Introduction
A controversial issue in pest regulation is whether maintaining natural plant biodiversity within agroecosystems has useful or undesirable implications for pest population size (Risch et al., Reference Risch, Andow and Altieri1983; Way and Heong, Reference Way and Heong1994). Some studies show that the presence of high natural plant diversity does not reduce the incidence of pests (Ratnadass et al., Reference Ratnadass, Fernandes, Avelino and Habib2012). However, other studies suggest that high natural plant biodiversity can positively contribute to agroecosystem productivity by enhancing natural pest biocontrol, because native vegetation can host natural enemies of crop pests which may assist natural regulation of pest outbreaks (Tscharntke et al., Reference Tscharntke, Bommarco, Clough, Crist, Kleijn, Rand, Tylianakis, van Nouhuys and Vidal2007; Haddad et al., Reference Haddad, Crutsinger, Gross, Haarstad and Tilman2011). Because of this role, many recent studies have concluded that conserving natural plant biodiversity around agricultural systems plays a positive function in helping to maintain crop health (e.g., Garbach et al., Reference Garbach, Milder, Montenegro, Karp and DeClerck2014; Kumalasari and Bergmeier, Reference Kumalasari and Bergmeier2014; Luo et al., Reference Luo, Fu and Traore2014).
However, as modern technologies are incorporated into agricultural systems, biological information such as this is increasingly ignored or dismissed, thereby contributing to the ecological instability of agroecosystems (Altieri and Nicholls, Reference Altieri and Nicholls2009). In large-scale agricultural practices, greater application of pesticides is required as cultivated zones expand, while areas of native vegetation decrease, hence reducing biodiversity and speeding up landscape simplification (Luo et al., Reference Luo, Fu and Traore2014). The combination of these things generates undesirable impacts both on people and on the environment (Guillette and Iguchi, Reference Guillette and Iguchi2012; Sández, Reference Sández2016), as well as increasing the incidence of pest species resistant to pesticides (Altieri and Nicholls, Reference Altieri and Nicholls2009).
In the Neotropical region, rice is an annual crop which can be produced in dry or irrigated systems. Unlike in Asia, where in most fields the rice seedlings are manually transplanted into irrigated plots (Arihara, Reference Arihara2013), in the Neotropics, the seeds are sown directly into the non-irrigated field plots with fertilized soil. Only when the plants enter the tillering stage does the irrigation phase begin, normally using water from natural sources in the rice production area (Rolon et al., Reference Rolon, Godoy and Maltchik2017). Because of this water use, irrigated rice agroecosystems, in contrast to terrestrial ones, are temporary artificial wetlands that not only provide nourishment but can also offer important ecosystem services for productive regions (Garbach et al., Reference Garbach, Milder, Montenegro, Karp and DeClerck2014; Berg et al., Reference Berg, Söderholm, Söderström and Tam2017). For this reason, it is necessary to develop, for large-scale producers, more profitable and sustainable alternatives for pest management in rice, reducing the ecological impacts generated by this crop (Hong-xing et al., Reference Hong-xing, Ya-jun, Yan-hui, Xu-song, Jun-ce, Feng-xiang, Qiang and Zhong-xian2017).
The rice stink bug Tibraca limbativentris Stål, 1860 (Hemiptera: Pentatomidae) is one of the most important and destructive rice pests in the Neotropics, mainly in Argentina (Ferreira et al., Reference Ferreira, Zimmermann, dos Santos and eves1997; Schaefer and Panizzi, Reference Schaefer and Panizzi2000; Kruger and Burdyn, Reference Kruger and Burdyn2015). This pest has been expanding its range, having been reported in Central America (Meneses, Reference Meneses2008) and appears to be approaching the southeastern USA (Hodges and Morse, Reference Hodges and Morse2007), with Florida's rice paddies potentially open to attack (Panizzi, Reference Panizzi2015). Tibraca limbativentris attacks rice plants in both the vegetative and reproductive stages (Pantoja et al., Reference Pantoja, Triana, Bastidas, García, Mejia and Duque2007; Kruger and Burdyn, Reference Kruger and Burdyn2015). The damage it causes to the crop has been considered similar to that caused to Asian rice crops by the pentatomids Scotinophara and Lagynotomus (McPherson, Reference McPherson2018). During the feeding process, this insect kills the stem by phloem sap-feeding, causing serious damage that results in crop diseases known as ‘dead heart’ and ‘white panicle’ which cause large losses in productivity (Ferreira et al., Reference Ferreira, Zimmermann, dos Santos and eves1997; Meneses, Reference Meneses2008). Annual losses from this insect of up to 65.2 kg hectare−1 have been recorded in irrigated rice crops (Costa and Link, Reference Costa and Link1992).
At the end of the rice growing season, when only stubble remains in the fields, and with progressive reduction of photoperiod, T. limbativentris populations enter diapause and take refuge in rice stubble and in natural vegetation around the fields (Link et al., Reference Link, Naibo and Pelentir1996; Kruger, Reference Kruger2014). These native plants serve as alternative hosts for T. limbativentris and allow it to survive low winter temperatures, helping the population to persist within rice fields and re-colonize the crop the following year (Kruger and Burdyn, Reference Kruger and Burdyn2015). This could imply that native vegetation and rice stubble both contribute to the maintenance of populations of this pest in rice agroecosystems by providing shelters to overwintering (‘bottom-up’ effect). However, there is limited information about what the alternative host plants of T. limbativentris may be when rice is absent, the abundance that its populations can reach in these plants, and whether this pest in winter hosts can be regulated by ‘top-down’ mechanisms through its natural enemies. This information is important for rice producers in the Neotropics, where this insect is native, because it can help understand the role of native vegetation within the agroecosystem.
