Stent implantation to treat stenoses of large vessels has become a well-established procedure in children and adolescents. It may also be an option in neonates and infants to overcome severe stenoses. Because of the small vessel diameters and the tightness of the stenosis, coronary stents are often used. When implanting a coronary stent, redilatations and growth adjustments are limited to the maximum diameter of these stents (<=5 mm), which requires its later surgical removal.
Bioabsorbable stents for vascular stenosis in newborns and infants have been considered to overcome these restrictions. First experiences with a magnesium stents in infants date back to 2004.Reference McMahon, Oslizlok and Walsh1–Reference Zartner, Buettner, Singer and Sigler4 The early results were encouraging, but later outcome was inconsistent.Reference McMahon, Oslizlok and Walsh1,Reference Schranz, Zartner, Michel-Behnke and Akinturk2 A new generation of bioabsorbable stents has been developed, now referred to as scaffolds.
We present our experience with the magnesium-based scaffold (Magmaris™, Biotronik, Berlin, Germany) in form of a multiple case analysis, presenting our data on indications, locations, short- and mid-term results, and the possible benefit for infants and young children with severe vascular stenoses.
Methods
Stent material
The magnesium scaffold is available in 3.0 and 3.5 mm diameter and 15, 20, and 25 mm length (Figure 1) and passes through a 4-F delivery sheath. It shows a degradation rate of its backbone material of 15% after 4 weeks, 40% after 3 months, and 95% after 1 year.Reference Haude, Ince and Abizaid5 From pre-clinical trials, the circumferential stability was estimated to last 4–8 weeks. This was considered to meet the requirements of further vessel growth in a fast-growing subject and would not hinder later surgical or interventional procedures should they become necessary.
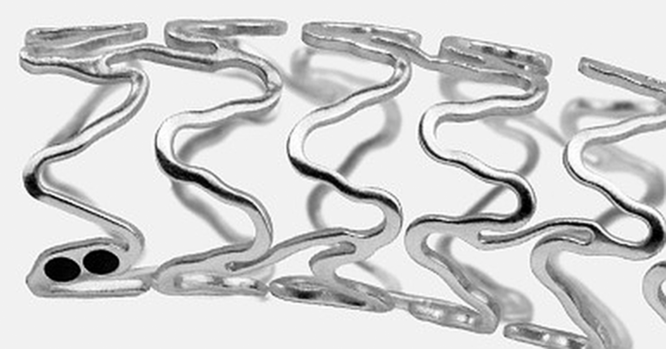
Figure 1. The magnesium scaffold with the two black platinum markers at both ends to visualise the stent position under fluoroscopy. It comes pre-mounted on a semi-compliant coronary balloon with two markers to allow proper positioning.
All bioabsorbable scaffolds are covered with a cytostatic medication to avoid restenosis caused by intimal hyperplasia. The struts of the magnesium scaffold are covered with a polymer layer containing Sirolimus® (rapamycin). Rapamycin is an immunosuppressive drug and mechanistic target of rapamycin inhibitor with a low thrombogenic potential.Reference Haude, Ince and Abizaid6,Reference Haude, Ince and Kische7 Rapamycin plays an increasing role in paediatric oncology and transplant medicine, and, if length of treatment is limited, reported adverse effects are rare and without permanent impairment.Reference Canpolat, Per and Gumus8,Reference Hammill, Wentzel and Gupta9
Population
Since June 2016, 15 magnesium scaffolds were implanted in 9 young children during 13 interventions. The age at the time of the magnesium scaffold implantation ranged from 15 days to 7.6 years (mean 2.4 years, standard deviation 2.1 years). The patients’ lengths ranged from 50 to 117 cm (mean 83.5 cm), and their weights from 3.2 to 27.8 kg (mean 6.8 kg). All magnesium scaffolds had a diameter of 3.5 mm, three were of 20 mm length, and the others were of 15 mm length.
