Introduction
Orthocentrinae (Hymenoptera, Ichneumonidae) is a group of small-bodied, koinobiont endoparasitoids of fungus gnats (Diptera: Sciaroidea) (Broad et al. Reference Broad, Shaw and Fitton2018). Some of their hosts are known to cause damage on orchid roots and shiitake crops, which might have their populations reduced by the action of these parasitoids (Humala et al. Reference Humala, Kruidhofb and Woelke2017; Mukai and Kitajima Reference Mukai and Kitajima2019). Despite recent efforts studying the diversity of this subfamily in the Neotropical region (Humala Reference Humala2010, Reference Humala2014, Reference Humala2017, Reference Humala2019; Veijalainen et al. Reference Veijalainen, Wahlberg, Broad, Erwin, Longino and Sääksjärvi2012, Reference Veijalainen, Broad and Sääksjärvi2014a; Zwakhals and Diller Reference Zwakhals and Diller2015; Humala and Ruiz-Cancino Reference Humala and Ruíz-Cancino2017; Camargo et al. Reference Camargo, Shimbori and Penteado-Dias2020), a large taxonomic gap regarding this fauna remains, mainly as a result of a high diversity combined with small body size (Quicke Reference Quicke2012).
Orthocentrinae may represent one of the most abundant and species-rich groups of ichneumon wasps in moist tropical forests (Quicke Reference Quicke2015). For example, Orthocentrinae and Cryptinae were the two most abundant subfamilies in Amazonian Ecuador and Peru, with Orthocentrinae comprising 15–34% of the collected ichneumonids (Veijalainen et al. Reference Veijalainen, Sääksjärvi, Erwin, Gómez and Longino2013), and in Central America, Orthocentrinae was third in abundance and fourth in species richness within Ichneumonidae subfamilies (Veijalainen et al. Reference Veijalainen, Sääksjärvi, Tuomisto, Broad, Bordera and Jussila2014b). The first publication highlighting the diversity of the Neotropical Orthocentrinae was a faunistic assessment in which 1549 specimens were analysed using morphological and molecular approaches, resulting in 177 morphospecies from Ecuador and Central America (Veijalainen et al. Reference Veijalainen, Wahlberg, Broad, Erwin, Longino and Sääksjärvi2012).
Such studies are instrumental to unravelling the diversity of ichneumonids in the Neotropics, and they corroborate the perception that theories regarding latitudinal patterns of distribution (e.g., Owen and Owen Reference Owen and Owen1974; Janzen Reference Janzen1981; Askew and Shaw Reference Askew, Shaw, Waage and Greathead1986; Gauld Reference Gauld1986, Reference Gauld1987) depend on substantial accumulation of information from tropical regions to be relevant (Quicke Reference Quicke2012). In fact, recent studies on latitudinal patterns repeatedly show higher diversity in the tropics for most ichneumonids (Veijalainen et al. Reference Veijalainen, Wahlberg, Broad, Erwin, Longino and Sääksjärvi2012, Reference Veijalainen, Sääksjärvi, Erwin, Gómez and Longino2013; Timms et al. Reference Timms, Schwarzfeld and Sääksjärvi2015; Shimizu et al. Reference Shimizu, Broad and Maeto2020). Local intensive faunistic assessments of the Ichneumonoidea along elevational gradients have been helpful in understanding diversity patterns in this group, which most commonly peaks at mid-elevations in tropical mountains (e.g., van Noort Reference van Noort2004; Peck et al. Reference Peck, Banko, Schwarzfeld, Euaparadorn and Brinck2008; Veijalainen et al. Reference Veijalainen, Sääksjärvi, Tuomisto, Broad, Bordera and Jussila2014b), a pattern predicted by the “mid-domain effect” (Colwell and Lees Reference Colwell and Lees2000). High diversity in lowlands has also been reported (Sääksjärvi et al. Reference Sääksjärvi, Haataja, Neuvonen, Gauld, Jussila, Salo and Burgos2004; Aguirre et al. Reference Aguirre, Shaw and Rodríguez-Jiménez2018). Richness peaks at low elevations may be predicted by Rapoport’s rule, as a result of increasing range size with increasing elevations or latitudes (Stevens Reference Stevens1992). The rule was first formulated after observation of patterns of species distribution along latitudinal gradients (Stevens Reference Stevens1989) and later applied for elevational patterns (Stevens Reference Stevens1992). According to the rule, both for latitude and elevation, the lower bands would present higher species richness as a result of narrower ranges (explained by their physiological constrain) of the species evolving in warmer and more stable climate. Despite these general patterns, some degree of idiosyncrasy is expected, and richness peaks may vary depending on the group studied (e.g., Gauld Reference Gauld1988, Reference Gauld1991, Reference Gauld1997, Reference Gauld2000; Gauld et al. Reference Gauld, Godoy, Sithole and Ugalde Gomez2002; Aguirre et al. Reference Aguirre, Shaw and Rodríguez-Jiménez2018).
The taxonomic history of the Orthocentrinae is complex, with several changes in classification and synonyms at genus and subfamily level. The genera that currently belong to Orthocentrinae include taxa previously classified as Microleptinae, Oxytorinae, and Helictinae (Townes Reference Townes1971; Gauld Reference Gauld1984, Reference Gauld1991; Gupta Reference Gupta1988; Wahl Reference Wahl1990; Dasch Reference Dasch1992). Whether the Cylloceriinae would be better placed as a tribe within Orthocentrinae or as a separate subfamily continues to be debated (Broad et al. Reference Broad, Shaw and Fitton2018). In fact, Klopfstein et al. (Reference Klopfstein, Langille, Spasojevic, Broad, Cooper, Austin and Niehuis2019) recently confirmed a close relationship between Cylloceriinae and Orthocentrinae, which form a clade including the Diacritinae. Klopfstein et al. (Reference Klopfstein, Langille, Spasojevic, Broad, Cooper, Austin and Niehuis2019) also transferred the genus Hemiphanes Förster from Orthocentrinae to Cryptinae.