Although there are previous reports of host plants for T. limbativentris in Brazilian rice agroecosystems (Aner and Becker, Reference Aner and Becker1993; Link et al., Reference Link, Naibo and Pelentir1996; Klein et al., Reference Klein, Rodrigues Redaelli, Barcellos, Klein, Redaelli and Barcellos2012; Botta et al., Reference Botta, Da Silva, De Bastos Pazini, Da Silva Martins and Rubenich2014; Batistella Pasini et al., Reference Batistella Pasini, Lúcio, Cargnelutti Filho, de Ribeiro, Zamberlan and Lopes2018), none of these contributions has studied the incidence of natural enemies during overwintering on alternative hosts. Also, this information about the host species cannot be simply extrapolated to the whole distribution area of this insect pest, because different management techniques are applied to the crop and also because the plant assemblage varies regionally in different parts of the insect's range. In this aspect, starting to integrate phylogenetic knowledge with ecological perspectives can improve the understanding of the evolution of insect–plant interactions (Winkler and Mitter, Reference Winkler, Mitter and Tilmon2008).
To elucidate the importance of native vegetation for population dynamics of T. limbativentris, and its relation with the incidence of natural enemies in rice agroecosystems, here we present results showing the insect's alternative host plants, the relative abundance/density that it reaches in these plants, and a preliminary assessment of the relationship between the plant phylogeny and insect abundance. Also, we report the impact that natural enemies have upon the survival of these pest populations in northeastern Argentina, which is the southern limit of the rice crops in the Neotropics. The primary aim of the study was to assess the hypothesis that, in the winter season when the rice crop is absent, the native vegetation inside rice fields provides an important refuge for T. limbativentris, and that within this habitat/refuge, winter enemies associated with the host plants exert top-down pressure that regulates insect population size.
Materials and methods
Study area
Sampling took place in commercial rice fields located in northeastern Argentina. This region contributes 85% of the national rice production and exports approximately 95% (BCSF, 2017), making Argentina one of the main rice exporting countries of South America (FAO, 2013). The study area climate corresponds to humid subtropical (Cfa) in the Köppen-Geiger system, with an average annual temperature of 21.7°C and average annual precipitation of around 1289 mm. Total rainfall in 2017 was 1975 mm, 43% higher than the annual historical value (Escalante and Fernandez, Reference Escalante and Fernandez2018).
Sampling was carried out during winter, which runs from mid-June until mid-September. The sites were sampled one time each between July and August 2017, which are usually the months with the greatest amount of cold days for this Neotropical area. However, as happened with the rain, the winter of 2017 was also more variable and warmer than the historical average, standing out July with a mean temperature of 17°C (Escalante and Fernandez, Reference Escalante and Fernandez2018).
In total, nine sampling sites were selected (fig. 1), which included both post-harvest rice plots and nearby native grassland vegetation. The sites were separated from each other by a minimum distance of 2 km. The irrigation system in the rice fields used water from the Paraná River.
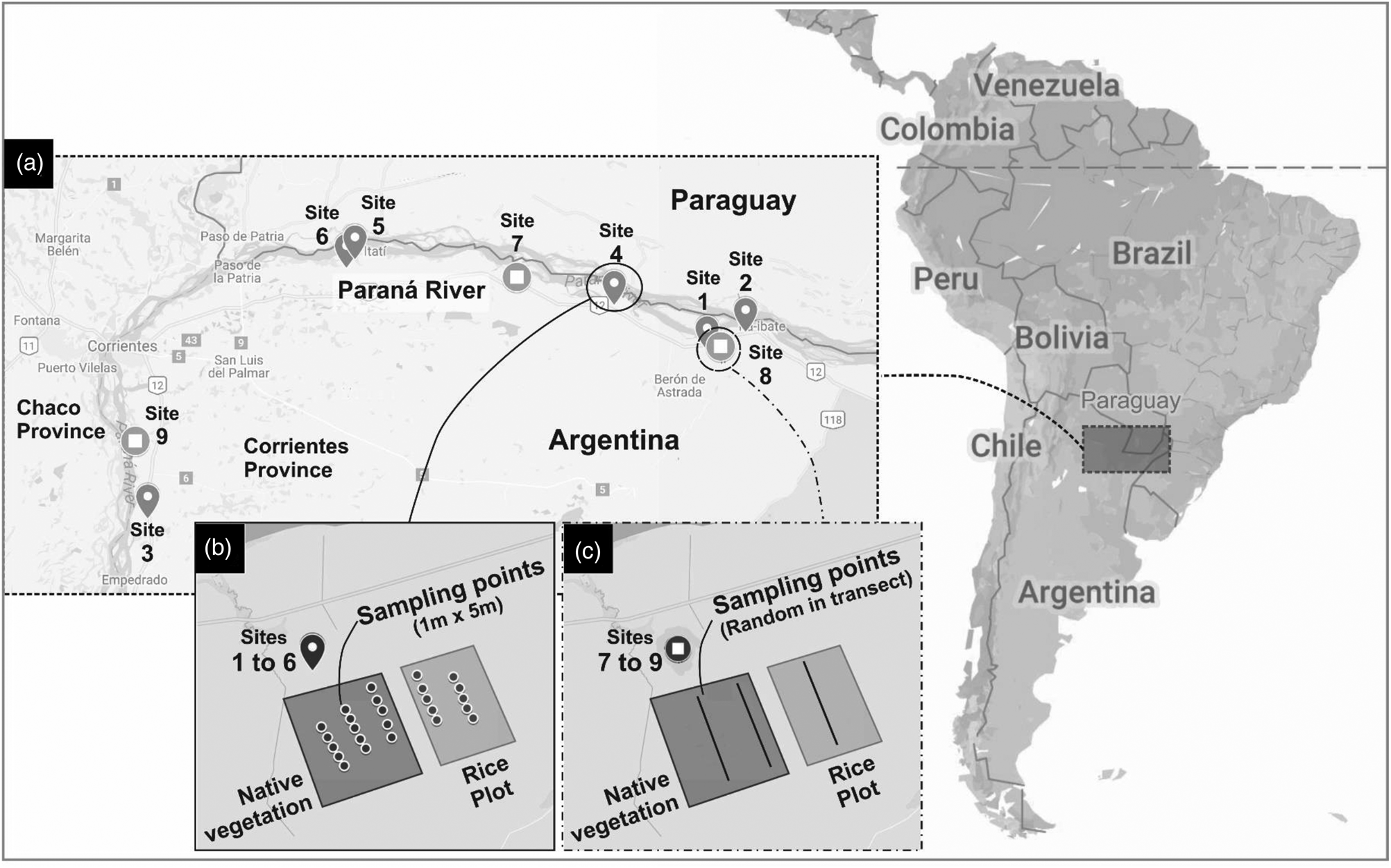
Fig. 1. Study area. (a) Sampling sites selected in Northeast of Argentina (Corrientes Province) (Site1: 27°28′53.73″S, 57°27′58.05″W; Site2: 27°26′71.61″S, 57°22′34.89″W; Site3: 27°49′46.26″S, 58°46′22.76″W; Site4: 27°23′16.16″S, 57°40′59.5″W; Site5: 27°18′39″S, 58°18′30.92″W; Site6: 27°17′34.25″S, 58°17′19.97″W; Site7: 27°19′35.9″S, 57°54′36.5″W; Site8: 27°28′13.8″S, 57°26′02.8″W; Site9: 27°39′57.5″S 58°48′06.5″W). (b) Systematic sampling points (sites 1–6). (c) Qualitative sampling points (sites 7–9).
Systematic sampling was carried out in six sites (fig. 1b). Due to heavy rain, in the remaining three sites, only random qualitative samples were taken to detect possible host plants and occurrence of T. limbativentris (fig. 1c).