Eight magnesium scaffolds were implanted in recurrent stenoses of the left upper pulmonary vein in three patients during seven interventions (Figure 2, Pat7, Table 1). One patient had a partial anomalous pulmonary venous drainage, the other two had a congenital pulmonary venous stenosis, all with restenosis after sutureless repair. Three other patients had stenotic aortopulmonary collateral arteries in pulmonary atresia after surgical unifocalisation. Two of them received magnesium scaffolds in peripheral lobar pulmonary artery stenosis (Figure 3, Pat4, Table 1). The right ventricular function of one patient in this group was severely depressed after unifocalisation with subtotal occlusion of both anastomosed pulmonary arteries. Right ventricular pressure was suprasystemic, and magnesium scaffolds were implanted in both anastomoses in an emergency procedure (Figure 4, Pat5, Table 1). One patient with a tetralogy of Fallot with severely hypoplastic pulmonary arteries after conduit implantation and conventional stenting of the bifurcation showed severe intimal hypertrophy within the stent in the left pulmonary artery. In an effort to maintain perfusion of the left lung, we performed a “scaffold-in-stent” implantation (Figure 5, Pat8, Table 1) in an attempt to reduce further intimal growth. The smallest patient in this group with a bodyweight of 3 kg with a double outlet right ventricle and Swiss cheese ventricular septal defect was palliated in a first step with a Norwood I procedure with a modified Blalock–Taussig shunt. The modified Blalock–Taussig shunt was implanted from the brachiocephalic trunk to the right pulmonary artery, but distorted the proximal part of the brachiocephalic trunk and reduced pulmonary perfusion. Post-procedure, the patient suffered from low oxygen saturations. The brachiocephalic trunk between the aorta and modified Blalock–Taussig shunt was stented with a magnesium scaffold (Figure 6, Pat3, Table 1). The final patient with a tetralogy of Fallot suffered from recurrent thrombosis of the innominate vein, which was recanalised twice before a magnesium scaffold was implanted into the innominate vein to maintain patency (Pat6, Table 1).

Figure 2. Male patient at the age of 17 months with repeated restenosis of the left-sided pulmonary veins after surgical sutureless correction. The subtotal occlusion of the left upper pulmonary vein (arrow in a) was stented with a 3.5 -mm magnesium scaffold (arrows in b) and showed patent at 60 days follow-up (c). It was redilated with Paclitaxel-covered balloons at the age of 19 and 23 months. Because of ongoing restenosis, three magnesium scaffolds were implanted at the age of 28, 38, and 42 months. Four weeks later, a 4-mm cutting balloon and a 5-mm Paclitaxel-covered balloon were used to further increase the vessel diameter. To have a secure entrance to the left atrium, a septum stent was implanted at one of the first procedures (white arrow). Small picture: The magnesium scaffold with the two black platinum markers at both ends to visualise the stent position under fluoroscopy. It comes pre-mounted on a semi-compliant coronary balloon with two markers to allow proper positioning.
Table 1. List of interventions and implanted material related to the magnesium scaffold implantation

angio=angiography; AVSD=atrioventricular septal defect; CoA=aortic coarctation; BMS=bare metal stent; BTC=brachiocephalic trunk; DEB=drug-eluting balloon (Paclitaxel-covered); DORV=double outlet right ventricle; dTGA=transposition of the great arteries; echo=echocardiography; hypopl.=hypoplastic; ll.=left lower; LPA=left pulmonary artery; lu.=left upper; MAPCAs=multiple aorto-pulmonary collateral arteries; mBTS=modified Blallock–Taussig shunt; MgS=magnesium scaffold; PA=pulmonary artery; PAPVD=partial anomalous pulmonary venous drainage, Patr=pulmonary atresia; PHT=pulmonary hypertension; PV=pulmonary vein; PVS=pulmonary venous stenosis; RPA=right pulmonary artery; RSt=Restenosis; RV=right ventricle; RVOT=right ventricular outflow tract; TOF=tetralogy of Fallot; V=vena; VSD=ventricular septal defect; ↙=followed by intervention next line.

Figure 3. Three-year-old patient with pulmonary atresia of Fallot type, after unifocalisation with (a) severe stenosis of the right upper lobe artery and (b) implantation of a 3.5-mm magnesium scaffold.

Figure 4. Patient weighing 4 kg with pulmonary atresia with ventricular septal defect presented with supra-systemic right ventricular pressures because of severe bifurcational stenoses after right ventricular to pulmonary artery conduit implantation (a) – two magnesium scaffold 3.5 * 15 mm (black arrows) were implanted into left and right pulmonary artery (b). Follow-up at 1.3 months (more cranial projection) (c) and implantation of two Formula stents ((5 * 12 mm, Cook Medical, white arrows) (d)).

