Introduction
For several decades, the conventional wisdom was the use of specialist natural enemies for each pest species; for example, Encarsia formosa Gahan and Phytoseiulus persimilis Athias-Henriot were exploited to restrict the population of greenhouse whiteflies and two-spotted spider mites (TSSM), respectively (Gould, Reference Gould1977; Messelink & Janssen, Reference Messelink and Janssen2009; Janssen & Sabelis, Reference Janssen and Sabelis2015). Consuming non-target pests and lower potential of population growth in generalist natural enemies are reasons that have encouraged farmers to use specialist natural enemies (Janssen & Sabelis, Reference Janssen and Sabelis2015). Whatever the reasons, because most crops are attacked by different pest species concurrently, the use of generalist natural enemies particularly predatory mites to control different pests has found many supporters in recent years (Fathipour & Maleknia, Reference Fathipour, Maleknia and Omkar2016). Phytoseiid mites which are beneficial biocontrol agents against different small insects and mites such as TSSM, whiteflies, and thrips act as generalist predators (Messelink et al., Reference Messelink, van Maanen, van Steenpaal and Janssen2008, Reference Messelink, Van Maanen, Van Holstein-Saj, Sabelis and Janssen2010). Amblyseius swirskii Athias-Henriot (Acari: Phytoseiidae) is a common predator that is used for restraining TSSM, Tetranychus urticae Koch, and greenhouse whitefly (GHWF), Trialeurodes vaporariorum Westwood, in strawberry greenhouses in many countries (Calvo et al., Reference Calvo, Knapp, van Houten, Hoogerbrugge and Belda2015). This species also has the ability to feed on non-prey food sources like pollen (Khanamani et al., Reference Khanamani, Fathipour, Talebi and Mehrabadi2017; Riahi et al., Reference Riahi, Fathipour, Talebi and Mehrabadi2017), hemipteran insect-produced honeydew (Nomikou et al., Reference Nomikou, Janssen and Sabelis2003; Juan-Blasco et al., Reference Juan-Blasco, Qureshi, Urbaneja and Stansly2012; Buitenhuis et al., Reference Buitenhuis, Murphy, Shipp and Scott-Dupree2015), and powdery mildew (Duso et al., Reference Duso, Pozzebon, Capuzzo, Bisol and Otto2003) as a source of water and complementary food (Nomikou et al., Reference Nomikou, Janssen and Sabelis2003). Using the alternative food or non-target prey has been mentioned as a negative property of the generalist predatory mites in some studies (Van Lenteren et al., Reference Van Lenteren, Babendreier, Bigler, Burgio, Hokkanen, Kuske, Loomans, Menzler-Hokkanen, Van Rijn and Thomas2003), whereas some of them have shown that it has positive effects on improving biological control strategies (Nomikou et al., Reference Nomikou, Janssen and Sabelis2003; Messelink et al., Reference Messelink, Van Maanen, Van Holstein-Saj, Sabelis and Janssen2010). Nevertheless, it has been hypothesized that the efficiency of different biological control agents such as phytoseiid mites could be promoted in the presence of alternative food and/or alternative prey, and pest species diversity (Nomikou et al., Reference Nomikou, Janssen and Sabelis2003; Messelink et al., Reference Messelink, Van Maanen, Van Holstein-Saj, Sabelis and Janssen2010). Buitenhuis et al. (Reference Buitenhuis, Murphy, Shipp and Scott-Dupree2015) employed A. swirskii in greenhouse crops and proved that it was able to control several pests at once. Along a similar vein, Messelink et al. (Reference Messelink, van Maanen, van Steenpaal and Janssen2008) showed that A. swirskii succeeded in restraining thrips and whiteflies when they were present together better than when they were used separately.