Alternative winter host plants and relative abundance/density of T. limbativentris
The winter period was selected because this is when T. limbativentris populations are utilizing their alternative host plants. To determine host plants and the abundance of T. limbativentris, surveys were carried out on native vegetation adjacent to the cultivation plots, and on rice plots with stubble (fig. 1b).
Transects were established along a distance gradient from rice plots to the native vegetation, following Link et al. (Reference Link, Naibo and Pelentir1996) with some modifications. In rice stubble plots, two transects were established, one in the center and other at the edge of the plot. In native vegetation, three transects were established, the first located 2 m from the rice plot edge, the second 50 m, and the third 100 m from the rice plot. In fields where management techniques in use did not leave rice stubble, only native vegetation transects were set up.
Along each transect, sample units, each 1 m wide and 5 m long, were established at 5 points (fig. 1b) using the geolocation points in ‘Grid’ method with modifications (Botta et al., Reference Botta, Da Silva, De Bastos Pazini, Da Silva Martins and Rubenich2014). The location of the first sampling point in each transect was random and the following points were defined in a systematic way, separated from each other by a distance of 10 m. Each point was marked with GPS (N = 120 sampling-points). Stink bugs were collected manually, and plants with T. limbativentris occurrence were collected and taken to the laboratory for identification with specific keys (Lovato-Echeverria et al., Reference Lovato-Echeverria, Lopez, Leguizamón and Vanni2013).
A quadrat method (0.25 m2) was used to determine host plant coverage. At each sampling point in native vegetation, a quadrat was placed at random (total N = 90), and photographs were taken and subsequently analyzed to obtain the percentage cover of each host plant using the CorelDRAW X7© program. The analysis of these photographic quadrats is a quantitatively precise means of estimating vegetation cover (Capello et al., Reference Capello, Marchese and De Wysiecki2013).
In the laboratory, the total number of T. limbativentris individuals found in each site and in each host plant was recorded, quantifying the number of nymphs, adult females, adult males, and sex ratio (males/females). We also calculated the relative abundance and relative density of the insects for each host plant species during the winter season. The relative abundance (RaH p) of T. limbativentris during winter was calculated for each host plant species using the following equation:

where H p is the host plant species, n is the number of individuals of T. limbativentris found in a host plant in each sampling point, and N t is the total number of sampling points in which the host plant was found.
We also calculated the relative density (RdH p) of T. limbativentris during winter, in individuals/plant, for each host plant species taking into account those samples in which the insect and the plant species actually occur (He and Gaston, Reference He and Gaston2000), using the following equation:

where H p is the host plant species, n is the number of individuals of T. limbativentris found in host plant in each sampling point, P Hp is the number of individuals of host plant found with individuals of T. limbativentris in all sampling points.
We propose the following equation to obtain the average relative density of T. limbativentris during winter, per square meter (DH p), considering as a variable each species of host plant in a certain area of vegetation nearby to the rice plot:

where H p is the host plant species, n is the number of individuals of T. limbativentris found in host plant in each sampling point, A is the standard area of the sampling point, and SP is the total number of sampling points in vegetation nearby to the rice plot with the presence of the host plant. This calculation is based on the insect presence/preference for a particular plant species within a specific area, regardless of the composition of plants that accompany the host plant in the particular area. This can be offered as a practical management tool for use by rice researchers and producers in assessing winter abundance of T. limbativentris.