Currently, the approximately 500 described Orthocentrinae species are distributed among 29 genera (Villemant et al. Reference Villemant, Jingxian and Rousse2016; Yu et al. Reference Yu, van Achterberg and Horstmann2016; Klopfstein et al. Reference Klopfstein, Langille, Spasojevic, Broad, Cooper, Austin and Niehuis2019) that are separated into two groups: the Orthocentrus genus group and the Helictes genus group (Broad et al. Reference Broad, Shaw and Fitton2018). In the Neotropical region, 109 species in 17 genera are known; of these, only seven species in four genera have been recorded in Brazil (Veijalainen et al. Reference Veijalainen, Wahlberg, Broad, Erwin, Longino and Sääksjärvi2012; Yu et al. Reference Yu, van Achterberg and Horstmann2016; Humala Reference Humala2017, Reference Humala2019; Humala and Ruiz-Cancino Reference Humala and Ruíz-Cancino2017; Camargo et al. Reference Camargo, Shimbori and Penteado-Dias2020). Here, we present the first comprehensive survey of the Orthocentrinae in Brazil that focuses on high-elevation sites (for Brazilian standards), characterising the fauna at the genus level and estimating its diversity along an elevational gradient based on molecular operational taxonomic units inferred by DNA barcoding analysis. An illustrated identification key to the Brazilian genera of Orthocentrinae is provided.
Material and methods
Field sampling
Samples were taken for one week, during the rainy season of 2015 (between October and December), using sweep nets, light traps, Malaise traps, and Moericke traps, as part of a research project on elevational gradients in parasitoid wasp faunas. Sampling sites were located in four conservation units in mountainous regions of the Brazilian Atlantic forest (Table 1): Reserva Privada do Patrimônio Cultural de Itamonte, Itamonte, Minas Gerais state (1400–2150 m); Reserva Biológica da Serra do Japi, Jundiaí, São Paulo state (896–1190 m); Parque Nacional da Serra da Bocaina, São José do Barreiro, São Paulo state (1149–1970 m); and Parque Nacional do Itatiaia, Itatiaia, Rio de Janeiro state (786–2451 m). Additional material, collected with Moericke traps at Parque Estadual da Serra do Mar, São Luis do Paraitinga São Paulo state, was also examined. There, samples were taken during one week per month for one year between April 2012 and April 2013 at elevations ranging from 961 to 1004 m. A map (Fig. 1) with the study locations was generated using SimpleMappr (Shorthouse Reference Shorthouse2010).
Table 1. Sampling sites, including geographic coordinates, elevations, and methods. Conservation units are abbreviated: PNI, Parque Nacional de Itatiaia; RBSJ, Reserva Biológica da Serra do Japi; PESM, Parque Estadual da Serra do Mar; RPPCI, Reserva Privada do Patrimonio Cultural de Itamonte; PNSB, Parque Nacional da Serra da Bocaina; followed by the state abbreviation: MG, Minas Gerais; RJ, Rio de Janeiro; SP, São Paulo.


Fig. 1. Map with locations of the five collection sites sampled in this study.
Identification
The material was identified to genus level using keys presented by Townes (Reference Townes1971), Dasch (Reference Dasch1992), and Broad (Reference Broad2010). The morphological terminology follows Gauld (Reference Gauld1991). Identification of Pantisarthrus Förster, 1871 at species level follows Camargo et al. (Reference Camargo, Shimbori and Penteado-Dias2020). The specimens were preserved in ethanol and dried using a critical point dryer (Leica EM-CPD030; Leica, Wetzlar, Germany). Digital scanning electron microscopy photographs were taken with a FEI Quanta 250 scanning electron microscope (Thermo Fisher Scientific, Waltham, Massachusetts, United States of America) in a low-vacuum mode of uncoated specimens. Colour digital photographs were taken with a Leica MC 170 high-definition video camera attached to a Leica M205C with a planapo 1.0× objective and edited using Adobe Photoshop (Adobe, San Jose, California, United States of America). The specimens are deposited at the DCBU Collection (Departamento de Ecologia e Biologia Evolutiva da Universidade Federal de São Carlos, campus São Carlos, Brazil). The genus Sphingozona Townes, 1971 was not collected, despite being known from Brazil. It was included in the key based on the original description (Townes Reference Townes1971).
Laboratory procedures and analysis
A total of 459 randomly selected specimens had whole-body genomic DNA extracted in a nondestructive method using the DNeasy Blood & Tissue Kit (QIAGEN Inc., Valencia, California, United States of America) and following the manufacturer’s instructions. Subsequent DNA purification was performed by ethanol precipitation (Sambrook and Russell Reference Sambrook and Russell2001), and then DNA extracts were resuspended in 50 mL of Tris–EDTA buffer. A cytochrome oxidase 1 (CO1) mitochondrial DNA fragment belonging to the barcoding locus was amplified using the universal LCO 1490 and HCO 2198 primers (Folmer et al. Reference Folmer, Black, Hoeh, Lutz and Vrijenhoek1994). Polymerase chain reaction was carried out in 25-µL final volume (2.5 mM MgCl2, 2.5 mM dNTP Mix, 0.2 M each primer, 1× HOT FIREPol Buffer B1, and 1 U HOT FIREPol DNA Polymerase (Solis Biodyne, Tartu, Estonia)) and followed a cycling process of initial denaturation at 95 °C for 15 minutes; 35 cycles of denaturation at 95 °C for 45 seconds, annealing at 56 °C for 30 seconds, and extension at 72 °C for 1 minute, and then a final extension at 72 °C for 5 minutes. Polymerase chain reaction products were purified by polyethylene glycol precipitation (Lis and Schleif Reference Lis and Schleif1975) and sequenced at Macrogen, Inc. (Teheran-ro, Gangnam-gu, Seoul, Republic of Korea). Sequences were edited using the programme Sequencher 4.14 and aligned using MAFFT, version 7 (https://mafft.cbrc.jp/alignment/software/), and the phylogenetic analysis was performed using the program Mega X (Kumar et al. Reference Kumar, Stecher, Li, Knyaz and Tamura2018).
Data analyses
A neighbour-joining tree using the bootstrap method with 500 replicates was reconstructed, and a genetic distances table was generated based on the P-distance model to cluster the specimens according to their molecular operational taxonomic units. The clustering respected the boundaries at a sequence divergence of 2%, which found high concordance with the final species delimitation in previous studies (Smith et al. Reference Smith, Rodriguez, Whitfield, Deans, Janzen, Hallwachs and Hebert2008; Fernandez-Triana et al. Reference Fernandez-Triana, Whitfield, Rodriguez, Smith, Janzen and Hallwachs2014; van Achterberg et al. Reference van Achterberg, Sharkey and Chapman2014; Sharkey et al. Reference Sharkey, Chapman, Janzen, Hallwachs and Smith2015, Reference Sharkey, Meierotto, Chapman, Janzen, Hallwachs, Dapkey and Solis2018; Tucker et al. Reference Tucker, Chapman and Sharkey2015; Sharkey and Chapman Reference Sharkey and Chapman2016; Kang et al. Reference Kang, Chapman, Janzen, Hallwachs, Dapkey, Smith and Sharkey2017; Meierotto et al. Reference Meierotto, Sharkey, Janzen, Hallwachs, Hebert, Chapman and Smith2019). Sequences are deposited at the National Center for Biotechnology Information, with the following GenBank accession numbers: MT784201–MT784659, and at the Barcode of Life Database, with process ID DEBE001-21–DEBE459-21.
