Introduction
It is estimated that about 80% of insects and 95% of mites are still unnamed. At the same time, it has been assessed that insect diversity is declining more rapidly than the diversity of vertebrates and plants (Behan-Pelletier & Newton, Reference Behan-Pelletier and Newton1999; Thomas et al., Reference Thomas, Telfer, Roy, Preston, Greenwood, Asher, Fox, Clarke and Lawton2004; Stork, Reference Stork2017). With insufficient knowledge, it is difficult to assess the changes in the diversity of arthropods, and most attempts involve large extrapolations from a limited number of well-studied taxa and habitats. In this context, undersampled regions or very specific and increasingly rare microhabitats need to be examined (e.g., Skelley & Kovarik, Reference Skelley and Kovarik2001). There is only one study devoted to arthropod assemblages inhabiting hamster burrows in the Netherlands (Evers, Reference Evers1940). The authors listed 60 species of beetles (Coleoptera L.), including very common species and several interesting records of rare species, such as Atomaria nigriventris Stephens J.F., 1830 (Cryptophagidae) or Otiorhynchus ligustici (L., 1758) (Curculionidae). Scattered data about the rove beetles occurring in the burrows of the common hamster (Cricetus cricetus L.) can also be found in Nowosad (Reference Nowosad1990), which was focused on Staphylinidae in the nests of Talpa europaea L. This insufficient knowledge has led us to undertake studies on arthropod assemblage compositions in the burrows of the common hamster.
The common hamster is a small rodent previously considered a pest and now classified as susceptible to becoming critically endangered in Europe (Nechay, Reference Nechay2000; Weinhold, Reference Weinhold2008). Its range, limited by climate conditions and the type of soil in which they build burrows, extends from the river Yenisei in Russia through central to western Europe (Korbut et al., Reference Korbut, Rusin and Banaszek2013; Feoktistova et al., Reference Feoktistova, Meschersky, Bogomolov, Sayan, Poplavskaya and Surov2017). It inhabits steppes (e.g., in East Siberia and Kazakhstan), various types of agricultural fields, vegetable gardens, and lawns in big cities where extra food resources can be found (Banaszek & Ziomek, Reference Banaszek and Ziomek2010; Surov et al., Reference Surov, Poplavskaya, Bogomolov, Kropotkina, Tovpinetz, Katzman and Feoktistova2016b). Nevertheless, over the last half-century, the population of this rodent has declined in all European states, and a massive reduction of its range has been noted at the westernmost range edge (Neumann et al., Reference Neumann, Jansman, Kayser, Maak and Gattermann2004; Weinhold, Reference Weinhold2008; Hegyeli et al., Reference Hegyeli, Kecskés, Korbut and Banaszek2015; Surov et al., Reference Surov, Banaszek, Bogomolov, Feoktistova and Monecke2016a). Additionally, in Poland, the common hamster has almost disappeared in much of the northern, western, and central regions and is preserved almost exclusively in the southeast parts of the country (Ziomek & Banaszek, Reference Ziomek and Banaszek2007; Melosik et al., Reference Melosik, Ziomek, Winnicka, Reiners, Banaszek, Mammen, Mammen and Marciszak2017). In Poland, this species is protected under the Regulation of the Minister of the Environment (Dz. U. December 28, 2016, pos. 2183). At this time, however, the structure and diversity of arthropods in their burrows remain poorly understood, especially in the context of parasitic species that may affect the health of the common hamster. Thus, our work may have some practical relevance to the management of the common hamster and its effective protection. Additionally, regarding the mass disappearance of the common hamster, this may be the last moment in which we can understand which arthropods inhabit its burrows.
The hamster burrow, a complex system of several chambers and corridors that can be more than a meter in length depending on the type of soil and the groundwater level, is a place to hibernate, raise young, store nutrient-rich food, and establish a latrine (Weinhold, Reference Weinhold2008). Because of the abundance of different kinds of food and relatively constant temperature conditions inside the burrow, as well as protection against predation, it may serve as a resting and feeding place for mating or oviposition for many arthropod groups. These arthropods, however, might be specific inhabitants (narrow-niched specialists) that are prone to extinction and subject to the influence of the changing environment and habitat fragmentation (Colles et al., Reference Colles, Liow and Prinzing2009; Clavel et al., Reference Clavel, Julliard and Devictor2011). By surveying such unusual microhabitats, it is possible to understand many aspects of the biology of arthropods and their ecosystem, as well as find species that are new to a particular area (Kovarik et al., Reference Kovarik, Chordas, Robison, Skelley, Connior, Fiene and Heidt2008). In the case of pathogens, surveying them may explain disease emergence in the host population (Woolhouse & Gowtage-Sequeria, Reference Woolhouse and Gowtage-Sequeria2005).