To confirm the diapause state of the individuals of T. limbativentris, dissections were performed on the specimens found alive and dead, considering the accumulation of fat bodies as an indicator of this state (Saulich and Musolin, Reference Saulich and Musolin2012). The proportion of fat bodies present in the abdomen was evaluated, classifying the samples according to three categories: 1 = from 0 to 33.33% of a full abdominal cavity, 2 = 33–66%, and 3 = 66–100% (Mielitz et al., Reference Mielitz, Becker and Romanowski1996). Mummified individuals (due to fungal mycelium growth) were not dissected, nor were those that were in a poor state of conservation. To confirm the occurrence of winter feeding, the presence of food in the digestive tract was also recorded.
Finally, to undertake a preliminary assessment of the relationship between plant phylogeny and insect abundance in the target area, we used the plant species found in both systematic and qualitative sampling. We reviewed the published phylogeny information available (APG IV, Reference APG2016) to define the clades at order level to which these plant species belonged. Then, we analyzed the host clades in relation to the relative abundance of T. limbativentris.
Impact of natural enemies in winter survival of T. limbativentris in host plants
For each host plant, we counted the total number of T. limbativentris that were found alive and dead during the winter, permitting the calculation of the winter survival/mortality percentage.
The individuals found dead were classified into two categories: undetermined mortality when individuals were found dead without signs of attack by natural enemies, and mortality due to entomopathogenic fungi, when individuals were found dead with entomopathogen mycelia. Mortality due to entomopathogenic fungi was considered because the attack of these fungi occurs when the individual is alive and their growth causes the death of the insect (Esparza Mora et al., Reference Esparza Mora, Conteiro Castilho and Fraga2017). Individuals mummified by fungal mycelium were sent to specialists for pathogen identification. The fungal material was isolated and incorporated into the fungi collection of the CIDEFI-CONICET-UNLP laboratory.
To corroborate the incidence of adult parasitoids, individuals of T. limbativentris dissected for the diapause study were also inspected for the presence of parasitoid larvae of known taxa that attack pentatomids (O'Hara, Reference O'Hara and Capinera2008).
Statistical analysis
Infostat Software (Di Rienzo et al., Reference Di Rienzo, Casanoves, Balzarini, Gonzalez, Tablada and Robledo2017) was used to perform all analyses. Generalized Linear Models (F; α = 0.05; significant when P < 0.05) were used to analyze the differences between host plants in relation to the relative abundance of T. limbativentris. GLM was also used to analyze the differences in relative and average densities, population characteristics, and survival of T. limbativentris, as well as plant coverage differences between plants and relationship between the relative abundance with the phylogenetic clades of host plants. Finally, the Pearson correlation coefficient (r; α = 0.05; significant when P < 0.05) was used to determine the relationship between the relative abundance of T. limbativentris and the plant cover of host plants.
Results
Alternative winter host plants and relative abundance/density of T. limbativentris
From the combined systematic and qualitative data, we found a total of 12 species of host plants (tables 1 and 2), and in these hosts, there were 160 hibernating individuals of T. limbativentris (averaged 3.57 ± 0.19 individuals). In the systematic sampling points in native vegetation (N = 90), we found nine species of host plants (table 1). Eight species were alternative hosts belonging to the families Poaceae, Cyperaceae, and Apiaceae. The remaining species was its main host, Oryza sativa, which was found as stubble in three sites, and as winter regrowth plants in one site. Regarding rice stubble (62.5 kg of stubble from 30 sampling points), only three individuals of T. limbativentris were recorded, showing that almost the entire overwintering population of the insect was in vegetation near rice plots (table 1). During qualitative samplings, we recorded five alternative host plant species of Poaceae (table 2).
Table 1. Relative abundance and density (per plant and per m2) of populations of T. limbativentris found in alternative host plants in all quantitative sampling points

Details and data from the population of T. limbativentris are presented. Standard error (±) is indicated. Within host plants, different letters (a, b, c) indicate significant differences in each category. RaH p: stink bugs relative abundance; RdH p: stink bugs relative density per plant; DH p: average relative density per m2 reached by stink bugs in presence of host plant.
1Considered as a weed within the rice fields; 2component of natural grassland within the rice fields; 3rice regrowth plants; 4rice stubble.
Table 2. Alternative host plants found with T. limbativentris during qualitative samplings. Relative abundance in each plant are presented

RaHp: stink bugs relative abundance.
aConsidered as a weed within the rice fields; bcomponent of natural grassland within the rice fields.
Significant differences in the relative abundance of T. limbativentris between host plant species were recorded (F = 4.64; P < 0.0001). Stink bugs were collected in all transects made in native vegetation (from 2 to 100 m). Of the total individuals, 47.24% were found at 50 m from the rice plots, without significant differences with respect to the other transects (F = 0.16; P < 0.8502). When considering the host plant species present in each distance from the rice plots, we found significant differences in relative abundance of T. limbativentris (F = 4.09, P < 0.0043). Among the host plants recorded at the sample sites, a greater relative abundance of T. limbativentris was obtained in Poaceae species Andropogon bicornis, Paspalum plicatulum, and in regrowth plants of O. sativa (tables 1 and 2).
The results of the various analyses of abundance used with our data for this stink bug vary depending on the parameter measured (table 1). It was observed that there are large differences if the data are analyzed in terms of relative abundance (RaH p) or relative density per plant (RdH p), or if the relative density per unit of square (m2) is analyzed considering the presence of the host plant species (DH p) in a specific area (table 1), although all three show significant differences.