Since a simultaneous outbreak of several pest species in greenhouses took place in our study (Mortazavi et al., Reference Mortazavi, Fathipour and Talebi2017), we decided to choose this predator to control different pest species. T. vaporariorum and T. urticae are two destructive pests and control of them is vital in greenhouses. Several research works have confirmed the ability of A. swirskii to control the two mentioned pest populations in greenhouses (Nomikou et al., Reference Nomikou, Janssen and Sabelis2003; Rezaie et al., Reference Rezaie, Saboori, Baniamerie and Allahyari2013; Calvo et al., Reference Calvo, Knapp, van Houten, Hoogerbrugge and Belda2015; Riahi et al., Reference Riahi, Fathipour, Talebi and Mehrabadi2017), but there is no information about the impact of the shared predator on biological control for the case of a one-predator-two-prey system. Furthermore, these two pests can change the appearance of their host plants by making dense webs and whitefly-produced honeydew, which may alter the performance of A. swirskii; this should be considered as a pivotal factor on biological control (Sabelis & Bakker, Reference Sabelis and Bakker1992; Messelink et al., Reference Messelink, van Maanen, van Steenpaal and Janssen2008; Lemos et al., Reference Lemos, Sarmento, Pallini, Dias, Sabelis and Janssen2010). The application of different pollen grains as an alternative food source can also affect the predator's performance and some of them are influential when it comes to maintaining populations of generalist predatory mites in the absence of pests (Nomikou et al., Reference Nomikou, Janssen and Sabelis2003, Reference Nomikou, Janssen and Sabelis2010). Delisle et al. (Reference Delisle, Brodeur and Shipp2015) evaluated the suitability of different types of alternative food for A. swirskii and found apple pollen as the best food source for this species in the absence of the western flower thrips on chrysanthemum. Similarly, Riahi et al. (Reference Riahi, Fathipour, Talebi and Mehrabadi2017) surveyed the impact of different pollen grains on the biological parameters of A. swirskii and found the maize pollen as an appropriate food source for mass rearing. One of the reasons why farmers are reluctant to use generalist predatory mites is the lack of coincidence of their dynamics with the pest population, which can be managed by utilizing a proper food source before the pest outbreak (Janssen & Sabelis, Reference Janssen and Sabelis2015). Moreover, taking advantage of the complementary food along with the target pest in some predators leads to an increase in their performance and in some cases to inverse results (Van Lenteren et al., Reference Van Lenteren, Babendreier, Bigler, Burgio, Hokkanen, Kuske, Loomans, Menzler-Hokkanen, Van Rijn and Thomas2003; Nomikou et al., Reference Nomikou, Janssen and Sabelis2010; Janssen & Sabelis, Reference Janssen and Sabelis2015). Hence, it is necessary to study the positive or negative effects of the presence of different pollen grains along with the target pest on the efficiency of this predator.
A. swirskii is a popular species in many countries, especially for the control of different pests such as spider mites, whiteflies, and thrips on strawberry plants (Camarosa variety) in greenhouses, yet some farmers complain about its low population growth and poor efficiency in pest control and do not achieve the desired outcome from the use of this predator in their greenhouses. Bearing this inconvenience in mind, we conducted our study to evaluate the factors that reduce the efficiency of A. swirskii in some greenhouses and to seek solutions to improve its performance. The maize pollen (Zea mays L.) was proposed as an alternative food in the presence and absence of the prey to investigate the contribution of this pollen to the performance of A. swirskii restraining TSSM and GHWF. The maize pollen was selected on account of its low price and high availability for most farmers (Riahi et al., Reference Riahi, Fathipour, Talebi and Mehrabadi2017). Moreover, due to the concurrent incidence of the two aforementioned pests on strawberry plants, the effect of the presence of GHWF on the performance of the predator controlling TSSM (as a target pest on strawberry plants) was explored. For this purpose, the population growth, reproduction rate, and predation rate of A. swirskii were assessed in three different conditions including feeding on each of the mentioned pests (i) with and (ii) without the pollen and honeydew, and (iii) the combination of the pests without the pollen. Any changes in the survival, fecundity, and predation rate of the predator can alter the predator's population and affect the success of biological control management (Fathipour & Maleknia, Reference Fathipour, Maleknia and Omkar2016). There are some studies that evaluated the effect of pest species diversity, different pollen grains, or even the effect of dense webs on population dynamics of different predators; however, none of them has examined the impact of these factors on the biological parameters and the predation rate of this predator against the target pest. Accordingly, this study can be helpful in providing a more sophisticated view of predator's performance under these conditions.