Sample-based species rarefaction curves and estimates of species richness (Chao 1, Jackknife 2, and ACE) were calculated using EstimateS 9.1 for Windows (Gotelli and Colwell Reference Gotelli, Colwell, Magurran and McGill2011; Colwell Reference Colwell2013). Trends in species richness were analysed using the molecular operational taxonomic units randomly pooled from the Orthocentrinae (459 specimens from 1566 total). Sampling sites were grouped into six 300-m elevation bands (700–1000, 1000–1300, 1300–1600, 1600–1900, 1900–2200, and 2200–2500). To evaluate Rapoport’s rule, the method developed by Stevens (Reference Stevens1992) was applied using the elevational range of each species to calculate the mean elevational range of all species present in each band. The mean elevational range for each band was regressed against elevation – that is, the midpoint for each of the six elevation bands. Steven’s method suffers from spatial nonindependence, as pointed out by Colwell and Hurtt (Reference Colwell and Hurtt1994), but the overall performance is similar to the quartile test designed to correct for these errors (McCain and Knight Reference McCain and Knight2013). The midpoint method (Rohde et al. Reference Rohde, Heap and Heap1993; Colwell and Hurtt Reference Colwell and Hurtt1994), which also corrects for spatial nonindependence but depends on large samples, was not used because our samples are restricted to one week.
Results
Taxonomy
Aperileptus Förster, 1869
Type species: Plectiscus albipalpus Gravenhorst, 1829.
Material examined. One specimen from Parque Estadual da Serra do Mar, São Luíz do Paraitinga, São Paulo state, Brazil (960 m).
Biology. Aperileptus species have been reported to parasitise Mycetophila alea Laffoon, 1965, Mycetophila evanida Lastovka, 1972, and Exechia bicincta (Staeger, 1840) (Diptera: Mycetophilidae) (Šedivý and Ševčik Reference Šedivý and Ševčik2003).
Distribution. Eighteen species are described from the Nearctic, Palearctic, and Afrotropical regions (Yu et al. Reference Yu, van Achterberg and Horstmann2016). The genus is recorded for the first time in Brazil and for the second time in the Neotropics (Veijalainen et al. Reference Veijalainen, Wahlberg, Broad, Erwin, Longino and Sääksjärvi2012), although no Neotropical species have yet been described.
Batakomacrus Kolarov, 1986
Type species: Batakomacrus crassicaudatus Kolarov, 1986.
Material examined. Thirty-eight specimens from Reserva Privada do Patrimônio Cultural de Itamonte, Minas Gerais state, Brazil (1399–2149 m); 15 specimens from Reserva Biológica da Serra do Japi, Jundiaí, São Paulo state, Brazil (895–1134 m); nine specimens from Parque Nacional da Serra da Bocaina, São José do Barreiro, São Paulo state, Brazil (1446–1969 m); four specimens from Parque Nacional de Itatiaia, Rio de Janeiro state, Brazil (786–1652 m).
Biology. Unknown
Distribution. Eight species are described, of which seven are Palearctic (Yu et al. Reference Yu, van Achterberg and Horstmann2016). The only Neotropical species, Batakomacrus hidalgo Humala, 2010, is from Mexico (Humala Reference Humala2010). This is the first record of the genus in Brazil despite the apparent commonness of the genus.
Comments. The identification of the genus is difficult, and no autapomorphic characters define Batakomacrus (Broad Reference Broad2010). Male specimens previously identified as Picrostigeus Förster, 1869 based on the parameres (apex level with the apex of the metasomal tergites, following Broad (Reference Broad2010)) were clustered by DNA barcoding with females identified as Batakomacrus, based mainly on the ovipositors, which are very distinctive in both genera. This result indicates that males from the Neotropics have more variable genitalia than are reported from the Palearctic fauna and could not be reliably identified using morphology, based on current knowledge. Therefore, a revision including the Neotropical fauna is recommended.
Catastenus Förster, 1869
Type species: Catastenus femoralis Förster, 1871.
Material examined. One specimen from Parque Estadual da Serra do Mar, São Luiz do Paraitinga, São Paulo state, Brazil (960 m).
Biology. Unknown
Distribution. Four species are described in this genus, recorded from the Palearctic, Nearctic, Afrotropical, and Neotropical regions (Yu et al. Reference Yu, van Achterberg and Horstmann2016; Watanabe Reference Watanabe2019). The only Neotropical species is Catastenus rufithoracicus (Cresson, 1868), from Cuba (Yu et al. Reference Yu, van Achterberg and Horstmann2016). This is the first record of the genus in Brazil.
Chilocyrtus Townes, 1971
Type species: Chilocyrtus carinatus Townes, 1971.
Material examined. Four specimens from Reserva Biológica da Serra do Japi, Jundiaí, São Paulo state, Brazil (895–1134 m); 37 specimens from Reserva Privada do Patrimônio Cultural de Itamonte, Minas Gerais state, Brazil (1399–2149 m); five specimens from Reserva Nacional da Serra Bocaina, São José do Barreiro, São Paulo state, Brazil (1149–1969 m); two specimens from Parque Nacional do Itatiaia, Rio de Janeiro state, Brazil (786–1652 m); 75 specimens from Reserva Estadual da Serra do Mar, São Luiz do Paraitinga, São Paulo state, Brazil (906–1004 m).
Biology. Unknown.
Distribution. Two species are described: C. carinatus Townes, 1971 from the Neotropical region, including Brazil, and C. hortorum (Seyrig, 1934) from the Afrotropical region (Yu et al. Reference Yu, van Achterberg and Horstmann2016).
Eusterinx Förster, 1869
Type species: Eusterinx oligomera Förster, 1871.
Material examined. Thirteen specimens from Parque Nacional do Itatiaia, Rio de Janeiro state, Brazil (786–934 m); 15 specimens from Parque Nacional da Serra da Bocaina, São José do Barreiro, São Paulo state, Brazil (1149–1969 m); 30 specimens from Reserva Biológica da Serra do Japi, Jundiaí, São Paulo state, Brazil (890–1190 m); one specimen from Reserva Privada do Patrimônio Cultural de Itamonte, Minas Gerais state, Brazil (1443 m); five specimens from Parque Estadual Serra do Mar, São Luiz do Paraitinga, São Paulo state, Brazil (960–1002 m).