The relative abundance (RaH p) showed clear differences between host plant species (table 1). The relative density per plant (RdH p), on the other hand, detected the marked difference in abundance of T. limbativentris between native vegetation and rice stubble (F = 15.91; P < 0.0001), but did not detect the differences or preferences between host plant species (table 1). When analyzing DH p, obtained with the proposed equation, significant differences were observed between host plant species (F = 4.64; P < 0.0001). This last analysis of density (DH p) presents high fidelity in relation to the statistical pattern of significance for relative abundance RaH p (table 1). This permits the conversion of relative abundance to relative density with the same pattern of statistical significance.
Significant differences in cover (%) were recorded between host plants in the study area (F = 18.48; P < 0.0001). Among the plants near the rice plot, A. bicornis, with a lower vegetation cover, had a higher relative abundance of T. limbativentris than the other host plants with higher cover (fig. 2), with abundance being significantly related to plant species cover (r = −0.22; P = 0.0138). It was also observed that the regrowth plants of the main host O. sativa with a high cover showed higher relative abundance of T. limbativentris, similar to what is seen during the rice cultivation phase (fig. 2).
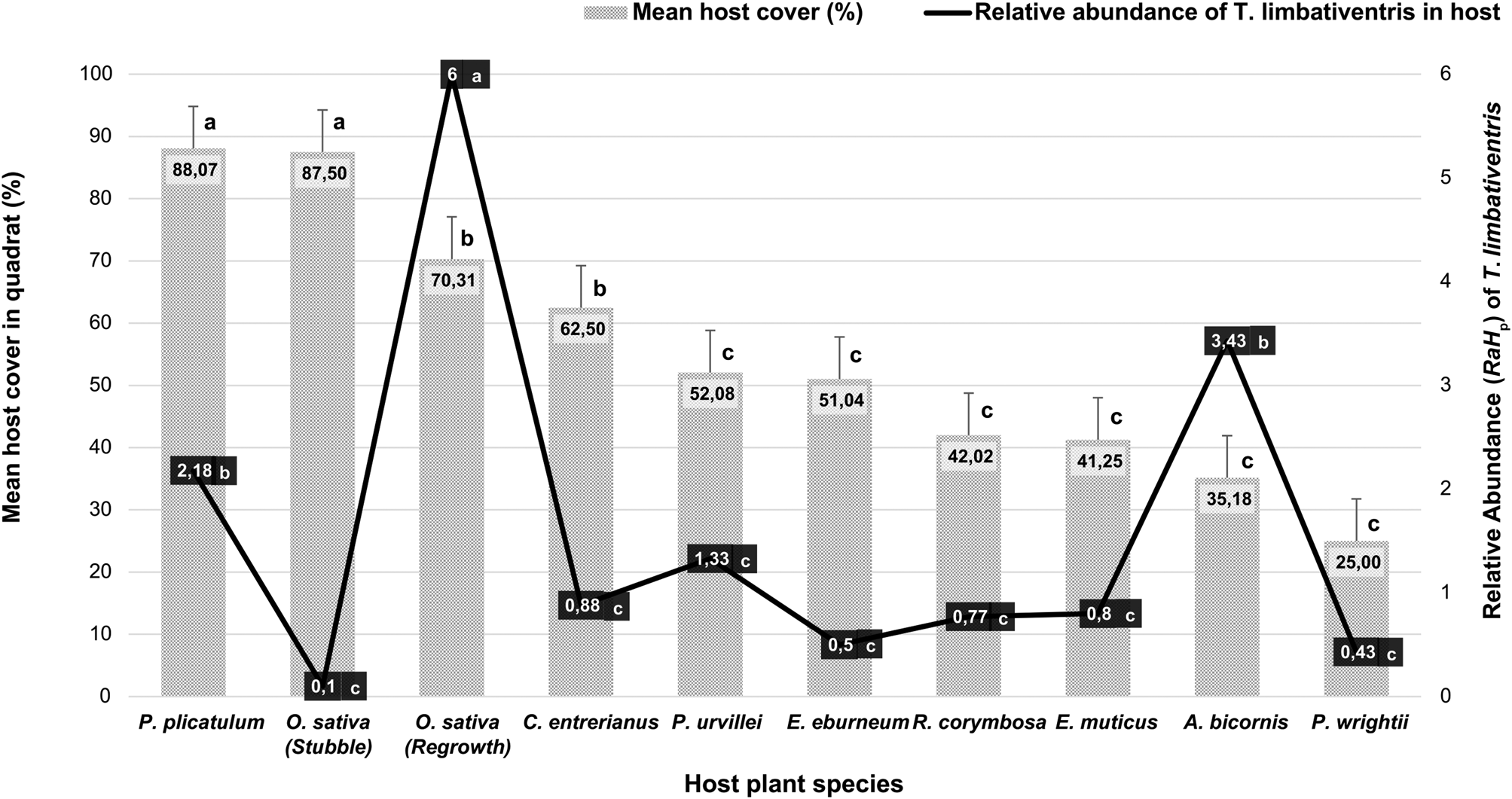
Fig. 2. Relationship between vegetal coverage (in quadrat) of host plants species (bars) and relative abundance (RaH p) of T. limbativentris found in each host plant (line) in all systematic sampling. Within each host plant, different letters (a, b) indicate significant differences in plant cover and relative abundance of T. limbativentris. For this figure, was used the data collected through systematic samplings.
Concerning the population status of T. limbativentris during winter in host plants (fig. 3), the sex ratio was 0.68, showing a moderate dominance of females. No eggs of T. limbativentris were found on any host and only 1.54% were dead nymphs belonging to the fifth instar (fig. 3a).