Material and methods
Rearing
Whitefly, TSSM
The individuals of TSSM and GHWF were collected from naturally infested plants at the campus of Faculty of Agriculture, Tarbiat Modares University. TSSM were reared on bean plants, Phaseolus vulgaris L. (Khanamani et al., Reference Khanamani, Fathipour and Hajiqanbar2013), and greenhouse whiteflies were reared on eggplants (Solanum melongena L.). All pests were kept on plants in the greenhouse (25 ± 3 °C, 65 ± 5% RH), under natural photoperiod. For the laboratory experiments, the strawberry plants (var. Camarosa) were also grown in small pots in similar greenhouse conditions.
Predatory mite
The culture of A. swirskii was purchased from Koppert Biological Systems (Special; Berkel en Rodenrijs, The Netherlands). These mites were reared on a plastic sheet, placed on top of water-saturated foam in a Plexiglas box containing water. The margins of the sheet were covered by moist tissue papers to provide moisture and prevent predators from escaping. The TSSM- and GHWF-infested strawberry leaves were prepared as a food source on the rearing arena twice per week. The colonies of the predatory mite were kept in a growth chamber at 25 ± 1 °C, 65 ± 5% RH and a photoperiod of 16L:8D hours.
Experimental design
With the aim of comparing the effects of spider mite webbing, whitefly-produced honeydew, maize pollen, and pest species diversity on the performance of A. swirskii, the following tests were performed. The treatments consisted of: (I) without any food as a control treatment, (II) about 0.005 g maize pollen alone, (III) TSSM eggs alone, (IV) TSSM eggs + ca 0.005 g maize pollen, (V) TSSM eggs on leaf discs covered by spider mite webs, (VI) GHWF eggs alone, (VII) GHWF eggs + ca 0.005 g maize pollen, (VIII) whitefly-produced honeydew alone, and (IX) mixed diet (TSSM eggs + GHWF eggs).
To begin the experiment, 15 TSSM adults and 20 GHWF adults were separated from the original colony and placed individually on each excised strawberry leaf disc (5 cm diameter). The leaf discs were placed on wet cotton inside the Petri dishes (8 cm diameter, 1 cm height) and their margins were closed by wet tissue. The Petri dishes were sealed when the lids were closed in order to prevent the escape of the pests. The circular holes were made on the lids of each Petri dish, which were covered by the mesh polyester netting to allow for ventilation (similar to the method of Bostanian et al., Reference Bostanian, Beudjekian, McGregor and Racette2009). After 2 days, the adults were removed by a paintbrush, and 50 eggs of TSSM or GHWF were left on each leaf disc. The eggs used as a food source in the treatments were covered with spider mite webs or whitefly honeydew due to their immobility on the leaves. Treatment III was similar to treatment V, but it was conducted in the presence of the TSSM webs. Therefore, the webs and adults on the infested leaves were gently removed with a fine needle in treatment III, but the adults were removed without damaging the web structure in treatment V.
To prepare both of the TSSM and GHWF eggs (treatment IX), 20 adults of both species were placed simultaneously on each leaf disc. After 2 days, the extra eggs were removed to reach 25 TSSM eggs + 25 GHWF eggs on leaf discs. The previous predation tests showed that these numbers of prey were enough to avoid starvation and ensure maximum prey consumption. For treatment VIII, the pre-infested leaves by T. vaporariorum (covered by honeydew) were used. All of the preys were removed and only the exuviae and honeydew were left on the leaf disc. Clean strawberry leaves without any pests were employed as the control.
Effect of different diets on development, survival rate, oviposition rate, and life table parameters of A. swirskii
At the same time as the experimental design was set up, the adults of the predatory mite were transferred onto strawberry leaf discs (5 cm diameter) on a cotton layer in a Petri dish and were fed with maize pollen (Z. mays L.). The pollen grains were collected from the cultivated plants in the Faculty of Agriculture campus at Tarbiat Modares University, Tehran, Iran, and were oven dried at 37 °C for 48 h. The Petri dishes were filled with water to keep the leaves fresh and a few cotton threads were placed on each leaf as a shelter and oviposition site. Furthermore, to prevent the predators from escaping, the margins of the leaves were covered by moist tissue papers. After one day, the adults were eliminated and the eggs were allowed to complete development. Water was added daily and leaves were changed if needed. The predatory mites were reared for two generations and the F3 eggs were used in all treatments.