Biology. Eusterinx species are known to parasitise Mycetophilidae (Diptera) (Dasch Reference Dasch1992).
Distribution. The genus is distributed in the Palearctic, Nearctic, Oriental, and Neotropical regions (Yu et al. Reference Yu, van Achterberg and Horstmann2016). There are three Neotropical species: Eusterinx (Eusterinx) solida Dasch, 1992, Eusterinx (Divinatrix) longipes Humala and Ruiz-Cancino, 2017, and Eusterinx (Ischyracis) tenuiventris Humala and Ruiz-Cancino, 2017 (Humala and Ruiz-Cancino Reference Humala and Ruíz-Cancino2017). This is the first record of the genus in Brazil.
Gnathochorisis Förster, 1869
Type species: Gnathochorisis flavipes Förster, 1871.
Material examined. Seven specimens from Reserva Biológica da Serra do Japi, Jundiaí, São Paulo state, Brazil (895–1134 m); 71 specimens from Reserva Privada do Patrimônio Cultural de Itamonte, Minas Gerais state, Brazil (1399–1652 m); 10 specimens from Reserva Nacional da Serra Bocaina, São José do Barreiro, São Paulo state, Brazil (1149–1969 m); 10 specimens from Parque Nacional do Itatiaia, Rio de Janeiro state, Brazil (786–2451 m); six specimens from Reserva Estadual da Serra do Mar, São Luiz do Paraitinga, São Paulo state, Brazil.
Biology. Gnathochorisis flavipes is a parasitoid of Neoempheria striata (Meigen, 1818) (Diptera: Mycetophilidae) (Humala Reference Humala2003, Reference Humala2017).
Distribution. The genus is known to occur in the Palearctic, Nearctic, Afrotropical, and Neotropical regions, with nine Neotropical species, none of which are recorded in Brazil (Yu et al. Reference Yu, van Achterberg and Horstmann2016; Humala Reference Humala2017; Watanabe Reference Watanabe2020; Varga Reference Varga2021).
Megastylus Schiødte, 1838
Type species: Megastylus cruentator Schiødte, 1838.
Material examined. Eighty-six specimens from Reserva Privada do Patrimônio Cultural de Itamonte, Minas Gerais state, Brazil (1399–2149 m); 15 specimens from Reserva Biológica da Serra do Japi, Jundiaí, São Paulo state, Brazil (895–1190 m); 13 specimens from Reserva Nacional da Serra Bocaina, São José do Barreiro, São Paulo state, Brazil (1149–1969 m); five specimens from Parque Nacional do Itatiaia, Rio de Janeiro state, Brazil (786–1652 m); eight specimens from Reserva Estadual da Serra do Mar, São Luiz do Paraitinga, São Paulo state, Brazil (940–1002 m).
Biology. The species with known biology are solitary koinobiont endoparasitoids of Keroplatidae (Diptera) larvae (Humala et al. Reference Humala, Kruidhofb and Woelke2017). Megastylus woelkei Humala, 2017 was observed parasitising Keroplatidae (Diptera) larvae in orchid roots (Humala et al. Reference Humala, Kruidhofb and Woelke2017).
Distribution. Worldwide, 41 species are described, and three of these are from the Neotropics: Megastylus (Megastylus) panamensis Wahl, 1997, Megastylus (Dicolus) kasparyani Humala, 2014, and Megastylus (Dicolus) pectoralis (Förster, 1871) (Yu et al. Reference Yu, van Achterberg and Horstmann2016; Zhang et al. Reference Zhang, Zhou, Li and Sheng2022). This is the first record of the genus from Brazil.
Orthocentrus Gravenhorst, 1829
Type species: Orthocentrus anomalus Gravenhorst, 1829.
Material examined. Fifty-six specimens from Reserva Biológica da Serra do Japi, Jundiaí, São Paulo state, Brazil (895–1190 m); 260 specimens from Reserva Privada do Patrimônio Cultural de Itamonte, Minas Gerais state, Brazil (1399–2149 m); 31 specimens from Reserva Nacional da Serra Bocaina, São José do Barreiro, São Paulo state, Brazil (1149–1969 m); 40 specimens from Parque Nacional do Itatiaia, Rio de Janeiro state, Brazil (786–2451 m); 124 specimens from Reserva Estadual da Serra do Mar, São Luiz do Paraitinga, São Paulo state, Brazil (906–1004 m).
Biology. An Orthocentrus species was recorded emerging from the host cocoon of Sciophila Meigen, 1818 (Diptera: Mycetophilidae) (Šedivý and Ševčik Reference Šedivý and Ševčik2003). Mukai and Kitajima (Reference Mukai and Kitajima2019) reported Orthocentrus sp. parasitising Neoempheria Osten Sacken, 1878 (Diptera: Mycetophilidae) on shiitake mushrooms, providing an effective biological control of Neoempheria carinata Sueyoshi, 2014.
Distribution. There are 154 described species for all regions. In the Neotropical region, 79 species are reported, but only two of these are recorded from Brazil (Veijalainen et al. Reference Veijalainen, Broad and Sääksjärvi2014a; Zwakhals and Diller Reference Zwakhals and Diller2015; Humala Reference Humala2019).
Pantisarthrus Förster, 1871
Type species Pantisarthus inaequalis Förster, 1871.
Material examined. Thirty specimens from Reserva Privada do Patrimônio Cultural de Itamonte, Minas Gerais state, Brazil (1412–1605 m); two specimens from Reserva Estadual da Serra do Mar, São Luiz do Paraitinga, São Paulo state, Brazil (961–1004 m).
Biology. Pantisarthrus lubricus (Förster, 1871) is a parasitoid of Phronia strenua (Diptera: Mycetophilidae) (Roman, 1923), and P. luridus Förster, 1871 is a parasitoid of Cordyla nitidula Edwards, 1925 and Cordyla fusca Meigen, 1804 (Diptera: Mycetophilidae) (Šedivý and Ševčik Reference Šedivý and Ševčik2003).
Distribution. The genus occurs in the eastern and western Palaearctic, Nearctic, Oceanic (Mascarenes Island), and Neotropical regions (Yu et al. Reference Yu, van Achterberg and Horstmann2016). Three species are known in Brazil: P. communis Camargo and Penteado-Dias, 2020, P. flavocingulatus Camargo and Penteado-Dias, 2020, and P. paraitinga Camargo and Penteado-Dias, 2020 (Camargo et al. Reference Camargo, Shimbori and Penteado-Dias2020).