Figure 5. Patient with pulmonary atresia after unifocalisation, stent implantation to the left and right pulmonary artery. Because of severe intimal proliferation (a) to the left pulmonary artery, a magnesium scaffold 3.5 mm was implanted (b). This ensemble was redilated 5 weeks later ((c) pre-redilation) with a 6-mm Paclitaxel-covered balloon.

Figure 6. Female patient at the age of 4 weeks after Norwood I operation with a modified Blalock–Taussig shunt from the brachiocephalic trunk with a stenosis/distortion of the trunk (arrows in a), after implantation of a 3.5-mm magnesium scaffold (b). Glenn anastomosis was completed at the age of 4 months without the need to address the brachiocephalic trunk (c) (black arrows mark the former magnesium scaffold location, white arrow – surgical clip to close the modified Blalock–Taussig shunt, some coils in the right arteria mammaria to occlude aorto to pulmonary collateral arteries).
Indications
Indications and rationale to use a magnesium scaffold for this patient group:
Severe restenosis or subtotal occlusion of pulmonary veins after surgical correction (magnesium scaffolds n = 8)
Where conventional or drug-coated (Paclitaxel-covered balloons) ballooning did not show a lasting effect
Ballooning alone did not show the necessary immediate decompression
Repeated redilations showed only short-term success of 1–4 weeks
Severe pulmonary artery branch stenosis where later surgical angioplasty would be complicated by a bare metal stent (n = 2)
Severe pulmonary artery bifurcational stenoses within 24 hours after conduit implantation, with the aim to decompress the right ventricle while applying only low pressures to the stenotic area not to disrupt the fresh sutures (n = 2) (Figure 4)
Severe in-stent stenosis for which the combination of stent and cytostatic medication is considered superior to either a bare metal coronary stent or a drug-eluting balloon (n = 1)
Repeated thrombotic occlusion with the need for recanalisation of the innominate vein (n = 1)
Relevant stenosis of an aortic branch vessel feeding a modified Blalock–Taussig shunt and expected to outgrow conventional stent diameters even with maximum redilations (n = 1).
All planned interventions were submitted to and approved by our clinic’s Medical Board and Ethics Committee working on innovative approaches, which is staffed by paediatric oncologists and transplant physicians to evaluate the role of rapamycin in this local application. Before each procedure, the responsible cardiac surgeon was consulted regarding the choice between a surgical procedure and implantation of a conventional bare metal coronary stent or a magnesium scaffold. All magnesium scaffold implants were extensively discussed with the parents, stressing the possible consequences of applying an antiproliferative drug in a growing subject. Only after informed consent was obtained from the patients’ parents, the procedure was performed.
Implantation procedure
The implantation procedure of the magnesium scaffold differs from the procedure of conventional coronary stents, as the magnesium scaffold itself is invisible on X-ray (not radio-opaque). Optimal delineation of the intended landing zone is mandatory. The delivery balloon catheter, as well as the scaffolds, has tiny titanium markers on both ends to be visible in situ (Figure 1). Proper scaffold placement is documented by angiography. The functional patency of the magnesium scaffold is confirmed by angiography of the stented vessel, for example, X-ray, CT, or MRI. If the vascular wall and the vessel diameter are accessible to 2D and Doppler echocardiography or intravascular ultrasound, this is an alternative tool to monitor the physical status of the magnesium scaffold.
Anticoagulation regime was an intravenous application of 75 IU/kg of heparin during the procedure, followed by 400 IU/kg for the first day and 200 IU/kg for the second day. In all venous implantations, the patients were set on warfarin (INR control 2–2.5) for at least 6 months, and for arterial implantations, the patients received acetylsalicylic acid 2–5 mg/kg for 3 months.
Results
All 15 scaffolds were successfully implanted as intended, and proper placement and effective stenting were documented by notch-free inflation of the delivery balloon and by selective angiography. No procedure-related complications occurred.
