systole. This alignment of cells produces a pulsatile change in electrical conductivity which leads to a decrease in electrical velocimetry during early systole and an increase later. Mathematical algorithms formulated by Bernstein and Lemmens Reference Bernstein and Lemmens22 are used to calculate the cardiac output. There is limited research available on the evaluation of electrical cardiometry on paediatric patients with congenital heart abnormalities, however, Narula et al have described its use in children with structural heart defects. Reference Narula, Chauhan and Ramakrishnan23 The heart defects in the study were atrial septal defect, aortic valve balloon dilation, major aortopulmonary collateral arteries, patent ductus arteriosus, and pulmonary valve balloon dilation. Due to the small population size in Narula et al and limited research on a range of congenital abnormalities, more research is needed in patients with congenital heart abnormalities including patients who are post-corrective surgery.
Electrical cardiometry does however offer a safe, simple, and non-invasive method of cardiac output monitoring and this has been validated against transthoracic echocardiography after cardiac surgery in smaller infants with low bias. Reference Holtby, Skowno and Kor16 Within the systematic review, electrical cardiometry was the most featured device, appearing in eight of the studies evaluated. Reference Suehiro, Joosten and Murphy20 It was also the most accurate technology evaluated in the systematic review, with the smallest bias and the lowest percentage error. However, the device is only as accurate as the reference method, and there was high heterogeneity found within the systematic review due to the wide range of techniques and reference standards used. Therefore, this evidence should be considered cautiously.
King et al conducted a study looking at the impact of desaturation events on various cardiovascular measurements, including cardiac monitoring using the ICON electrical cardiometry monitor in a group of paediatric patients under general anaesthesia. Reference King, Anderson and Sui24 The study did not find a definitive correlation between desaturation and subsequent effect on cardiovascular parameters, but does suggest that continuous monitoring may allow for better identification of decreasing cardiac function. However, this study was conducted in the anaesthetic period and not during the perioperative period and so further investigation would be needed to test the feasibility of using electrical cardiometry during the perioperative period.
A major barrier to the clinical use of electrical cardiometry perioperatively is the intermittent interference caused by electrocautery. Further studies should aim to investigate the clinical application of electrical cardiometry perioperatively and whether this interference would prevent continuous cardiac output monitoring.
Thoracic bioreactance
Thoracic bioreactance is another modification of thoracic electrical bioimpedance and an example of such a device is the non-invasive cardiac output monitoring device. Reference Mehta and Arora9 Rather than detecting changes in the resistance due to fluid volume or red blood cell orientation, bioreactance monitors how the phase of the current across the chest increases in proportion to the stroke volume. Cardiac parameters can be calculated using the phase shifts in voltage. This allows for less interference from noise and other external influences. Bioreactance is also safe, simple, and completely non-invasive. However, it has not currently been adopted into paediatric clinical practice. Research on the use of bioreactance shows viability in term and preterm populations; however, this is only in regard to normal heart anatomy and has not been studied in those with congenital abnormalities. Reference McGovern and Miletin25
The review conducted by Holtby et al included a study that discouraged the use of bioreactance for paediatric hypovolemic shock, however, this was based upon an animal model. Reference Holtby, Skowno and Kor16 Additionally, Holtby et al described a neonatal study which assessed bioreactance compared to transthoracic echocardiography. The population studied were pre-term infants after patent ductus arteriosus ligation. There were similar measurements found between the two modalities, however, there was a significant bias of 31 ± 8%. Due to the presence of bias and the lack of evidence to support this modality, Holtby et al concluded that further evaluation of bioreactance in paediatrics is required before recommending its clinical use. Reference Holtby, Skowno and Kor16
Sun et al investigated the accuracy of bioreactance compared to echocardiography in paediatric patients during the anaesthetic period. Reference Sun, Wu and Wu26 The study investigated this in two groups: patients with ventral septal defects and those with normal heart anatomy. The bioreactance device used was a non-invasive cardiac output monitoring device. The results of this study found the non-invasive cardiac output monitoring device had good agreement with the echocardiography reference in the normal heart anatomy group. However, in the group with ventral septal defect, the values of cardiac output were lower than the echocardiography value. Sun et al described some possible confounding factors causing this outcome. When calculating cardiac output using an echocardiogram, Simpson’s rule is used to calculate the right and left ventricular end-diastolic volume and end-systolic volume and so blood ejected from the aorta and left-to-right shunt is accounted for. Whereas when using non-invasive cardiac output monitoring, cardiac output is calculated by measuring blood flow ejected from only the left ventricle. Therefore, the measurement determined by an echocardiogram will be greater than a measurement using non-invasive cardiac output monitoring in children with ventral septal defect. In addition, in the normal heart anatomy group, fentanyl was used to induce the patient. This drug has a limited haemodynamic effect up to 5–10 minutes. Whereas in the ventral septal defect groups, sufentanil was used, which has a more prolonged and potent effect. Ultimately, this may have led to a variation in heart rates and mean blood pressures between the two groups.