The eggs of A. swirskii, <24 h old, were transferred one by one on the leaf discs of each treatment, which were prepared in the previous section. Each leaf disc, considered as one replicate and up to 90 replicates were used per treatment. The leaf discs were checked daily to record the survival and the growth stage of the predator. After the adult emergence, every female was coupled with one male from the stock colony and these couples were kept together up to the end of the experiment. The dead and escaped males were replaced by new ones from the stock colony and the survival and oviposition rate of adult mites were calculated daily. Also, pre-oviposition, oviposition, and post-oviposition periods of females were scored every day. All the experimental units were kept in a growth chamber (25 ± 1 °C, 65 ± 5% RH and a photoperiod of 16L:8D hours).
Predation rate
The predation rate of A. swirskii on TSSM in the presence and absence of maize pollen, GHWF, and spider mite webbing, as well as the predation rate of the predator on GHWF in the presence and absence of maize pollen and GHWF-produced honeydew were evaluated during the previously examined study. Due to the presence of both female and male predators on each leaf disc and to avoid confusion in calculating the consumption rate of male and female individuals, a separate experiment was performed to calculate the proportion of consumption rate of adult female in each treatment. The third-generation eggs of the predator were kept under the same conditions explained in the Experimental design section. When the mites reached adulthood, ten females and ten males were allowed to mate for 24 h, after that 50 TSSM or GHWF eggs were left on leaf discs described before. The consumed prey was recorded and replaced every day by new ones. The feeding rate of both female and male predators was recorded individually until the death of mites. In the end, the ratio of feeding rate of female to male was determined every day. To obtain the predation rate of A. swirskii in the presence or absence of maize pollen, spider mite webs, and honeydew, the number of killed TSSM and GHWF was recorded daily through the life table experiments until the death of all individuals.
Statistical analysis
For the life table experiment, all individuals’ data were analyzed according to the age-stage, two-sex life table theory (Chi & Liu, Reference Chi and Liu1985; Chi, Reference Chi1988). Analysis of variance and multiple comparison test (Tukey) at P < 0.05 were opted for assessing the developmental duration of the immature and adult stages, oviposition period, and daily fecundity of A. swirskii on different treatments. The population growth parameters (GRR, R 0, r, λ, and T) and the age-stage-specific survival rate (s xj) (x is the age and j is the stage); age-specific survivorship (l x); the age-specific fecundity (m x); and age-stage-specific fecundity (f xj) were estimated for each treatment by TWOSEX-MS Chart program (Chi, Reference Chi2016a). Also, bootstrap method was applied for the estimation of standard error, and multiple comparisons were done by the paired bootstrap test with 40,000 samples (Riahi et al., Reference Riahi, Fathipour, Talebi and Mehrabadi2017).
The computer program of CONSUME-MS Chart (Chi, Reference Chi2016b) was used to analyze the daily consumption of all individuals. The predation rates in different stages were compared running the Tukey test (P < 0.05). Furthermore, according to Chi & Yang (Reference Chi and Yang2003), the parameters C 0 (the net predation rate; mean number of prey consumed by an individual predator during its life span), Q P (the transformation rate from prey population to predator progeny; the number of prey needed for the production of an offspring from a predator), ψ (the stable predation rate; the total predation capacity of a stable population whose maximum size is one), and ω (finite predation rate; the predation potential of predator population by combining its growth rate (λ), age-stage predation rate (c xj), a stable age-stage structure (a xj)) were calculated (Fathipour & Maleknia, Reference Fathipour, Maleknia and Omkar2016). In addition, the C 0, Q P, ψ, and ω were compared by bootstrap test using TWOSEX-MS Chart program (40,000 samples).