Variance in arthropod assemblages can vary according with environmental and spatial factors and trapping techniques (e.g., Peterson et al., Reference Peterson, Hendrix, Haydu, Graham and Quideau2001; Liu et al., Reference Liu, Li, Liu, Liu and Niu2012, Reference Liu, Zhu, Song, Yang and Chai2013; Lamarre et al., Reference Lamarre, Herault, Fine, Vede, Lupoli, Mesones and Baraloto2016; Skvarla & Dowling Reference Skvarla and Dowling2017). Evidence shows that the type of vegetation cover, species identity, the age of planted plants, and habitat types affect the distribution and diversity of arthropods. Also, based on coarse-scale studies, there is a general pattern of arthropod community change with geographic distance (Cleary & Genner Reference Cleary, Genner, Hawksworth and Bull2006; Gathmann et al., Reference Gathmann, Manne and Williams2009; Krasnov et al., Reference Krasnov, Mouillot, Shenbrot, Khokhlova, Vinarski, Korallo-Vinarskaya and Poulin2010). Additionally, the results of arthropod inventories depend on trapping techniques. Pitfall traps appeared to be one of the most effective for catching some groups of beetles (Skvarla & Dowling, Reference Skvarla and Dowling2017). Nevertheless, arthropod-composition research at a fine scale in the context of dominant vegetation adjacent to rodent burrows or geographic distances between sites has been rarely conducted, and therefore, the factors governing assembly rules are poorly understood.
The main purpose of our study was to characterize the species composition and diversity, including trophic structure, of arthropod assemblages living in burrows of the common hamster. Using the sampled data, we tested the following hypotheses: (1) arthropod assemblages are associated with a particular dominant vegetation occurring in the vicinity of the burrows; (2) a correlation exists between fine-scale geographic distances among burrows and arthropod assemblage dissimilarity; and (3) trap type influences the sampling success of captured arthropods.
Materials and methods
Study area
We focused our arthropod sampling on the cultivated area in Przybyszów village (50.62N 19.92W) in the southern part of Poland (fig. 1). This location was chosen because we knew, based on country-wide monitoring, that the common hamster inhabits the area. For the survey, we used a location sampling method, and we analyzed all of the burrows found that were considered active or possibly active and characterized by a specific depth (over 30 cm).

Fig. 1. Study area in southern Poland. The distribution of the investigated hamster burrows (dots) is shown.
Burrows were considered active based on visible traces of hamster activity in the immediate proximity of entrance holes, whereas the possibly active burrows were identified based on open entrances and the lack of fresh traces of activity. The specific depth (>30 cm) was chosen based on our field observations related to the depth at which the turn of the vertical corridor leading to the main chamber was present.
The sampled area (22 ha) comprises a mosaic of fields with different vegetation types. The principal crops are triticale (x Triticosecale), clover (Trifolium repens L.), wheat (Triticum sp.), and fodder peas (Lathyrus sp.). The cultivated fields were separated by narrow, slightly elevated belts of ruderal plants (e.g., Taraxacum officinale Wigg., Plantago sp., Urtica sp., Achillea sp., Polygonum sp., Vicia sp., Capsella sp., Centaurea sp., Bellis sp., Matricaria sp., Chenopodium sp., and Senecio sp.) that formed a natural fence (otherwise known as balks) between narrow patches of cultivated fields. A residential area surrounded the fields. The cultivation areas, as well as the distribution of the dominant vegetation, are changed from year to year. The arthropods, identified by the authors to the species level or a higher taxonomic level, were collected from 109 burrows in total. The burrows were separated by at least 7 m (fig. 1).
Arthropod trap design
To capture the arthropods, we modified a live trap designed by Szujecki (Reference Szujecki1980) (see Celebias, Reference Celebias2017; Celebias et al., Reference Celebias, Przewoźny, Gwiazdowicz and Ziomek2017). We tried various container designs, but, eventually, we made a cube-shaped container (40 mm × 40 mm) using wire (Fig. S1). One wall of the container can be opened. Because the common hamster is an omnivorous species that eats both plants and small animals (invertebrates and small vertebrates), we decided to use traps baited with meat. The trap container was filled with dry grasses and was baited by approximately 3–5 g of meat or was non-baited (control). The mesh size (2 × 2 mm) prevented bait dropping; however, several randomly distributed holes of ca. 4 × 4 mm on the walls of the trap allowed arthropods of larger dimensions to enter the trap. A metal loop installed in the container allowed the placement and subsequent removal of the trap from the burrow. The box and the loop form a total length of 50 cm. We did not use any solutions designed to kill and preserve the trapped arthropods (e.g., ethylene glycol or methylated spirits), and we positioned the traps at a depth that was no <30 cm (in the turn of the vertical corridor leading to the main chamber) and in a burrow with more than one entrance. Additional burrow entrances allowed the hamsters to leave or enter the burrow when the trap was placed. After 3 days, the traps were retrieved, and the arthropods were segregated and stored in 70–96% ethyl alcohol. In the laboratory, all the specimens were classified to the species or higher taxonomic levels. The specimens are preserved in the Department of Systematic Zoology at Adam Mickiewicz University in Poznań and in the Department of Forest Pathology at Poznań University of Life Sciences, Poland. We collected the arthropods in the early and late summer (June and August) over 2 years (2015–2016).