Comments. The Pantisarthrus molecular operational taxonomic unit was identified as Pantisarthrus flavocingulatus Camargo and Penteado-Dias, 2020 (Camargo et al. Reference Camargo, Shimbori and Penteado-Dias2020).
Plectiscus Gravenhorst, 1829
Type species: Plectiscus impurator Gravenhorst, 1829.
Material examined. Twenty-eight specimens from Reserva Privada do Patrimônio Cultural de Itamonte, Minas Gerais state, Brazil (1399–2149 m); one specimen from Reserva Biológica da Serra do Japi, Jundiaí, São Paulo state, Brazil (1036–1134 m); six specimens from Reserva Nacional da Serra Bocaina, São José do Barreiro, São Paulo state, Brazil (1446–1969 m); four specimens from Reserva Nacional do Itatiaia, Rio de Janeiro state, Brazil (1443–2451 m); 51 specimens from Parque Estadual da Serra do Mar, São Luiz do Paraitinga, São Paulo state, Brazil (940–1003 m).
Biology. Two Plectiscus species were recorded emerging from the pupae of Bradysia giraudii (Egger, 1862) (Diptera: Sciaridae) (Broad et al. Reference Broad, Shaw and Fitton2018).
Distribution. Eleven species are described from the Afrotropical, Nearctic, and Palearctic regions (Yu et al. Reference Yu, van Achterberg and Horstmann2016). Even though this genus is known to occur in the Neotropics (Veijalainen et al. Reference Veijalainen, Wahlberg, Broad, Erwin, Longino and Sääksjärvi2012), to date, no species are described from this region. This is the first record from Brazil.
Proclitus Förster, 1869
Type species: Proclitus grandis Förster, 1871.
Material examined. Nine specimens from Reserva Privada do Patrimônio Cultural de Itamonte, Minas Gerais state, Brazil (1399–1606 m); one specimen from Reserva Biológica da Serra do Japi, Jundiaí, São Paulo state, Brazil (1183–1190 m); six specimens from Reserva Nacional da Serra Bocaina, São José do Barreiro, São Paulo state, Brazil (1446–1528 m); seven specimens from Reserva Nacional do Itatiaia, Rio de Janeiro state, Brazil (786–2451 m); 95 specimens from Parque Estadual da Serra do Mar, São Luiz do Paraitinga, São Paulo state, Brazil (934–1002 m).
Biology. Proclitus species are known to parasitise Cordyla fusca Meigen, 1804, Mycetophila finlandica Edwards, 1913, and Allodia zaitzevi Kurina, 1998 (Diptera: Mycetophilidae) (Šedivý and Ševčik Reference Šedivý and Ševčik2003).
Distribution. The genus is found worldwide. Two species are described from the Neotropical region: Proclitus floridanus Dasch, 1992 and P. fulvicornis Förster, 1871, neither of which occurs in Brazil (Yu et al. Reference Yu, van Achterberg and Horstmann2016).
Stenomacrus Förster, 1869
Type species: Orthocentrus silvaticus (Holmgren, 1858).
Material examined. There are 220 specimens from Reserva Privada do Patrimônio Cultural de Itamonte, Minas Gerais state, Brazil (1399–2149 m); 54 specimens from Reserva Biológica da Serra do Japi, Jundiaí, São Paulo state, Brazil (895–1190 m); 32 specimens from Reserva Nacional da Serra Bocaina, São José do Barreiro, São Paulo state, Brazil (1149–1969 m); 19 specimens from Reserva Nacional do Itatiaia, Rio de Janeiro state, Brazil (786–1652 m); six specimens from Parque Estadual da Serra do Mar, São Luiz do Paraitinga, São Paulo state, Brazil (934–1002 m).
Biology. Stenomacrus meijeri Humala, 2020 was reported rearing from Bradysia impatiens (Johannsen, 1912) (Diptera: Sciaridae) (Woelke et al. Reference Woelke, Pham and Humala2020), and Stenomacrus curvulus (Thomson, 1897) was reported from Trichosia sinuata Menzel and Mohrig, 1997 (Diptera: Sciaridae) (Vilkamaa and Komonen Reference Vilkamaa and Komonen2001).
Distribution. The genus has 74 described species distributed in the Palearctic, Nearctic, Oceanic, Australasian, and Neotropical regions (Yu et al. Reference Yu, van Achterberg and Horstmann2016; Woelke et al. Reference Woelke, Pham and Humala2020). The single Neotropical species, Stenomacrus meridionalis Ashmead, 1900, is known only from the type locality in the Caribbean (Yu et al. Reference Yu, van Achterberg and Horstmann2016). This is one of the most common genera found in our samples. Nevertheless, this is the first record of Stenomacrus in Brazil.
Symplecis Förster, 1869
Type species: Symplecis alpicola Förster, 1871.
Material examined. Fourteen specimens from Reserva Privada do Patrimônio Cultural de Itamonte, Minas Gerais state, Brazil (1399–1606 m); one specimen from Reserva Nacional da Serra Bocaina, São José do Barreiro, São Paulo state, Brazil (1446 m); four specimens from Parque Estadual da Serra do Mar, São Luiz do Paraitinga, São Paulo state, Brazil (934–1002 m).
Biology. Symplecis breviuscula Roman, 1923 is a parasitoid of Diadocidia ferruginosa Meigen (Diptera: Diadocidiidae), and Symplecis matilei Delobel, 1975 parasitises Neoempheria ombrofila, Matile, 1975 (Diptera: Mycetophilidae) (Delobel and Matile Reference Delobel and Matile1975; Humala et al. Reference Humala, Choi and Lee2016).
Distribution. The genus has a worldwide distribution, with 16 described species. There is only one Neotropical species, Symplecis laticinta (Cresson, 1868), from Mexico (Yu et al. Reference Yu, van Achterberg and Horstmann2016). This is the first record from Brazil.