Results
The individuals of A. swirskii completed their immature stages in all the treatments except on clean leaves. The duration of each immature stage, adult longevity, adult pre-oviposition period (APOP), total pre-oviposition period, oviposition period, total fecundity, and total life span of A. swirskii were detailed in Table 1, indicating the clear effect of the diets on the biological parameters of A. swirskii. The experiments showed that few numbers of A. swirskii reached adulthood on the clean leaf disc, but they were survived by being fed on maize pollen or GHWF-honeydew. Although A. swirskii fed on maize pollen and honeydew laid less number of eggs and had lower survival rate than those fed on the TSSM or GHWF eggs, the complementary food contributed significantly to the survival of the predator population in the absence of the pests (Table 1). The pre-adult developmental time of A. swirskii in the presence of pollen was not different from that involving honeydew, though the survival and fecundity of the predator were significantly higher when the pollen was introduced. A. swirskii had the ability to oviposit on honeydew and pollen as well, but its total fecundity was significantly lower when fed on GHWF (3.91 ± 0.44 and 17.3 ± 0.96 eggs/female on honeydew and GHWF, respectively). Our results showed that although this predator was more capable of feeding, surviving, and ovipositing on TSSM eggs than on GHWF eggs, the webs brought about a deterioration in its performance by reducing the adult longevity and fecundity. The TSSM webs adversely affected the oviposition of A. swirskii, which delayed the period starting with the adult emergence to the first oviposition (longest APOP) and decreased the oviposition rate compared with the other treatments. The total fecundity of A. swirskii on TSSM was about 20 times greater in the absence of the webs (20.25 ± 1.13 eggs/female) than in their presence (1.52 ± 0.14 eggs/female).
Table 1. Duration of development (day) and fecundity (egg) (±SE) of Amblyseius swirskii fed on different food diets.

Means followed by different letters in the rows are significantly different (P < 0.05, Tukey).
APOP, adult pre-oviposition period (the duration from adult emergence to first oviposition).
TPOP, total pre-ovipositional period (the duration from egg to first oviposition).
As it is apparent from Table 1, the presence of alternative food and prey along with TSSM eggs had a notable impact on the APOP and total fecundity of A. swirskii. Feeding on mixed diet comprised of GHWF + TSSM led to the shortest APOP and the highest total fecundity of the predator (35.93 ± 1.38 eggs/female) compared with the other diets. Moreover, the fecundity of A. swirskii fed on TSSM was 20.25 ± 1.13 eggs/female, and was enhanced to 26.5 ± 1.16 eggs/female in TSSM + pollen diet. Notwithstanding that the presence of pollen along with GHWF had a positive effect on the fecundity and total lifespan of this predator compared with its absence, it was not substantially different.
The female adult longevity of A. swirskii differed markedly among the adopted treatments: the longest and the shortest longevity was observed, respectively, in diets of TSSM + GHWF (42.17 ± 1.42 days) and in the presence of the spider mite webbing (10.88 ± 0.42 days). Also, feeding on the combination of both pests resulted in the longest total lifespan in both sexes compared with feeding on the other diets.
Survival and fecundity curves
The age-stage-specific survival rate (s xj) of A. swirskii on different diets is illustrated in fig. 1. The probability that a newborn egg survived to the female adult stage was 0.62 on mixed diet (TSSM + GHWF) and 0.38 in the presence of spider mite webbing, which are the highest and lowest s xj among the treatments. Furthermore, fig. 1 illustrates the overlap of different life stages during the developmental period. Fig. 2 compares the age-specific survivorship (l x); the age-specific fecundity (m x), and the age-stage-specific fecundity (f xj) of A. swirskii on different diets. The highest and lowest values of the daily age-specific oviposition of A. swirskii were 1.41 and 0.27 eggs on mixed diet (TSSM + GHWF) and in the presence of spider mite webbing, respectively, which occurred at the age of 13 and 16 days, respectively.

Fig. 1. Age-stage-specific survival rate (s xj) of Amblyseius swirskii fed on different food diets.

Fig. 2. Age-specific survivorship (l x), age-stage fecundity of female (f xj), and age-specific fecundity (m x) of Amblyseius swirskii fed on different food diets.