Data analysis
To evaluate our sampling effort, we used a species accumulation curve to predict the species richness and estimate the minimum effort required for satisfactory completion of the survey. We also used a CHAO1 estimator based on the species abundance distribution (SAD) (Chao, Reference Chao1984; Gotelli & Colwell, Reference Gotelli and Colwell2011) and calculated the extrapolation curve and the 95% lower and upper boundaries of confidence intervals with 100 randomized runs. To calculate the degree of sample completeness (Chao & Chiu, Reference Chao, Chiu, Balakrishnan, Colton, Everitt, Piegorsch, Ruggeri and Teugels2016), we evaluated the abundance-based coverage estimator (ACE) of species richness (i.e., the number of species present in the study area), for the baited and non-baited (control) traps. To estimate the number of species in common for sample pairs, we computed a Chao shared species estimator (Chao et al., Reference Chao, Hwang, Chen and Kuo2000). We calculated all of these parameters using the program EstimateS 9.1.0 (Colwell et al., Reference Colwell, Chao, Gotelli, Lin, Mao, Chazdon and Longino2012).
To assess arthropod assemblage properties, we evaluated the SAD (i.e., the pattern of commonness and rarity among species) (McGill et al., Reference McGill, Etienne, Gray, Alonso, Anderson, Benecha, Dornelas, Enquist, Green, He, Hurlbert, Magurran, Marquet, Maurer, Ostling, Soykan, Ugland and White2007; Volkov et al., Reference Volkov, Banavar, Hubbell and Maritan2007) as implemented in the sads package in R (Prado et al., Reference Prado, Miranda and Chalom2016). The procedure is composed of several steps broken into two phases: an explanatory analysis and model fitting. The explanatory analysis includes a tabulation of the number of species into classes of abundances followed by the sorting of abundances and their ranks. The model fitting allowed us to test models representing different families, as implemented in the sads package, for describing the SAD. We used maximum likelihood estimation for fitting distributions to data and evaluating their fit. We employed the Akaike information criterion (AIC) to compare the fit of the models (Matthews & Whittaker, Reference Matthews and Whittaker2014; for more details see McGill et al., Reference McGill, Etienne, Gray, Alonso, Anderson, Benecha, Dornelas, Enquist, Green, He, Hurlbert, Magurran, Marquet, Maurer, Ostling, Soykan, Ugland and White2007).
To quantify the diversity patterns of arthropods, we calculated the inverted Simpson's diversity index (1/D) with vegan 2.4–3 in R (Oksanen et al., Reference Oksanen, Kindt, Legendre, O'Hara, Stevens, Oksanen and Suggests2007), http://cc.oulu.fi/~jarioksa/softhelp/vegan/html/diversity.html. The Simpson's diversity index is based on D = ∑ p i2, where p i is the proportional abundance of species i. The inverted Simpson's diversity index, used in this study, is linearly related to the exponent of the Shannon index (Hill, Reference Hill1973).
We assumed the explored agroecosystems (defined as crop habitats and non-crop habitats adjacent to the crops [You et al., Reference You, Hou, Liu, Yang, Li and Cai2004]), a mosaic of patches characterized by different dominant vegetation types, affect arthropods species diversity and abundance. We defined the dominant type of vegetation in the vicinity of burrows based on field observations and aerial photographs. The dominant vegetation was identified within the radius of a minimum of 2 m from the burrow assuming 90–100% coverage of the area by the dominant species. The following groups of dominant vegetation were defined: (1) clover, (2) fodder peas, (3) wheat, (4) triticale, and (5) wild grasses.
To contrast the strongest link of a species to a particular resource (neighboring vegetation) with the other types of vegetation, we calculated the abundance-weighted paired difference index (PDI) using the package bipartite 3.2.5 in the R environment (Poisot et al., Reference Poisot, Lepennetier, Martinez, Ramsayer and Hochberg2010, Reference Poisot, Bever, Nemri, Thrall and Hochberg2011, Reference Poisot, Canard, Mouquet and Hochberg2012). We started with the null hypothesis that the investigated agricultural fields are uniform and took into account the arthropod species composition. In this case, faunistic dissimilarity should have no identifiable pattern. In particular, the degree of faunistic dissimilarity should not depend on the geographic distance between the analyzed burrows. To calculate the dissimilarity of arthropod species composition between the investigated burrows, we calculated the Jaccard index, which is the most commonly used index based on the presence/absence of data (Jaccard, Reference Jaccard1912) (https://sites.google.com/site/fredsoftwares/products). We also plotted the values of the index against the geographic distances between burrows using a Mantel test, as implemented in GenAlEx 6.5 (Peakall & Smouse, Reference Peakall and Smouse2006). We considered species assemblages in burrows where three of the most abundant species occurred. We used a non-parametric Kruskal–Wallis test and a Mann–Whitney U test (adjusted for ties), as implemented in STATISTICA (StatSoft), to check for significant differences in arthropod assemblages among different types of vegetation, between baited and unbaited traps, and cultivated fields and balks (the data obtained did not follow a normal distribution).
Results
Abundance, richness, and diversity
The extrapolation of the accumulation curve shows there is no saturation regarding the number of arthropod species collected from the investigated area. The calculation of the Chao1 estimator demonstrates that we should expect to find 106 species of arthropods (95% confidence interval 76.56–135.9 species) (fig. 2). We estimated that a mean of 535 burrows would need to be surveyed to identify all of the potential species. To reach the 95% lower boundary (76.56 species), an additional 12 burrows and 50 arthropod individuals (at minimum) need to be found and identified.