Key to Brazilian genera of Orthocentrinae (based on Townes Reference Townes1971, Dasch Reference Dasch1992, and Broad Reference Broad2010)
-
1. Clypeus not separated from face by a groove, making the face uniformly convex (Fig. 2A) .... 2
-
–. Face not uniformly convex, clypeus evident, separated from face by a groove (Fig. 2B)..... 6
-
2. Mandibles hardly overlapping, labrum never exposed; vein cu-a on hind wing mostly reclined, sometimes vertical; ovipositor short, never surpassing the metasoma apex (Fig. 2A, C) .............................................................................................................................................. Orthocentrus
-
–. Mandibles overlapping when closed, labrum exposed; vein cu-a on hind wing inclivous or vertical; ovipositor variable ................................................................................................................... 3
-
3. Epicnemial carina absent; ovipositor short and straight (Fig. 2D)............................. Plectiscus
-
–. Epicnemial carina present, ovipositor variable (Fig. 2E)............................................................. 4
-
4. Mandibles with lower tooth present and visible in antero-ventral view; with the following combination of characters: vein cu1 on hind wing present; female with ovipositor not so long, upcurved, ovipositor sheaths, and metasoma capable of rotational movements; male with apex of parameres usually before metasoma apex, metasoma elongate, T3 usually longer than T2 (Figs. 3A, 4A, B) ............................................................................................................. Batakomacrus
-
–. Mandibles rotated, if lower tooth present, it is located on the inner face of the mandible; without the combination of characters above (Fig. 3B, C)........................................................... 5
-
5. Clypeus with a transverse convexity on ridge and with apex incurved in a concave way, propodeum without median longitudinal carinae (Figs. 3C, 4C) ........................... Chilocyrtus
-
–. Clypeus without a transverse convexity on ridge and with apex incurved but not concave, flat; propodeum usually with median longitudinal carinae (Figs. 3B, 4D) ......................... Stenomacrus
-
6. Sternite of first metasomal segment separated from tergite, usually with posterior end at or in front of spiracle; glymma present (Fig. 4E)..................................................................................... 7
-
–. Sternite of first metasomal segment fused to tergite, usually with posterior end at or behind spiracle; glymma absent (Fig. 4F) ...................................................................................................... 8
-
7. Propodeum only with posterior portion of lateral longitudinal carinae; ovipositor long, 1.45–2.55 × hind tibia length (Fig. 4G, H) ................................................................. Aperileptus
-
–. Propodeum with more complete carination, only anterior transverse carina absent, and median longitudinal is present only in some species; ovipositor short, 0.16–0.5 × length of hind tibia (Fig. 5A, B) ....................................................................................................Pantisarthrus
-
8. Epipleura of second and third metasomal tergites not separated from terga by a crease; with the following combination of characters: areolet absent; vein cu1 of hind wing absent; antenna with very long flagellum; clypeus foveae as impressed areas, not open; propodeum with a deep impression towards the anterior and a prolonged anterior section Sphingozona
-
–. Epipleura of second and in some cases third metasomal tergites separated from terga by a crease; without the combination of characters above (Fig. 5C) .................................................. 9
-
9. Scape inflated, hind margin membranous; areolet never present; ovipositor short (Fig. 5D, E)...................................................................................................... Megastylus
-
–. Scape variable, but not inflated and without hind margin membranous; areolet present or absent; ovipositor variable .................................................................................................................. 10
-
10. Mandibles twisted, only one tooth visible; with the following combination of characters: antennae with 14–24 segments; vein cu1 of hind wing absent; ovipositor 0.5–1.25 × hind tibia length (Fig. 6A) ....................................................................................................................... Eusterinx
-
–. Mandibles not twisted, both teeth visible; without the combination above......................... 11
-
11. Lateral margins of face subparallel (Fig. 6B)................................................................................ 12
-
–. Lateral margins of face convergent ventrally (Fig. 6C).............................................................. 13
-
12. Areolet absent; vein cu1 of hind wing weak; clypeus narrow to moderately long, usually wide; ovipositor 1.2–3.7 × hind tibia length, not upcurved (Fig. 7A) .................................. Proclitus
-
–. Areolet present; vein cu1 of hind wing strong; clypeus small, width 1.3–2.25 × height; ovipositor 0.65–1.10 × hind tibia length, upcurved with a subapical notch (Fig. 7B) ................................................................................................... Gnathochorisis
-
13. Ovipositor 0.65–1.75 × hind tibia length; hind tarsal claws small; hind femur length 4.0–6.10 × height (Fig. 7C) ..................................................................................... Symplecis
-
–. Ovipositor 0.4–0.6 × hind tibia length; hind tarsal claws exceptionally large; hind femur length 3.45–4.0 × height (Fig. 7D, E) .......................................................................... Catastenus

Fig. 2. A, Orthocentrus sp. head, anterior view; B, Pantisarthrus communis (Camargo et al. Reference Camargo, Shimbori and Penteado-Dias2020), head, anterior view; C, Orthocentrus sp. posterior wing; D, Plectiscus sp. mesosoma, lateral view; E, Chilocyrtus sp. mesosoma lateral view.

Fig. 3. A, Batakomacrus sp. face, anterior view; B, Stenomacrus sp. face, anterior view; C, Chilocyrtus sp. face, anterior view.

Fig. 4. A, B, Batakomacrus sp. metasoma, lateral view; C, Chilocyrtus sp. propodeum, dorsal view; D, Stenomacrus sp. propodeum dorsal view; E, Pantisarthrus communis Camargo et al., Reference Camargo, Shimbori and Penteado-Dias2020, first metasomal tergite, lateral view; F, Megastylus sp. first metasomal tergite, lateral view; G, Aperileptus sp. propodeum, dorsal view; H, Aperileptus sp. female habitus.

Fig. 5. A, Pantisarthrus paraitinga (Camargo et al. Reference Camargo, Shimbori and Penteado-Dias2020), propodeum, dorsal view; B, Pantisarthrus communis (Camargo et al., Reference Camargo, Shimbori and Penteado-Dias2020), female habitus; C, Megastylus sp. metasomal tergites 2 and 3, dorsal view; D, Megastylus sp. scape; E, Megastylus sp. female habitus.