Fig. 3. Status of T. limbativentris population during winter. (a) Percentage of females (black bar), males (gray bar), and nymphs (white bar) per host plant during winter. (b) Percentage of individuals with digestive tract full (light gray bar) and abdomen with 100% fat bodies (dark gray bar). No significant differences between host plants were registered for any of these categories with the GLM (P < 0.05). For this figure, was used the data collected through systematic samplings.
For the evaluation of fat bodies, 69.23% of the total individuals collected were dissected. A high percentage of individuals with 100% of the abdomen containing fat bodies was obtained without significant differences between host plants (fig. 3b; F = 0.63; P = 0.7327), confirming that the population was in diapause. Regarding feeding, 40% of the population of T. limbativentris presented a digestive tract with food content, with the percentage varying between host plants, although not significantly so (fig. 3b; F = 0.65; P = 0.7149). A greater number of adults with a full stomach was recorded during July.
Regarding the host plant phylogeny, the plant species recorded belong to two accepted clades: the first is order Poales in which most of the recorded host plants are grouped (table 3). The second clade corresponds to the order Apiales in which only one species of host plant occurred (table 3). A significant difference in the relative abundance of T. limbativentris between clades was found (F = 4.09; P = 0.0457).
Table 3. Preliminary approach between host plant phylogeny and T. limbativentris abundance in the target area. Distribution of host plants registered in the phylogenetic clades and relative abundance (RaH p) of T. limbativentris in each clade are presented

*Represents significant differences registered between clades with GLM (P < 0.05).
Impact of natural enemies on winter survival of T. limbativentris in host plants
In all host plants registered, the percentage of T. limbativentris found alive was 56.92%, and were found dead the 43.08% of the individuals. Between hosts, a significant difference was obtained in the survival of stink bugs (F = 2.09, P = 0.0424), and also in stink bug mortality (F = 2.80, P = 0.0081). It was observed that survival was higher in populations related to A. bicornis and lower on Paspalum species, as well on E. muticus and E. eburneum (fig. 4a).

Fig. 4. Survival of T. limbativentris in host plants. (a) Total percentages of live individuals (in black, ‘Survival’), and dead individuals (in gray, ‘Mortality’) are presented. (b) Percentages of dead individuals without signs of attack by natural enemies (light gray, ‘Mortality: undetermined’) and dead with pathogens (dark gray, ‘Mortality: Entomopathogenic fungi’) are presented. Different letters (a, b) indicate significant differences between host plants in each category with the Generalized Linear Models (P < 0.05). In categories without a letter, no significant differences were registered. For this figure, was used the data collected through systematic samplings.
Out of 43.08% of dead insects, 23.21% of deaths were due to indeterminate causes without significant differences between host plants (fig. 4b; F = 0.56, P = 0.8104). The remaining 76.79% of deaths were caused by entomopathogenic fungi, and a significant difference was obtained between hosts (F = 3.49; P = 0.0015). The highest mortality due to entomopathogens was recorded in stink bugs on Paspalum species as well as on E. eburneum (fig. 4b).
Three entomopathogenic fungi (Ascomycota: Hypocreales) were found: Metarhizium anisopliae (Metsch.) Sorokin, Beauveria bassiana Bals. (Vuill), and Purpureocillium lilacinum (Thom) Luangsa-Ard, Hou-Braken, Hywel-Jones & Samson. The most abundant was M. anisopliae with 23.85% of the total percentage of mortality by pathogens recorded.
Regarding parasitoids of adult stink bugs, during dissections only one T. limbativentris individual was recorded with a protelean parasitoid. The larva found inside the stink bug was a dipteran of the Tachinidae family, a group of adult parasitoids known to attack T. limbativentris. The stink bug containing the tachinid was collected alive, which confirms the interaction with adult parasitoids during the winter. However, the percentage of attack was low, corresponding to just 0.77% of the total dissected individuals.
Discussion
This is the first study that analyzes both intrinsic (diapause and population status) and extrinsic population factors (host plants as a bottom-up factor and natural enemies as a top-down factor) regulating the abundance of a hemipteran pest species. Previous work on T. limbativentris reported the presence/absence of this insect hibernating in native plants (Aner and Becker, Reference Aner and Becker1993; Link et al., Reference Link, Naibo and Pelentir1996), or was restricted to studies of the ecology and seasonal variation of this insect in a dominant host plant around fields in a particular rice region (Klein et al., Reference Klein, Rodrigues Redaelli, Barcellos, Klein, Redaelli and Barcellos2012; Botta et al., Reference Botta, Da Silva, De Bastos Pazini, Da Silva Martins and Rubenich2014; Batistella Pasini et al., Reference Arihara2018).
For the studied rice region, in which herbaceous plant diversity is still conserved in areas near to rice plots, we found several species of host plants associated with this stink bug. According to our results, the hibernating population of T. limbativentris has different abundance in the 12 host plant species recorded in the field, with significant variations in relative abundance inside phylogenetic clades. This suggests that in this rice region, T. limbativentris may have a winter preference for certain plant species within the Poales clade, a group to which its main host O. sativa also belongs. This agrees with previous results about other insect species in which the phylogenetic relationships among plants can explain patterns of plant utilization (Gilbert et al., Reference Gilbert, Briggs and Magarey2015; Nakadai and Murakami, Reference Nakadai and Murakami2015). It is suggested, for future studies, to perform fine-scale phylogenetic distance analysis between host plant species in order to see if there is a winter host selection pattern directly related to the phylogenetic distance between these winter host plants and rice.