Fig. 2. Extrapolation of a sample-based rarefaction curve of the arthropod assemblages detected in hamster burrows [S(est)] calculated with EstimateS. The mean number of burrows that should be examined to detect all arthropod species inhabiting the studied area is indicated by a dot (535 burrows), and the number of investigated burrows and identified species in this study were set up for zero point.
Out of the 508 specimens collected, we identified 398 individuals to species and 110 specimens at higher taxonomic levels (families, orders, or genera). In 103 burrows, we identified 73 species of arthropods belonging to 16 families (fig. 3 and table 1) and three orders: the most abundantly represented were Coleoptera (74.12% individuals), Acari (23.37%), and Araneae (2.51%). The specimens that were identified to the family level represented the families already named for the identified species and a new family – Liocranidae. The specimens identified to the order level represented nine orders: Hymenoptera (30%), Araneae (23.64%), Diptera (21.82%), Collembola (15.45%), Acari (3.64%), Isopoda (2.73%), and the sporadically occurring Thysanoptera, Coleoptera, and Diplura (0.91% each). The specimens identified to the genera level represented Clubiona, Cryptophagus, Gnaphosa, Oedothorax, Pardosa, Trochosa, Hypoaspis, Phrurolithus, and Haldaelaps.

Fig. 3. Family richness and abundance for arthropods collected in the hamster burrows.
Table 1. Arthropod species assemblage detected in the hamster burrows.

The values of the paired difference index (PDI): (1) between accessions captured with meat-baited and control traps; (2) between burrows situated on cultivated fields and balks; (3) among burrows localized in different dominant vegetation; (4) inverted Simpson's diversity index (invSimpson index) (1/D); (5) D (%) = n/N × 100, where n is the number of individuals of a given species present in the collected samples, and N is the total number of collected individuals; (6) I A absolute infestation intensity (per all burrows); nomenclature: Coleoptera (Löbl & Smetana, Reference Löbl and Smetana2003, Reference Löbl and Smetana2007; Löbl & Löbl, Reference Löbl and Löbl2015) and mites: (Hyatt, Reference Hyatt1980; Mašán, Reference Mašán2003).
The arthropod species richness and abundance differed among families (fig. 3). Staphylinidae (41 species) and Parasitidae (seven species) were the richest, whereas the remaining 14 families were represented by only one to three species. The following families were the most abundant: Staphylinidae, Cryptophagidae, Parasitidae, and Macrochelidae (78.89% of individuals). The individuals belonging to Leiodidae, Histeridae, Carabidae, and Silphidae were less represented (10.80%), whereas the least numerous arthropods belonged to Linyphiidae, Ascidae, Ptiliidae, Lycosidae, Clubionidae, Gnaphosidae, Hydrophilidae, and Haemogamasidae (10.31%).
A histogram of the number of species vs abundance (see Fig. S2) produces a typical J-curve showing a general pattern, with many rare species and only a few abundant species. Our comparison of different models for the SAD, performed across all species and sites, demonstrated that the purely statistical Poisson-log normal distribution model (McGill, Reference McGill2003; McGill et al., Reference McGill, Etienne, Gray, Alonso, Anderson, Benecha, Dornelas, Enquist, Green, He, Hurlbert, Magurran, Marquet, Maurer, Ostling, Soykan, Ugland and White2007) had the highest AIC weight compared with the other models considered (see Fig. S2). Three species, Macrocheles matrius (Macrochelidae), Cryptophagus schmidti (Cryptophagidae), and Pycnota paradoxa (Staphylinidae) were particularly abundant (inhabiting 31.86–60.18% of burrows). In contrast, in the latter family, we found species that occurred only once in the analyzed burrows (24 singletons), for example, Aleochara irmgardis, Amischa analis, Atheta vilis, Cercyon analis, Drusilla canaliculata, Lathrobium fulvipenne, Oxypoda opaca, Philonthus addendus, Quedius invreae, Sepedophilus transcaspicus, Stenus palustris, Tachinus corticinus, and Xantholinus elegans. Drassodes lapidosus and Haplodrassus silvestris represented rarely occurring species belonging to Gnaphosidae. Species that were found only once included: Eulaelaps stabularis (Haemogamasidae), Poecilochirus sexclavatus (Parasitidae), and Pardosa palustris and Trochosa robusta (Lycosidae).
The richness and abundance differ between June and August. In August, we captured 42.86% more species and 61.96% individuals than in June (63 and 36 species and 368 and 140 individuals, respectively). The mean value of the Shannon diversity index for the investigated burrows was equal to 3.10. In pairwise comparisons of burrows, the mean number of species shared between sample pairs appeared to be low (0.95).
Is habitat type in the vicinity of burrows a good predictor of arthropod community composition?
There was a strong positive correlation between the number of arthropod species detected in burrows, situated in a particular vegetation type, and the number of burrows investigated (r = 0.88). The triticale habitat was species-rich (51 out of 73 species identified) and the most abundant (64.07%) compared with the other habitats, probably because of the preference of hamsters to settle in this type of habitat (71 burrows investigated) (figs 4a, b and 5). Additionally, 39 arthropod species inhabited 21 burrows located in clover (Trifolium sp.). However, we found a small number of burrows in fodder peas and wild grasses, resulting in a small number of arthropod species discovered (nine and four species, respectively). Therefore, our statistical testing may be biased because of the small sample size in some cases (fig. 5).