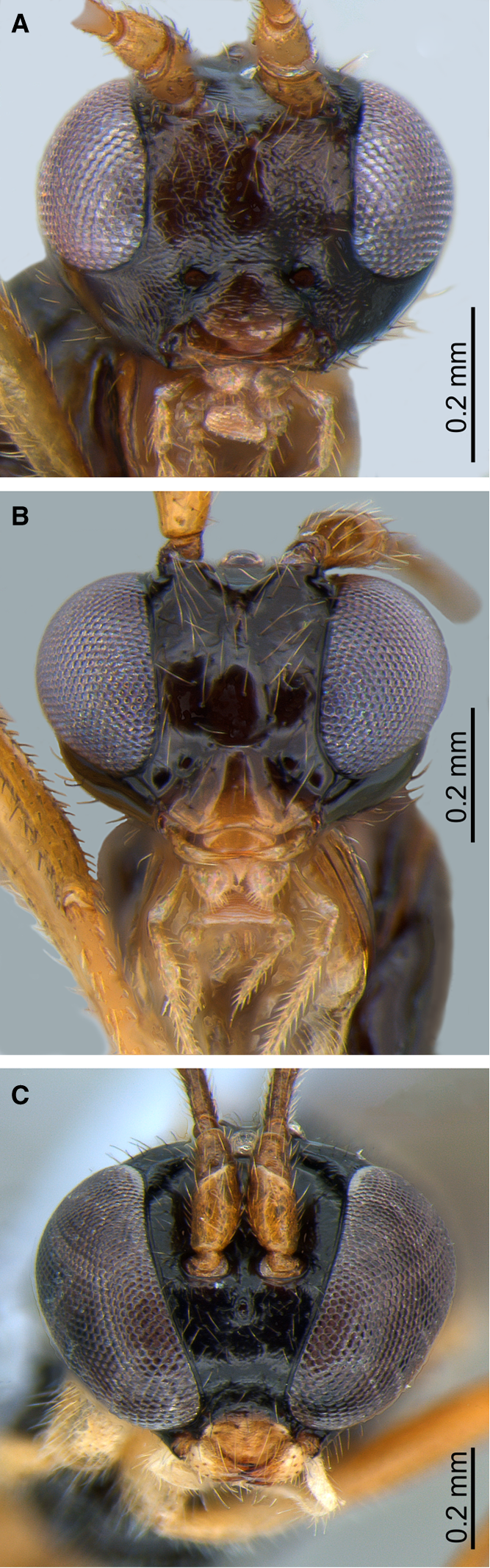
Fig. 6. A, Eusterinx sp. head, anterior view; B, Proclitus sp. face, anterior view; C, Symplecis sp. face, anterior view.

Fig. 7. A, Proclitus sp. female habitus; B, Gnathochorisis sp. female habitus; C, Symplecis sp. female habitus; D, Catastenus sp. male habitus; E, Catastenus sp. hind tarsal claw.
Faunistic analyses
The 1566 specimens of Orthocentrinae collected in our samples were identified to 13 genera (Fig. 8), of which only three were previously recorded in Brazil. These are Chilocyrtus, Orthocentrus, and Pantisarthrus (Townes Reference Townes1971; Veijalainen et al. Reference Veijalainen, Broad and Sääksjärvi2014a; Camargo et al. Reference Camargo, Shimbori and Penteado-Dias2020). The genera Aperileptus, Batakomacrus, Catastenus, Eusterinx, Gnathochorisis, Megastylus, Plectiscus, Proclitus, Stenomacrus, and Symplecis are reported here for the first time from Brazil. The most common genera sampled in this survey were Orthocentrus and Stenomacrus. Most of the Neotropical genera were represented in our samples, except for Apoclima Förster, Helictes Haliday, Plectiscidea Viereck, and Sphingozona Townes. To date, only Sphingozona has been previously recorded in Brazil.

Fig. 8. Abundance of Orthocentrinae genera in the 300-m elevational bands in Brazilian mountains: A, most abundant genera; B, least abundant genera.
Our samples covered an elevational range of 1665 m, ranging from 786 to 2451 m above sea level. The abundance of each genus, divided into six 300-m elevational bands, is shown in Figure 8. Most genera occurred throughout the entire elevational range of the study, except for the highest elevational band, where only nine specimens in four genera were collected and the most common genera were Gnathochorisis and Plectiscus. The genera Aperileptus and Catastenus were restricted to the lowest band (700–1000 m), and another two genera – Pantisarthrus and Symplecis – were not observed above 1900 m.
In the molecular analysis, 459 specimens from 10 genera were included and were found to be clustered in 127 molecular operational taxonomic units (Fig. 9). Because the molecularly analysed specimens represent roughly one-third of the collected specimens, the actual diversity should be much higher. Our results represent a subsample of the local fauna, and estimations of species richness resulted in nearly twice the observed richness for the 54 samplings (Fig. 10), meaning at least 197 species might occur in the studied area.

Fig. 9. Number of molecular operational taxonomic units in the total data set (blue bars), described Orthocentrinae species in the Neotropics (orange bars) and described Orthocentrinae species in Brazil (grey bars).

Fig. 10. Species accumulation curve (+), and sample-based rarefaction (○) and estimations of species richness curves (): Chao 1 (
); ACE (
); Jackknife 2 (
).
The elevational range for the molecular operational taxonomic units varied from sea level to 1240 m, with a mean elevational range of 241.61 ± 79.87 m (1 – α = 95%). As observed in the elevational distribution for genera, diversity dropped steeply at the highest elevational band (2200–2500 m; Fig. 11), in part due to a reduced sampling effort (only one Malaise trap; Table 1). The absence of Eusterinx above 1300 m and of Plectiscus below 1300 m is clearly an artefact of subsampling because both genera are recorded from the lowest band up to at least 2200 m. The elevational range size of the species was positively correlated with elevation, according to our linear regression using the Stevens’s (Reference Stevens1992) method (n = 06, r 2 = 0.78, P = 0.02; Fig. 12).

Fig. 11. Species (molecular operational taxonomic units) richness for each genus in six 300-m elevational bands in the Brazilian mountains (legend colour for genera is the same for all figures).

Fig. 12. Linear regression analysis based on Stevens’s (Reference Stevens1992) method, of the relationship between elevation (midpoint for each 300-m band) and the mean elevational range of the species of Orthocentrinae present in each of the six elevational 300-m bands in Brazilian mountains (P = 0.02). The increase in range with increasing elevations supports prediction of the Rapoport’s rule.
Discussion
The observed species richness of Orthocentrinae is based on molecular operational taxonomic units inferred from less than one-third of the 1566 specimens collected during a one-week survey in a small region of Brazil. We found at least 55 new species in 10 genera, meaning a potential increase of 50% in species richness for the Neotropical Orthocentrinae, compared with the 109 described species, and nearly eight times the number of species in Brazil (Fig. 9). Estimates confirm that this number represents only a fraction of the local fauna (Fig. 10) and, thus, probably a very small fraction of the Neotropical diversity. The significance of this result alone, in terms of the enormous taxonomic gap, is remarkable, and the result corroborates the works of Veijalainen et al. (Reference Veijalainen, Wahlberg, Broad, Erwin, Longino and Sääksjärvi2012, Reference Veijalainen, Sääksjärvi, Erwin, Gómez and Longino2013, Reference Veijalainen, Sääksjärvi, Tuomisto, Broad, Bordera and Jussila2014b) and Timms et al. (Reference Timms, Schwarzfeld and Sääksjärvi2015), indicating a huge and largely unknown diversity in the tropics, especially considering that this study covered only a small area of the rainy mountains in southeastern Brazil.