Life table parameters of A. swirskii
The age-stage, two-sex life table parameters of A. swirskii are presented in Table 2. The highest values of the intrinsic rate of increase (r), finite rate of increase (λ), net reproductive rate (R 0), and gross reproductive rate (GRR) were obtained on the mixed diet (TSSM + GHWF). By contrast, the spider mite webbing resulted in a considerable reduction in these parameters.
Table 2. Population growth parameters (mean ± SE) of Amblyseius swirskii on different food diets.

Means followed by different letters in the same column are significantly different between diets using the paired bootstrap test at 5% significance level.
Predation rate of A. swirskii
The predation rate of the different life stages, net, stable, and finite predation rate of A. swirskii feeding on TSSM and GHWF in the absence and presence of pollen, whitefly-produced honeydew, and spider mite webbing is shown in Table 3. Our results did not record any remarkable predation activities by the predator's larvae in all the treatments. The highest predation rate for all of the stages and both sexes was observed on TSSM eggs, which diminished in the presence of webbing by about 90%. The female pre-adult stages consumed conspicuously greater numbers of TSSM eggs (21.76 ± 1.16 preys) in the absence of the webs in comparison with their presence (5.46 ± 0.29 preys). Furthermore, the highest predation rate of the female in adult stage (632.9 ± 44.79 preys) and female in total lifespan (656.44 ± 44.64 preys) was observed on TSSM in the absence of webbing.
Table 3. Mean predation rates (±SE) of Amblyseius swirskii fed on different food diets.
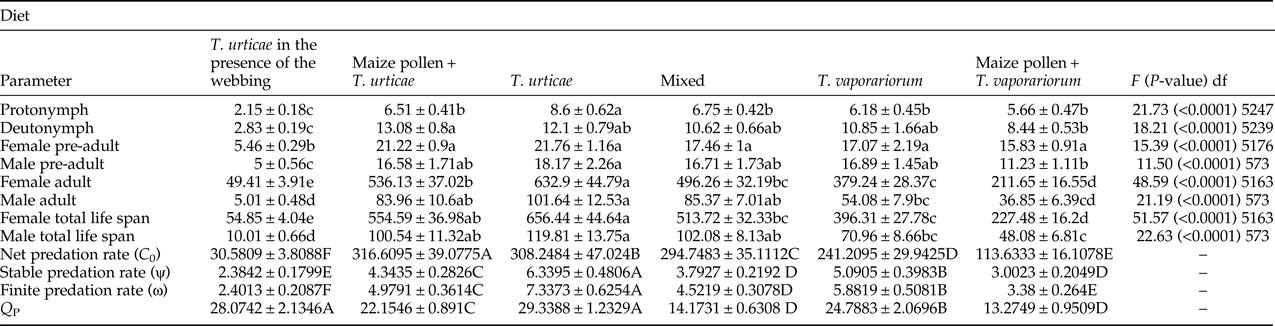
Small different letters in each row are significantly different (P < 0.05, Tukey) and the capital different letters are significantly different using paired bootstrap test (with 40,000 resampling).
Our results implied that although A. swirskii consumed lower numbers of GHWF than those of TSSM in all the growth stages, the combination of two preys (TSSM + GHWF) had a negative effect on the predation rate on TSSM. As mentioned before, the female adult predation capacity of A. swirskii feeding on TSSM (on strawberry leaves) was about 633 TSSM eggs, which declined to 496.26 ± 32.33 TSSM eggs in the presence of GHWF. Moreover, the combination of TSSM + pollen had an adverse effect on the consumption rate of the female and male adults on TSSM. For instance, the female predation rate in a total life span on TSSM dropped by about 15% in the presence of pollen. Furthermore, the value of predation rate of the female adult on GHWF was 379.24 ± 28.37 preys, but it decreased to 211.65 ± 16.55 preys in the presence of pollen along with GHWF.