Seven out of 11 species of plants reported as alternative hosts of T. limbativentris during winter in this work are reported as such for the first time. One of the preferred hosts, A. bicornis, which has been reported several times, is a common plant in floodplain pastures and in areas affected by anthropogenic disturbances in the Neotropics, often being an important species within the plant succession in such habitats (Pinzón Pérez et al., Reference Pinzón Pérez, Castellano Castro, Cardona Cardozo, Mora Fernández, Vargas Ríos and León Sicard2011). Probably, this plant species is present in many South American rice areas, in abandoned or fallow fields within the crop rotation. Although A. bicornis is endemic to the Neotropical bioregion, and only introduced, outwith that area, to Hawaii (GBIF, 2019a), the 120 species of Andropogon show a pantropical distribution (Nagahama and Norrmann, Reference Nagahama and Norrmann2012). For two other species first recorded here as hosts of T. limbativentris, Rhynchospora corymbosa also shows a pan-tropical distribution (Guaglianone, Reference Guaglianone2001), while Elionurus muticus is widely distributed in both the Afrotropics and Neotropics, though rarely found in other tropical regions (GBIF, 2019b). This suggests that surveys of these, and other alternative host plants (some of which can also act as rice weeds) for similar hemipteran pests in rice fields throughout the tropics, might yield interesting results.
Our results also have strong methodological implications. Regarding the host plants recorded here, the results of density per square meter with the presence of a particular host plant (DH p), indicates that the abundance and density that T. limbativentris reaches in a particular area depend on the host plant species present in a square meter of vegetation next to the rice plot. The fidelity that shows the DH p with respect to the relative abundance (RaH p) allows us to recommend the use of this index in research on alternative host plants for insect pests. Our results also show that, for seasonal studies of T. limbativentris host plant species, expressing insect abundance in terms of relative density per plant (RdH p) is not an appropriate measure because it cannot reflect variations in the use/preference of plant species. This shows the importance of properly selecting the measure of density and the method to be used, as in previous studies for other pest species (Yamamura et al., Reference Yamamura, Imura, Morimoto and Ohto1999). The new index (DH p) that we propose in this work is relevant for pest research because it allows us to transform the relative abundance of a pest found in any area with any host plant (independent of the mix of plants in the native vegetation) to a type of data in density that is usually used to decide when pesticides should be applied (‘action thresholds’).
For example, for rice cultivated areas, the best-recognized action thresholds suggest that one individual of T. limbativentris per m2 feeding in the vegetative stage produces losses of 58.7 kg ha−1, and in the reproductive stage produces losses of 65.2 kg ha−1 (Costa and Link, Reference Costa and Link1992). In this research, the densities obtained for T. limbativentris in areas with the presence of each of their alternative host plants (DH p) are lower than the density established as the action threshold for T. limbativentris in areas with its main host O. sativa. However, the relative abundance and DH p of T. limbativentris in areas where winter rice regrowth plants are present turns out to be very similar to the threshold. This shows again that the population density which these stink bugs reach in their host plants could be related to the plant species itself and not to the time of year or to the physiological state of the insect.
This represents a challenge for the management of this pest because it would imply that T. limbativentris, in the presence of rice, will always reach higher densities than those it reaches in its alternative host plants in the adjacent environment. However, this also gives greater importance to the DH p conversion factor. With the transformation of pest abundance in a specific area in terms of density, field technicians can compare their field data with the action thresholds established. This offers a potential for further experimental research to establish winter action thresholds for T. limbativentris populations (and other pest species) in areas around the rice fields with the presence of each host plant species, in order to utilize alternative methods of controlling the winter populations before cultivation begins.
On the other hand, the negative correlation obtained between host plant cover and abundance of T. limbativentris shows that there exists an inversely proportional relationship between these two variables and that the greater extension in coverage that a winter host plant can reach, compared to another in a given area, may not be a selection factor for this stink bug. This does not match with the resource concentration hypothesis (RCH) proven for other insects (Nerlekar, Reference Nerlekar2018). The RCH proposes that specialist herbivorous insects should be more abundant in simple large patches of their host plants, because these patches offer all requirements for feeding, thereby helping the insects to stay longer, reproduce, and attain high densities in such environments (Root, Reference Root1973). The RCH apparently explains what normally happens with crop pests, in which the simple artificial environment offered by monoculture encourages higher abundances of ‘specialist herbivores’ (pests) than the native vegetation, with a higher diversity of plants around the field. Our results show that probably T. limbativentris in its winter host plants would be acting as a ‘generalist’ herbivore (maintaining some phylogenetic preference within the plant availability), while during the summer, it would act as a ‘species-specialist’ herbivore in rice cultivation. In this work, we present alternative host plant species belonging mostly to the Poales clade. Previous studies have shown that T. limbativentris density decreases drastically during the rice-growing season (spring–summer) in Poaceae host plant species in native vegetation near to rice plots, with the total individuals concentrating almost exclusively on rice cultivation (Batistella Pasini et al., Reference Arihara2018).
Unlike what happens with plant cover, in general, distance to the host plants from crop was found to be significant in the selection and winter distribution of the stink bug population. In subsequent studies, it would be useful to explore further the relevance of this distance metric at a specific level in individual rice fields. Also, host plant architecture could be relevant in influencing the abundance and distribution of this insect. Such studies may usefully contribute to the understanding of plant–pest interactions, thereby informing optimal pest management programs (Wang and Li, Reference Wang and Li2008).