Fig. 4. Number (a) and abundance (b) of arthropod species in burrows located in different types of habitat.

Fig. 5. Mean diversity (inverted Simpson's diversity index [1/D]) of arthropods per burrow of the common hamster ± standard error (SE) in each habitat type (see Materials and methods). (a) Arthropods living in burrows situated within patches of different dominant vegetation. (b) Arthropods caught in traps baited and arthropods caught in the non-baited traps. (c) Arthropods living in burrows located on cultivated fields and balks. *Statistical significance at *P < 0.05 and ***P < 0.001.
We considered habitat specialization or rarity in the context of the number of habitats from which we sampled a species. Thus, a species found exclusively in one habitat defined by the dominant vegetation in which the burrows were located was considered a habitat specialist or rare species, whereas a species occurring in at least two habitats was defined as a habitat generalist. Based on PDI index values, the majority of species appeared to be specialists or rare species (PDI = 1) and were only from the burrows found in one type of vegetation (61.64%). We collected 51 species (64.07% of all individuals sampled) in the burrows located in triticale, 39 species (22.87% of individuals) in clover, 24 species (9.27% of individuals) in wheat, nine species in fodder peas, and four species in wild grasses (2.77 and 1.02% of individuals, respectively) (fig. 4a). As many as 27 species out of 73 (36.99%) were found exclusively in burrows situated in the triticale habitat, whereas 13 species (17.81%) were exclusively found in clover, but only four species (5.48%) were found in wheat, and two species (2.74%) were exclusively found in fodder peas. The other insect species (36.98%), considered habitat generalists, occurred at the burrows located in different crops. Habitat generalists (21.92%) occurred in equal frequency in both kinds of traps (PDI < 1), (table 1). Farmland appeared to be a more favorable environment for arthropods than the barren balks. Additionally, 27 species out of 73 (36.99%) were exclusively found in the burrows situated on the cultivated fields, and less than half (16.44% of the cases) in burrows occurring on the balks. The remaining species were common in their occurrence in both types of habitats (46.57%).
Is geographic distance between burrows associated with dissimilarity in arthropod assemblages?
When comparing arthropod biodiversity, expressed by the Jaccard index values for the assemblages in which the most common inhabitants occurred, i.e., C. schmidti, M. matrius, and P. paradoxa (figs 6a–6c), and geographic distances among the burrows we found that there was no significant correlation between these values (R xy = −0.013, P = 0.447, test R xy = 0.037, P = 0.283, and R xy = −0.543, P = 0.684, respectively).
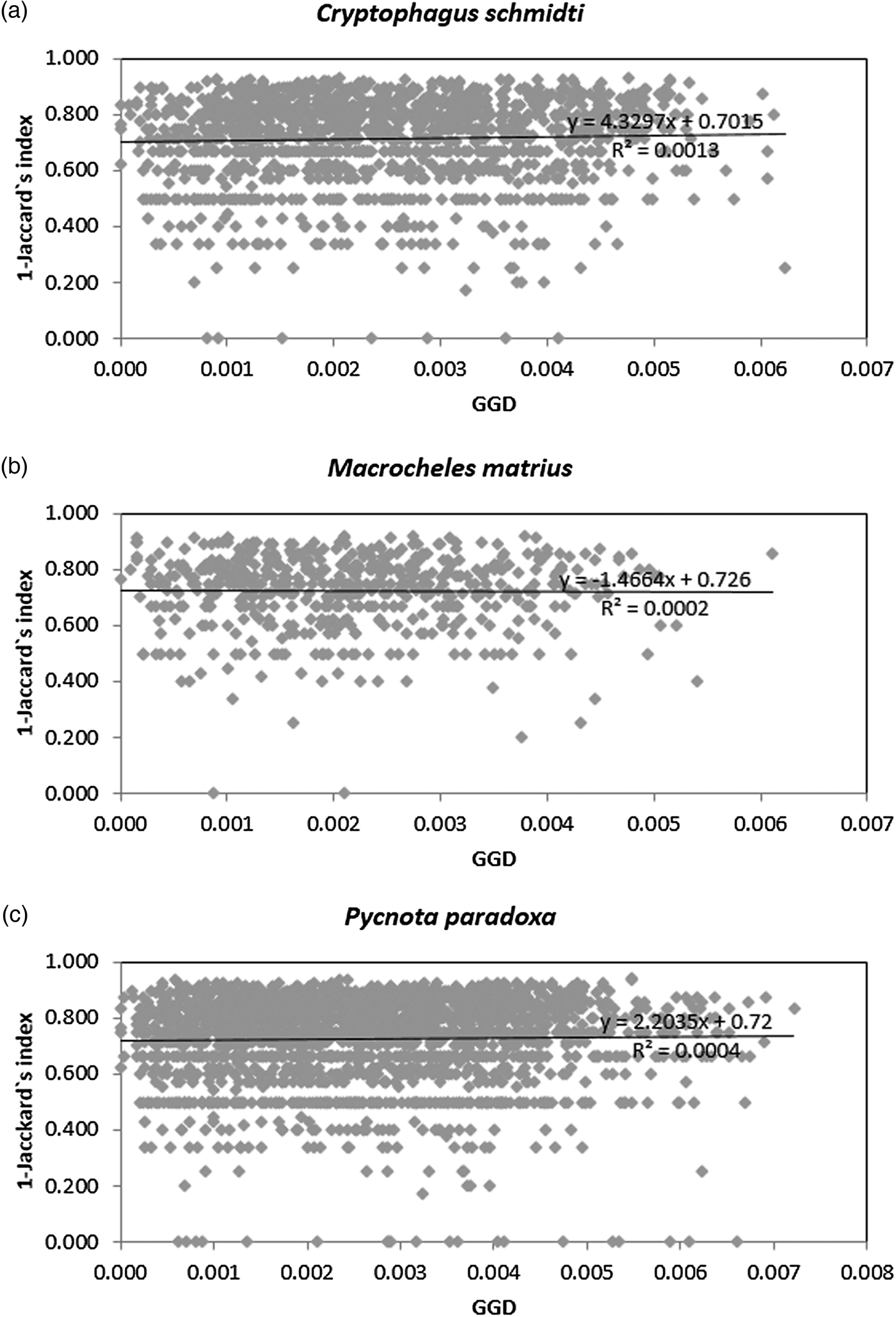
Fig. 6. Between-insect sample set dissimilarity, as revealed by the Jaccard method, plotted against the geographic distances between burrows, as measured with the most abundant species Cryptophagus schmidti (a), Macrocheles matrius (b), and Pycnota paradoxa (c).