A first follow-up angiography was performed, when clinically indicated, between 1 and 304 days (mean 76 days, standard deviation 77 days) post-implantation and showed open vessels in all, but the magnesium scaffold in the innominate vein which thrombosed early after stenting. This patient died after 1.3 weeks in progressive multi-organ failure (Pat6, Table 1). A second patient, treated for left-sided pulmonary venous stenosis with two magnesium scaffolds, died after 3.2 months during anaesthesia for a minor surgical intervention (Pat1, Table 1).
With the dissolution of the magnesium scaffold, the native form of the stented vessels reinstated itself stepwise, beginning after 4–6 weeks. This was seen by echocardiography after 30–48 days [mean 42 days]. There were no instabilities found before day 30th after implantation.
The mean time interval between implantation and the first control angiography was 2.5 months. After this time interval, the arterial implantations (n = 6) showed a minimum/maximum diameter of the stent area of 89%/99% in relation to the original scaffold diameter, with a relative diameter of 66%/77% in the pulmonary venous implantations.
No magnesium scaffold restricted the growth of the vessels nor further interventions or surgical procedures. The magnesium scaffold in the brachiocephalic trunk did not require any reintervention. This patient underwent Glenn anastomosis together with takedown of the modified Blalock–Taussig shunt 4 months later without the need to operate on the stented region (Pat3, Table 1, Figure 6). In the pulmonary circulation, bifurcational restenting with two Formula stents (Cook Medical Europe LTD., Limerick, Ireland) was required after 41 days (Pat5, Table 1, Figure 4). This patient of 4 kg weight with two magnesium scaffolds implanted showed therapeutic Sirolimus plasma levels of initially 14 µg/L, falling below 4 µg/L 2 days after the implantation (therapeutic range for renal transplantation in children ranges from 4 to 20 µg/L). Further controls showed subtherapeutic Sirolimus levels until day 17. Redilations with a much bigger drug-eluting balloon were performed for the in-stent stenosis and no reintervention was necessary in the two patients with pulmonary artery branch stenoses (Pat4 & Pat9, Table 1).
In one patient with left-sided pulmonary vein stenoses and two simultaneously implanted magnesium scaffolds, moderate, but clinically relevant restenosis occurred after 36 days, but could be effectively dilated with a drug-eluting balloon (covered with Paclitaxel) of 5 and 6 mm diameter (Pat1, Table 1). In the other two patients with pulmonary venous stenosis (Pat2 & Pat7, Table 1), two respectively four magnesium scaffolds were implanted consecutively, when an accelerated Doppler flow of more than 2.2 m/s was measured after a mean interval of 3.9 months (0.3–10 months). In this situation, a magnesium scaffold was preferred when an acute angulation of the pulmonary vein behind the left atrium (kinked orifice) could be seen in lateral projection, else redilations with a drug-eluting balloon and one in combination with a cutting balloon were completed.
Discussion
Indication and characteristics
The indication to implant a magnesium scaffold in an infant was led by the intention to solve an acute circulatory problem in a small vessel, caused by a severe stenosis while avoiding the disadvantages of a conventional coronary stent in a growing subject, which are a limited maximum diameter and unavoidable surgical explanation. In this observational study, we focused on the Magmaris scaffold, as its stability as a stent lasts long enough to overcome the acute stenosis, buying time for the patient’s condition to improve. Thereafter, further therapy at lower risk or with different materials can be considered. On the other hand, the scaffolds’ decay as a stent of 4–6 weeks does not permanently denature the native vessel. Since the degradation of the scaffold’s backbone is a multi-factorial process that depends on pulsations, local torsional and shear stresses, and chemical reactions, there is no specific time or pre-defined location for the fractures to start and how to spread along, but more a statistical probability of when the scaffold loses its supporting function. This was primarily followed by echocardiography, but complemented by angiography and further interventions in the catheter laboratory if necessary.
If required, all scaffolds are easy to fracture at any time using an oversized balloon and an inflation pressure of more than four atmospheres. As a consequence, bioabsorbable scaffolds may not limit vascular growth, even if they are not fully absorbed.