This study is limited in its application to our topic, as the investigation was only conducted during induction and intubation, and so provides no information on the use of non-invasive cardiac output monitoring perioperatively. The authors explained that it would be impractical to use echocardiography as a reference method throughout surgery and an alternative method would need to be deployed in order to minimise interference during surgery. Additionally, this study only decided to recruit patients with ventral septal defect and none of the other heart defects found in children. Therefore, this study cannot be generalised to other paediatric cardiac surgery.
Kang et al have investigated thoracic fluid content and haemodynamic parameters in a group of paediatric patients with CHD undergoing corrective surgery. Reference Kang, Lee and Shin27 Thoracic fluid content is an indicator of total fluid volume, both intracellularly and extracellularly, which is measured non-invasively using impedance cardiography. A mean transthoracic electrical impedance (Zo) reading is produced and varies with the volume of fluid in the thorax. As thoracic fluid increases the Zo reading decreases. This study also used a non-invasive cardiac output monitoring bioreactance device and measured cardiac output, cardiac index, and stroke volume continuously. The values of cardiac output measured by the non-invasive cardiac output monitoring bioreactance device were lower than normal range values, which reinforces previous evidence that the bioreactance method consistently under reports cardiac output. In this study, bioreactance via the non-invasive cardiac output monitoring device was used to measure both cardiac output and thoracic fluid content and it was concluded that thoracic fluid content is a good benchmark for haemodynamic status. This suggests that using bioreactance to measure cardiac output alone may be misleading and underestimates the true reading. However, combining cardiac output and thoracic fluid content measurements may provide the clinician with a more reliable estimate of the patient’s status and whether intervention is required. Reference Kang, Lee and Shin27
Limitations
The recurring limitation of this literature review is the lack of conclusive data regarding our chosen field: cardiac output monitoring during paediatric cardiac surgery. As a result, our search produced few studies, some of which were conducted at a lower quality of research. Ultimately, this hindered our ability to conduct a true representation of the cardiac output modalities available. Apart from the Sun et al study looking at the bioreactance technique, Reference Sun, Wu and Wu26 none of the other studies evaluated compared the technique in children with normal heart anatomy against those with congenital heart defects. Therefore, no conclusion can be made about the accuracy of these techniques for paediatric cardiac surgery as we are unsure if this inaccuracy is related to the heart defect or the paediatric population. In addition, the outcomes of these studies were incredibly varied meaning direct comparisons were not able to be made, which limited our analysis.
The study by Esteso et al was a retrospective cohort study as part of an ongoing quality improvement study and, therefore, was only reviewed in abstract form without the full data. Reference Esteso, Blume and VanderPluym15 Additionally, Holtby et al wrote a review that covered three separate paediatric issues, one of which was cardiac output monitoring. Reference Holtby, Skowno and Kor16 This resulted in the inability to critically analyse using the critical appraisal skills programme tool. In addition, this study included papers from a wide range of clinical scenarios, thus the data cannot be generalised fully to our chosen area of cardiac surgery. Finally, none of the included studies covered the topic of cost-effectiveness, which is a key consideration for evaluating the clinical adoption of any device.
Future research
Cardiac output monitoring has been investigated in depth in adult populations, however, the evidence for measurement in vulnerable paediatric populations is lacking. Adult research has benefited from a history of gold standard techniques to allow for comparison with more novel modalities. Conversely, there is no agreement in the literature for a gold standard method for cardiac output monitoring in children. The evidence conducted so far has no standardisation in the population investigated, nor do the reference methods chosen, leading to much uncertainty surrounding cardiac output monitoring in children. Therefore, clinicians cannot make confident evidence-based decisions regarding their choice of cardiac output monitoring method.
It has been previously mentioned that in children with anatomic shunts it has been difficult to assess the utility of cardiac output monitoring devices. Currently, the Fick equation is used to assess cardiac output in patients with a shunt. The Fick equation states that cardiac output can be calculated by dividing the difference between the inspired and expired oxygen (VO2) by the difference between the arterial oxygen concentration and the venous oxygen concentration. Reference Hoeper, Maier and Tongers28 VO2 is equal to oxygen consumption. This equation is not affected by valvular regurgitation, shunts, or arrhythmias, and therefore could become a feasible standard to compare the use of different devices for cardiac output monitoring. However, one weakness of using the Fick equation clinically is that oxygen concentration is rarely measured.
It would be beneficial for a standardised method for data collection and reference standards to be adopted for comparison between studies. To supplement this, cost-effectiveness studies for each modality should be investigated to aid future guideline construction.
Conclusion
Cardiac output monitoring for children remains in a grey area with no current evidence able to safely encourage the use of any technique in clinical practice. Future research should observe clinical outcomes from cardiac output monitoring perioperatively and efforts need to be concentrated on a standardised data collection method for this field to advance.
Acknowledgements
None.
Financial support
This research received no specific grant from any funding agency, commercial, or not-for-profit sectors.
Conflicts of interest
None.