Fig. 3 illustrates the trends in the age-stage-specific predation rate (C xj) referring the average number of prey consumed by an individual of age x and stage j. The predation rates of the different stages of A. swirskii varied dramatically between the different treatments. The age-stage-specific consumption rate of A. swirskii was enhanced through increasing the life stage of the predator in all the treatments, so the adult stage was the more voracious stage. The highest numbers of prey consumed by females were 30.22, 23.41, 15.88, 12.62, 22.68, and 5.41 preys at the age of 14, 25, 13, 20, 15, and 11 days on TSSM, TSSM + pollen, combination of TSSM + GHWF, GHWF + pollen, GHWF alone, and in the presence of spider mite webbing, respectively.

Fig. 3. Age-stage-specific predation rate (C xj) of Amblyseius swirskii fed on different food diets.
The net predation rates (C 0) were significantly different among the treatments, and the highest and lowest values obtained on the diet of TSSM and in the presence of spider mite webbing were 308.24 ± 47.02 and 30.58 ± 3.8 preys, respectively. In addition, the transformation rate from prey population to predator offspring (Q P) was 29.33 ± 1.23 preys on the diet of TSSM, then decreased to 28.07 ± 2.13, 22.15 ± 0.89, and 24.78 ± 2.06 preys in the presence of webbing, on TSSM + pollen and GHWF, respectively.
Moreover, as can be seen in Table 3, the highest and lowest values of other parameters such as stable predation rate (ψ) and finite predation rate (ω) were obtained on TSSM in the absence and presence of webbing, respectively.
Discussion
The predator efficiency in restraining pests depends on their predation rate and population growth on the target pest (Riahi et al., Reference Riahi, Fathipour, Talebi and Mehrabadi2016). Many studies have demonstrated that there are several factors contributing to the success of a biological control system; in this regard, some of the factors that can increase and decrease the performance of A. swirskii were evaluated in this study. The capability of TSSM to produce complex webs, which is recognized as a functional anti-predator strategy in the genus Tetranychus, is one of these factors that reduces the efficiency of most phytoseiid predators (Sabelis & Bakker, Reference Sabelis and Bakker1992; Lemos et al., Reference Lemos, Sarmento, Pallini, Dias, Sabelis and Janssen2010). In our study, despite the high survival rate of A. swirskii feeding on TSSM on clean leaves, the survival, and fecundity of this predator diminished in the presence of webs. The predation rate of the predator on TSSM in the presence and absence of the spider mite webbing was also determined. This showed that although A. swirskii can potentially feed on TSSM, the webs lead to a significant reduction in predation rate. These webs can decrease the predation rate of predators through impeding their movement and restricting their foraging, and eventually affect their growth rate, survival, and fecundity (Weis et al., Reference Weis, Smith and Santiago-Bass2000; Fathipour & Maleknia, Reference Fathipour, Maleknia and Omkar2016). It should be noted that eggs were used in this study as a food source, but the active immature stages are also found in nature, which cannot be easily predated because of their ability to escape and hiding in the webs (Messelink et al., Reference Messelink, Van Maanen, Van Holstein-Saj, Sabelis and Janssen2010). These results indicated that A. swirskii has no ability to cope with the webs, which is in line with the findings of Messelink et al. (Reference Messelink, Van Maanen, Van Holstein-Saj, Sabelis and Janssen2010) who stated that A. swirskii cannot sufficiently control TSSM in the presence of spider mite webbing. The adaptation of predators to these webs is dependent on the patterns of their mid-dorsal and lateral setae as well as their body size (Lemos et al., Reference Lemos, Sarmento, Pallini, Dias, Sabelis and Janssen2010). It is worth mentioning that only few species of phytoseiid mites such as P. persimilis can overcome these webs (Sabelis & Bakker, Reference Sabelis and Bakker1992; Messelink et al., Reference Messelink, van Maanen, van Steenpaal and Janssen2008; Lemos et al., Reference Lemos, Sarmento, Pallini, Dias, Sabelis and Janssen2010). Based on these results, it can be suggested that A. swirskii can be a potential predator of TSSM and is able to effectively lessen the TSSM population at the beginning of the TSSM colonization. Thereby, the introduction and persistence of A. swirskii population in crops prior to TSSM infestation, which can be supplied by providing the alternative food or prey, is very important (Nomikou et al., Reference Nomikou, Janssen and