Does the bait used influence the sampling success of captured arthropods?
Arthropod samples originating from the baited and non-baited traps had different degrees of completeness based on the ACE (fig. 7). For the baited traps, the ACE estimator increased logarithmically, reaching a horizontal asymptote at 44 burrows, whereas for the control (non-baited) traps, the ACE showed the sample was incomplete. In other words, for the control traps, the sample covered only 36% of the population.

Fig. 7. Abundance-based coverage estimator curves for baited and non-baited traps. The x-axis is re-scaled to the percentage of the sampling effort, and 100% is the maximum value of the larger sample (baited traps).
The traps baited with meat caught a greater variety of arthropods species, which represented different trophic groups, and in a greater abundance than the control traps. Zoophagous species were dominant in both kinds of traps. In the baited traps, we found more saprophages, coprophages, and necrophages (fig. 8).

Fig. 8. Number of arthropod individuals belonging to different trophic groups caught using baited and control traps. Trophic groups were defined for 84.93% of the identified species.
The traps baited with meat caught 71 species (82.16% of individuals identified to the species level). We found mainly Staphylinidae (39 species, 54.93%) and Parasitidae (seven species, 9.86%), as well as one to three species represented by the other 14 families. In the non-baited traps, we observed the same pattern (Staphylinidae and Parasitidae were most strongly represented, 33.33 and 22.22%, respectively), but we caught fewer species (18 species, 17.83%). Only one or two species were from the other five families (Leiodidae, Cryptophagidae, Histeridae, Carabidae, and Macrochelidae). Two species (A. vilis and X. elegans, 2.74%) occurred exclusively in the control traps.
Discussion
In this study, conducted in southern Poland, we evaluated the taxonomic composition and trophic structure of arthropod species in the burrows of the common hamster (C. cricetus). This assessment is important because it is an unstudied subject, a decline in the range of the hamster, and subsequent need for conservation efforts (Nechay, Reference Nechay2000; Ziomek & Banaszek, Reference Ziomek and Banaszek2007; Weinhold, Reference Weinhold2008; Hegyeli et al., Reference Hegyeli, Kecskés, Korbut and Banaszek2015; Surov et al., Reference Surov, Banaszek, Bogomolov, Feoktistova and Monecke2016a). We assessed diversity of arthropods in relation to methods used to collect them as well as the role of the local environment and geographic distances among burrows. We also provided information on parasitic organisms that may have an impact on the health of the common hamster.
Characterization of the arthropod community
Every arthropod assemblage differed in composition from the others, but as in the burrows of other small mammals (Šustek & Stanko, Reference Šustek and Stanko2012), the core species found in burrows of common hamsters were shared. Staphylinidae and Parasitidae dominated the total assemblage (fig. 3), and these groups also dominate arthropod assemblages in the nests of other mammals and birds (Soliman et al., Reference Soliman, Zaher and Mohamed1978; Fend'a, Reference Fend'a, Sabelis and Bruin2010; Šustek & Stanko, Reference Šustek and Stanko2012; Krawczyk et al., Reference Krawczyk, Augustiniĉová, Gwiazdowicz, Konwerski, Kucharczyk, Olejniczak, Rutkowski, Skubała, Solarz, Zdrojewska and Tryjanowski2015). The number of species found in the burrows of common hamsters (table 1) was lower than found in the above-ground nests of Micromys minutus (Pallas, 1771) (105 species/44 nests, Krawczyk et al., Reference Krawczyk, Augustiniĉová, Gwiazdowicz, Konwerski, Kucharczyk, Olejniczak, Rutkowski, Skubała, Solarz, Zdrojewska and Tryjanowski2015), but a direct comparison with other surveys is difficult because of the different criteria employed. We detected a high representation of saprophagous, coprophagous, and necrophages species. Following a general division of the trophic groups of mites characteristic for the nests of small mammals (Mašán & Stanko, Reference Mašán and Stanko2005, see also Gwiazdowicz, Reference Gwiazdowicz2007), we found predominantly non-parasitic mites (Acari, Mesostigmata), representing four major groups [i.e., (1) obligatory or facultative nidicolous, free-living mite species having trophic relations to the burrows, (2) edaphic species without any trophic or topic relation to their host, (3) coprophilous species, and (4) a single parasite species].