Drug coverage
All bioabsorbable scaffolds are covered with an antiproliferative drug to reduce intimal proliferation and early restenosis. The magnesium scaffold is covered with rapamycin. Rapamycin inhibits activation of T cells and B cells by reducing their sensitivity to interleukin-2 through mechanistic target of rapamycin inhibition,Reference Mukherjee and Mukherjee10 and its immunosuppressive characteristics were considered an obstacle for the use in infants.Reference Sallmon, Berger, Cho and Opgen-Rhein11 Following literature review and discussions on the use of rapamycin in transplant medicine, its effect on cell proliferation is considered reversible and severe adverse reactions are seen only at mid- and long-term systemic treatment.Reference Dambrin, Klupp and Birsan12–Reference Uhlmann, Weber and Ludwig17 There were no clinical signs of adverse effects connected to the scaffold implantation in this patient group including Pat5 with therapeutic Sirolimus levels for 2 days. During follow-up, hepatic, renal, and haematopoietic parameters were monitored, but did not show pathologic values.
In five patients with either pulmonary vein restenosis or in-stent stenosis, drug-eluting balloons were used before or after the magnesium scaffold implantation, so a therapeutic local cytostatic administration was intended to treat the lesion in those patients. For the other four patients, a rapamycin-free scaffold would have been the first choice, but was not available.
Locations
The magnesium scaffold led to acceptable early results and acute relief of clinical symptoms after implantation in severe forms of pulmonary vein stenoses. With progressive degradation, restenosis occurred and no lasting effects were observed. Repeated implantation at the same location kept the stented vessel open, while other pulmonary vein in the same patient occluded irreversibly. But on the long-term course, several reinterventions were necessary.
Implantation in a stenotic central vein with low blood flow did thrombose early. The use of a magnesium scaffold, which has antithrombotic qualities,Reference Lipinski, Acampado and Cheng18 was thought to better resist occlusion in this setting, but did not. We propose that this occurred as a consequence of severely limited flow through the feeding vein and the scaffold, as well as the presence of pre-existing collateral veins.
In the brachiocephalic trunk with pulsed high-flow perfusion, the magnesium scaffold showed a good result. Even months after the degradation, the dissection of the modified Blalock–Taussig shunt and construction of a bidirectional Glenn shunt was not hindered nor was any intervention at the stented site necessary.Reference Brandt-Wunderlich, Ruppelt and Zumstein19,Reference Hideo-Kajita, Garcia-Garcia and Haude20 In another centre and similar to earlier results,Reference Schranz, Zartner, Michel-Behnke and Akinturk2 the new magnesium scaffold has not produced a lasting positive result in coarctations in newborns,Reference Sallmon, Berger, Cho and Opgen-Rhein11 where ductal tissue may play a role.
Similarly, good results were found in pulmonary arteries where all stented vessels remained open and later redilations or implantation of larger stents were possible without the limitations of a coronary stent.
As conventional bare metal stents are available covering a broader range of diameters such as the Formula stent or a breakable stent (Babystent®, Osypka, Rheinfelden, Germany), these should be considered as first choice to resolve vascular stenosis in children, as they provide a stable and ongoing support and can be redilated according to the patient’s growth. The available diameters of the magnesium scaffold are limited to 3 and 3.5 mm, which reduces their application to small vessels or side branches in infants or very tight stenoses.
Conclusion
The magnesium scaffold is a reliable solution to stent very tight vessel stenosis in small patients. As it will dissolve within 4–6 weeks and does not cause permanent damage to the endothelial tissue layer, it may support vascular remodelling and may not hinder possible vessel growth or consecutive interventions. The results seem to be better in the arterial than in the venous systems. In the difficult setting of pulmonary vein restenosis, the magnesium scaffold is not a lasting solution despite its rapamycin coverage, but can be helpful to improve the acute clinical situation and gain some time. Serious side effects have not been observed. The use of a magnesium scaffold as a bridging solution can be considered in infants and small children, but the small diameter of the scaffolds available reduces the field of application.
Acknowledgement
None.
Financial Support
There is no grant or funding from any funding agency, commercial or not-for-profit sectors related to this research.
Conflicts of Interest
The authors declare that there is no conflict of interest.
Ethical Standards
This retrospective observation was approved by our institutional ethics board at the German Heart Centre and all procedures contributing to this work comply with the ethical standards of the relevant national guidelines on human experimentation and with the Helsinki Declaration of 1975, as revised in 2008.