Smith et al.’s (Reference Smith, Fernandez-Triana, Roughley and Hebert2009) study of Orthocentrinae based on molecular operational taxonomic units from a temperate region (latitude: 58° N) also showed a high diversity and several cryptic species, suggesting that the global knowledge of the group is still incipient. The problem of cryptic species was also observed for the Neotropical Orthocentrinae (Veijalainen et al. Reference Veijalainen, Wahlberg, Broad, Erwin, Longino and Sääksjärvi2012), corroborating the importance of integrating molecular data in species identification. Our results demonstrate the adequacy of the DNA barcoding CO1 region as a taxonomic tool for the separation of species in understudied groups. For example, barcoding allowed us to identify some taxonomic problems at the genus level and to better understand the morphological diversity of the Neotropical genera (e.g., see comments for the genus Batakomacrus in the taxonomy section). On the other hand, the costs of this method are usually prohibitive, limiting the number of specimens analysed. The aforementioned reasons emphasise the need for taxonomic revisions using integrative taxonomy.
When comparing our results with Veijalainen et al.’s (Reference Veijalainen, Wahlberg, Broad, Erwin, Longino and Sääksjärvi2012) faunistic survey of Neotropical Orthocentrinae from Amazonian Peru, Ecuador, and Central America, we found most genera to be common to both surveys, with only three genera absent in the present survey (i.e., Helictes, Plectiscidea, and Sphingozona) and two not found in the earlier study (i.e., Batakomacrus and Catastenus). Orthocentrus was the most common and species-rich genus in both surveys. Interestingly, Stenomacrus was the second-most common and speciose genus in Brazil but is relatively rare in Peru, Ecuador, and Central America.
All Neotropical studies on Orthocentrinae fauna were conducted in moist environments, highlighting the need of studies in different biomes such as dry forests and savannahs, especially in a huge and megadiverse country like Brazil. For example, Shapiro and Pickering (Reference Shapiro and Pickering2000) observed that aridity negatively affects abundance of ichneumonids. They observed that Orthocentrinae are also affected by forest type, being most abundant in primary old-growth forests, which is interesting, considering their hosts are fungus gnats. Therefore, we expect to find lower diversity in drier areas, and studies in the Brazilian Cerrado and Caatinga would be important to analyse this hypothesis.
Trends in species richness inferred from Figure 11 should not be considered for comparison with previous studies nor be interpreted as representative of actual local trends, because the sampling efforts were not standardised and no samples at low elevations (below 780 m) were taken in the present survey. The elevational distribution most commonly found for ichneumonids is a mid-elevation peak in species richness (van Noort Reference van Noort2004; Peck et al. Reference Peck, Banko, Schwarzfeld, Euaparadorn and Brinck2008; Veijalainen et al. Reference Veijalainen, Sääksjärvi, Tuomisto, Broad, Bordera and Jussila2014b). In apparent disagreement with this general trend for elevational gradients, latitudinal trends of higher diversity in the tropics, even in the lowlands, seem to be consistently corroborated by recent publications (Veijalainen et al. Reference Veijalainen, Wahlberg, Broad, Erwin, Longino and Sääksjärvi2012, Reference Veijalainen, Sääksjärvi, Erwin, Gómez and Longino2013; Timms et al. Reference Timms, Schwarzfeld and Sääksjärvi2015; Shimizu et al. Reference Shimizu, Broad and Maeto2020), indicating that the anomalous pattern therewith observed and theorised for ichneumonids (Owen and Owen Reference Owen and Owen1974; Askew and Shaw Reference Askew, Shaw, Waage and Greathead1986; Gauld Reference Gauld1986, Reference Gauld1987) might be caused by biased data, especially for small-bodied koinobiont species that are harder to identify (Sääksjärvi et al. Reference Sääksjärvi, Haataja, Neuvonen, Gauld, Jussila, Salo and Burgos2004; Santos and Quicke Reference Santos and Quicke2011; Quicke Reference Quicke2012), such as the Orthocentrinae.
Conclusions
Here, we evaluated Rapoport’s rule by testing range sizes against elevation and observed a significant correlation supporting the rule, with species at higher elevations having larger ranges. Support for Rapoport’s rule, even for invertebrates, and using Stevens’s (Reference Stevens1992) method, which is less rigorous, was found in less than 41% of the literature (McCain and Knight Reference McCain and Knight2013). Although criticisms have been made of Rapoport’s rule and on the methods for its evaluation, the underlying mechanism seems to be valid and received good support recently (e.g., Chan et al. Reference Chan, Chen, Colwell, Liu, Huang and Shen2016), even when species ranges do not follow predictions due to complex climate gradients (Pintor et al. Reference Pintor, Schwarzkopf and Krockenberger2015). This and the fact that mountain passes are higher in the tropics (Janzen Reference Janzen1967; Sheldon et al. Reference Sheldon, Huey, Kaspari and Sanders2018) may be important factors that could lead to observations confirming larger elevational ranges at higher elevations in tropical mountains, as observed for braconids (Aguirre et al. Reference Aguirre, Shaw and Rodríguez-Jiménez2018). This is an extremely relevant consideration for conservation because tropical lowlands could harbour the highest diversity and concurrently suffer the greatest impacts.
The need for taxonomic studies on Neotropical Orthocentrinae, especially revisions of most genera, is indisputable. The taxonomic problems, aggravated by the fact that the subfamily has been considered a “trash basket” for many years, have prevented the advancement of biological and ecological knowledge and the subfamily’s application as biological control agents. In the present study, we presented the first illustrated key for Brazilian Orthocentrinae genera, including both genus groups (Orthocentrus and Helictes). By providing this taxonomic synopsis, we hope to facilitate future taxonomic reviews and to encourage research on this group.
Acknowledgements
The authors thank CNPq (Conselho Nacional de Desenvolvimento Científico e Tecnológico), CAPES (Coordenadoria de Aperfeiçoamento de Pessoal de Nível Superior), FAPESP (Fundação de Amparo à Pesquisa do Estado de São Paulo), and INCT-Hympar for financial support; the managements of the Reserva Privada do Patrimônio Cultural de Itamonte, Reserva Biológica da Serra do Japi, Parque Nacional da Serra da Bocaina, Parque Nacional do Itatiaia, Parque Estadual da Serra do Mar, and Airton Santos Soares and the collection team for the collections of material; Luciana Bueno dos Reis Fernandes for taking the images; and all the technicians from INCT-Hympar.