Although the investigated burrows were similar in some respects, there were also differences in abundance and composition. Out of all arthropod species observed in the study area, the most abundant were P. paradoxa (Staphylinidae) and Cryptophagus schmidti (Cryptophagidae). In Poland, both species were reported as sparsely occurring, and the former was unobserved for decades (Burakowski et al., Reference Burakowski, Mroczkowski and Stefańska1981). We also reported other species from Staphylinidae rarely occurring in Poland, but these were less numerous in the investigated burrows, such as Aleochara kamila, Anotylus rugifrons, Atheta aeneicollis, A. vilis, Q. invreae, S. transcaspicus, S. palustris, X. elegans, and X. gallicus. Nevertheless, it is difficult to distinguish between an authentically rare species and those that have rarely been collected, with presently unknown or incomplete distribution. Some species, which are rare or commonly occur in Poland, were already collected from the nests of mammals, birds or insects, but not recorded from hamster burrows; thus, our data represent a new record for this microhabitat [e.g., Sciodrepoides watsoni or Sunius melanocephalus (Staphylinidae) (Mašán & Stanko, Reference Mašán and Stanko2005; Šustek & Krištofík, Reference Šustek and Krištofík2009)]. We also discovered an arthropod species new to Poland and significantly extended the known range for the species [i.e., A. irmgardis (Staphylinidae) and P. sexclavatus (Parasitidae)]. The latter was detected on carriers, such as Phosphuga atrata L., Hister uncinatus Illiger, 1807, and Nicrophorus vespillo, in Ukraine (Skljar, Reference Skljar2002), whereas A. irmgardis was found in nests of a mole in other parts of Europe (Schatz, Reference Schatz2009).
The surprising result of this study is that we found only one parasitic species in the hamster burrows (E. stabularis). Parasitic mites were abundantly represented in nests of Mus spicilegus (Stanko et al., Reference Stanko, Čanády, Fričová, Mošanskỳ, Čisláková, Karbowiak, Prigioni and Sforzi2007) and other small rodents (Büchner et al., Reference Büchner, Stubbe and Striese2003; Kaminskienė et al., Reference Kaminskienė, Radzijevskaja, Balčiauskas, Gedminas and Paulauskas2017). Nevertheless, our result is somewhat consistent with the study of Bright and Morris (Reference Bright and Morris1996). The rarity of parasitic species may be explained by the variation in nest characteristics across taxa (Athias-Binche, Reference Athias-Binche and Rodriguez1979; Krištofìk et al., Reference Krištofìk, Masan, Sustek and Gajdos1993), the result of declining host abundance (Stanko et al., Reference Stanko, Krasnov and Morand2006), difficulties surrounding species identification, limited sampling, or the local character of this study. In the context of hamster rarity, additional studies should be conducted on the relationship between parasite abundance and common hamster abundance and how the parasite and host abundance is regulated (e.g., parasite- vs. host-induced mortality, interactions among parasites) (Stanko et al., Reference Stanko, Krasnov and Morand2006).
Is habitat type in the vicinity of burrows a good predictor of arthropod community composition?
We found an effect of fine-scale landscape heterogeneity, regarding dominant vegetation in the vicinity of burrows, on arthropod abundance and richness. However, undersampling bias strongly influenced our biodiversity assessment. The effect of undersampling at some sites and oversampling at others is difficult to overcome because of the rarity of common hamsters in Poland and their preference for particular habitats. Further research is necessary that takes into account factors such as balanced sampling in areas where the common hamster is more common, the chemical impact of plant protection products on a particular vegetation, or biophysical characteristics of the under- and above-ground parts of plants to conclude a causal association between arthropod diversity and the dominant vegetation adjacent to the burrows.
Is geographic distance between burrows associated with dissimilarity in arthropod assemblages?
While some emphasis has been placed on describing the effect of geography on arthropod diversity at a regional scale (e.g., Krasnov et al., Reference Krasnov, Shenbrot, Mouillot, Khokhlova and Poulin2005), few studies so far have investigated a fine-scale geographic distance effect on arthropod diversity, species richness, or abundance (Krasnov et al., Reference Krasnov, Mouillot, Shenbrot, Khokhlova, Vinarski, Korallo-Vinarskaya and Poulin2010; Dominik et al., Reference Dominik, Seppelt, Horgan, Marquez, Settele and Václavik2017). Consistent with these studies, we found no significant correlation between geographic distances between sites and dissimilarity in the arthropod assemblages. The mechanism underlying this pattern may be the general external and internal environmental similarity and the lack of hard geographic barriers blocking the dispersal of the arthropods and the host. Factors such as diverse climatic conditions, elevation or landscape structure at a broader scale might be more important than fine-scale differences in distance among burrows (see also Dominik et al., Reference Dominik, Seppelt, Horgan, Marquez, Settele and Václavik2017).