Regarding insect population status, our finding that the individuals spend the winter as adults and in diapause, added to the absence of eggs and nymphs of the first instar, show that T. limbativentris does not reproduce on its winter hosts. This could also indicate that T. limbativentris currently depends on rice to fulfill its life cycle. This may be coincidental because in winter time, physiologically these insects remain in diapause, which is presumed to be closely related to temperature decrease and the photoperiod in hemipteran pentatomids (Saulich and Musolin, Reference Saulich and Musolin2012). Diapause for T. limbativentris was cataloged as ‘imaginal diapause’ (Klein et al., Reference Klein, Rodrigues Redaelli, Barcellos, Klein, Redaelli and Barcellos2012), which indicates that the reproduction is not initiated before diapause ends (Denlinger, Reference Denlinger, Resh and Cardé2009). In the target bioregion, where rice cultivation begins in spring–summer, it is probable that the presence of rice plants with high nutritional quality, and phylogenetically related to the ancestral native host plants, has facilitated feeding and reproduction for T. limbativentris populations when leaving diapause: a ‘bottom-up’ mechanism acting on this insect species. For T. limbativentris, we suggest that future studies should involve winter food preference experiments with diapause insects, in order to complement the knowledge of winter behavior for this pest species.
On the other hand, the high incidence of entomopathogens recorded indicates that there are top-down regulatory mechanisms acting naturally on the population of stink bugs during the winter season in these host plants. Our results show that entomopathogens reduce T. limbativentris population by one-third during winter in host plants, acting efficiently as winter biocontrollers. The winter survival of T. limbativentris was higher in A. bicornis, with lower fungal attack seen for insects occurring on this plant. Stink bug survival was lowest in Paspalum species, where there was higher insect mortality due to pathogens. These variations in fungal action between host plant species are relevant because they imply a host plant-mediated effect that favors entomopathogen attack. There is evidence that host plants can harbor entomopathogens on the surfaces of the leaves, favoring spore retention and fungal survival (Cory and Ericsson, Reference Cory and Ericsson2010) These plant-mediated effects are possibly related to leaf topology, to plant-structure surface chemistry, and to the microclimate offered by each host plant species, which are likely to differentially influence humidity and temperature conditions, these being important factors for fungal development, survival, and impact (Bugeme et al., Reference Bugeme, Maniania, Knapp and Boga2008; Cory and Ericsson, Reference Cory and Ericsson2010). This suggests that the importance of winter host plants for integrated management lies not only in contributing to pest detection, but also in the influence that these plants have on fungal establishment and the consequent natural regulation of T. limbativentris population by entomopathogens. Increasing these species of entomopathogenic fungi during winter in this zone could be an alternative to biological control for T. limbativentris.
Likewise, the finding of just one parasitized adult individual of T. limbativentris suggests that entomopathogens only would be acting as biological control agents during winter in the study area. The low incidence of parasitoids could be related to the refuge hypothesis in which the host plants provide escape zones to avoid the attack of natural enemies (Obermaier et al., Reference Obermaier, Heisswolf, Poethke, Randlkofer and Meiners2008). It could also be because the adult parasitoids, being insects, are also strongly affected by temperature, and it has been demonstrated in the case of the Tachinidae that have pupal diapause as an overwintering mechanism (Paur and Gray, Reference Paur and Gray2011).
Finally, considering the facts that irrigated rice usually uses water from natural sources, that intensive agriculture is one of the main anthropogenic activities generating habitat and biodiversity loss (Sánchez-Bayo and Wyckhuys, Reference Sánchez-Bayo and Wyckhuys2019); and that increasing food demand will require substantial increases in food production in coming years (FAO, 2013), it is clear that current agricultural practices for rice must be re-thought in order to reduce its ecological impact. Further development of ecologically-based management tools for rice agriculture, based on work such as that reported here, can have a major role to play in this process.
Conclusions
This research shows that during winter, native vegetation inside rice fields acts as an important refuge for T. limbativentris, and in these shelters, there occurs a natural top-down mechanism that regulates insect population by entomopathogenic fungi. Tibraca limbativentris populations spend the winter as adults in diapause and do not reproduce in the winter host plants, suggesting that this species could depend on O. sativa to fulfill its life cycle. The relative abundance/density which this pest reaches in its host plants could be related to the plant species itself and not to the time of year or to the physiological state of the insect. Our data show that there are both bottom-up and top-down mechanisms acting naturally in the winter regulation of T. limbativentris populations. This reinforces the importance of native vegetation around rice plots as a provider of ecosystem services for pest control in Neotropical rice-growing areas.
Our findings offer a tool for the detection of host plant species of T. limbativentris regardless of the plant composition around the crop. The transformation of abundance data through the DH p method allows researchers and field technicians to compare their winter field data with the action thresholds available. Further experimental research is needed to establish IPM schemes that allow for winter preventive actions on hibernating populations of T. limbativentris in native host plants. These investigations should evaluate the preservation of native vegetation areas near rice fields and the use of entomopathogenic fungi species found in the target area, as a biological control strategy for reducing T. limbativentris populations before sowing.
Acknowledgements
This study was funded by PICT 1910-2015, PI-17Q003, and PUE Project (Argentina). We thank Dr Andrea Toledo (CIDEFI-CONICET-UNLP, Argentina) for entomopathogenic fungi identification; and Dr Gimena Dellapé (Museo de La Plata, Argentina) for the Hemiptera taxonomic confirmation. We are grateful to Dr Kevin Murphy (University of Glasgow, Scotland) and Dr Martín Núñez (Comahue National University, Argentina) for critically reviewing the manuscript and offering useful comments and contributions. We also thank Agr.Eng. Javier Araujo of the Corrientes Rice Planters Association (ACPA), the rice producers in our target areas, and Agr.Eng. Nelson Niquén, the field campaign technician.