Does the bait used influence the sampling success of the captured arthropods?
The arthropod taxonomic and trophic composition were affected by the sampling method used. In our work, we found that baited traps caught a greater variety of arthropods than unbaited ones. In the latter case, the species accumulation curve did not reach an asymptote, which suggests that the sampling effort was insufficient. Generally, the meat-baited traps were more efficient, particularly at capturing zoophages, saprophages, coprophages, and necrophages, in comparision with the unbaited traps (fig. 8).
Each sampling method is somewhat biased because it is selective for a specific arthropod group, species, or developmental stage (Penny & Arias, Reference Penny and Arias1982; Nentwig, Reference Nentwig1989). A good example of method selectivity is P. paradoxa, a rare species known only from Upper Silesia and Lublin Upland (Poland). This species was captured abundantly using baited traps in the hamster burrows, as well as nests of the speckled ground squirrel (Spermophilus suslicus Guld.) (Melke & Staniec, Reference Melke and Staniec1999). Based on the observed differences between baited and unbaited traps, multiple methods should be used concurrently to provide complementary information. For example, to capture more diverse arthropod assemblages of coprophilous and necrophagous arthropods, we recommend, for future studies, the simultaneous use of meat-baited traps and dung-baited traps at each site. Moreover, traps should also be situated on the surface to differentiate between arthropods resident in the burrow or accidentally lured by traps. An investigation should be performed from spring to autumn (see also Doblas-Miranda et al., Reference Doblas-Miranda, Sánchez-Piñero and González-Megías2007) because, across the season, the diversity indices of the arthropods tended to increase.
Study limitations
An evaluation of how many species potentially occur in the study area is an important issue because the estimation can then be used in the assessment of different sampling methods or inventories, inferences about species change over time in the context of climate warming or other disturbances, as well as in planning sampling effort (and costs) (Colwell & Coddington, Reference Colwell and Coddington1994; Moreno & Halffter, Reference Moreno and Halffter2001; Willott, Reference Willott2001; Gardner et al., Reference Gardner, Barlow, Araujo, Ávila-Pires, Bonaldo, Costa, Esposito, Ferreira, Hawes, Hernandez, Hoogmoed, Leite, Lo-Man-Hung, Malcolm, Martins, Mestre, Miranda-Santos, Overal, Parry, Peters, Ribeiro-Junior, Da Silva, Motta and Peres2008). Although our study is the first extensive investigation, and it may provide the basis for further studies, we were only able to detect and identify 69% of the potential species in the area. Moreover, the number of species is probably much higher because only six species were common for a Netherlands arthropod inventory (Evers, Reference Evers1940) and this study. Besides, there were 23 species of Staphylinidae in hamster burrows recorded in 1919–1928, and in more recent times (see Nowosad, Reference Nowosad1990), but only five such species were confirmed by our research. Additionally, in the investigated burrows, non-parasitic mites representing the suborder Uropodina were absent but found, for example, in nests of T. europaea (Napierała & Błoszyk, Reference Napierała and Błoszyk2013; Napierała et al., Reference Napierała, Mądra, Leszczyńska-Deja, Gwiazdowicz, Gołdyn and Błoszyk2016). Given the sampling limitations because of host rarity, improved estimates of arthropod species composition and diversity may be obtained from the allocation of sampling effort in areas where the common hamster is more abundant (i.e., in the eastern part of its range) using one of the sampling methods for rare populations.
Conclusions
In this study, we present the first extensive survey of the arthropod assemblages occurring in hamster burrows, with records of two new species for Poland. The effect of the methods used on the taxonomic composition of taxa identified and the trophic structure are considered. The species richness and abundance do not contrast across geography on a small spatial scale. The hamster habitat preferences may explain the differences in richness and abundance of arthropod assemblages across habitats, as defined by the dominant vegetation. Based on our results and the previously published study, we can conclude that the total species number is much higher than presently known. Although the captured arthropods were not specific to the host, as is the case with other small mammals, they contribute to the ecosystem biodiversity. Additional study will be necessary to examine the consistency of patterns we observed by utilizing large-scale sampling across varied habitats throughout the geographic range of the common hamster.
Supplementary material
The supplementary material for this article can be found at https://doi.org/10.1017/S0007485319000087.
Acknowledgements
We thank Aleksandra Pieczewska for a spider identification (order Araneae). We thank the editor Professor Chris Weldon and two anonymous reviewers for their helpful comments and suggestions. All these comments greatly contributed to improving the final version of the paper.
Author contributions
J. Ziomek conceived the experiment and prepared data; P. Celebias prepared the equipment necessary for this study, collected arthropods, identified species (Silphidae), sorted arthropods to families, and prepared data; A. Melke identified Staphylinidae; D. J. Gwiazdowicz identified Acari and prepared data; M. Przewoźny identified arthropods from Carabidae, Histeridae, Hydrophilidae, and Leiodidae; E. Baraniak inspired the design of the trap and prepared data; K. Komosiński identified Cryptophagidae and Ptiliidae; K. Winnicka helped with the data analysis; and I. Melosik designed and carried out the data analysis and wrote the paper. All the authors read and approved the final version submitted.
Conflicts of interest
The authors declare that they have no conflict of interest.