Sabelis2003). Consequently, we investigated whether spider mite control by A. swirskii would be improved by the presence of maize pollen or GHWF as an alternative food. There are quite a number of pieces of research concerning the effects of pollen and pest species diversity on the predator population density, but there is not much work about the impact of these factors on survival, fecundity, and predation rate of the predators at the same time (Messelink et al., Reference Messelink, van Maanen, van Steenpaal and Janssen2008; Nomikou et al., Reference Nomikou, Janssen and Sabelis2010; Riahi et al. Reference Riahi, Fathipour, Talebi and Mehrabadi2017) proved that some of pollen grains such as almond pollen are nutrient-rich food source and even are more suitable than target pest for predators, but in this study, despite the satisfactory survival of A. swirskii on maize pollen, their feeding on pollen resulted in lower reproduction rates than those rates related to TSSM or GHWF. This reduction in fecundity and longevity is probably due to the lower nutritional quality of the maize pollen compared with the main pests (Riahi et al., Reference Riahi, Fathipour, Talebi and Mehrabadi2016). Nevertheless, based on our findings, we suggested that the maize pollen can be utilized as an alternative food in the absence of target pest to help the predator's establishment on the pest-free corps or on the crops with the low pest density. Although other pollen grains with higher quality can be put to use for supporting A. swirskii at the beginning of pest infestation (Riahi et al., Reference Riahi, Fathipour, Talebi and Mehrabadi2017), the maize pollen was chosen thanks to its availability and low price. Hence, it is recommended that farmers protect the predator population by planting maize next to the main plants and providing sufficient pollen. Similar results were also observed in the presence of whitefly-produced honeydew, which caused the persistence of predator population and higher survival on the diet of honeydew in comparison with the clean leaf tissue. GHWF-honeydew is a favorite diet for phytoseiid mites owing to its rich source of sugar, and it can therefore be employed as an alternative food in the absence of pests (Nomikou et al., Reference Nomikou, Janssen and Sabelis2010). Honeydew is an excretion product of hemipteran insects and its quality varies depending on the pest species and host plant species. This changeable quality of honeydew can affect different predators in various ways (Nomikou et al., Reference Nomikou, Janssen and Sabelis2003). For instance, in this study, feeding A. swirskii on GHWF-honeydew led to lower oviposition and development rates than those rates achieved on GHWF; having said that, Ragusa & Swirski (Reference Ragusa and Swirski1977) demonstrated the positive effect of coccid produced on the survival and reproduction of A. swirskii. Nevertheless, in both experiments, the honeydew resulted in a proper pest control by supporting the predator population. This ability of feeding on alternative food sources such as pollen and honeydew can open up opportunities for farmers to establish such predators in the absence of the pests (Nomikou et al., Reference Nomikou, Janssen and Sabelis2010).
The alternative food sources can also be used along with the target prey, which can be considered as a mixed diet and may have a positive or negative effect on the performance of the predatory mites controlling target pests (Janssen & Sabelis, Reference Janssen and Sabelis2015). The diversified pest species is another form of the mixed diet, which have a significant effect on predator performance (Messelink et al., Reference Messelink, Van Maanen, Van Holstein-Saj, Sabelis and Janssen2010). Many studies have shown the ability of A. swirskii in control of TSSM and GHWF, separately (Riahi et al., Reference Riahi, Fathipour, Talebi and Mehrabadi2017); yet according to the fact that both pests often appear simultaneously, emphasizing on the interactions between these pests and the shared predator is critical to the successful implementation of an augmentative biological control. It should be noted that since TSSM is more destructive than GHWF and regarded as the common pest of the strawberry plant (Camarosa variety) in Iran, it was selected as a target pest in the combination of TSSM + GHWF eggs. Using the maize pollen along with the pest species (pollen + TSSM and pollen + GHWF), as well as the presence of other pest species (GHWF along with TSSM), showed complex results in this study. The simultaneous presence of maize pollen and prey brought about a reduction in the A. swirskii predation rate on TSSM and GHWF. Moreover, this predator consumed almost half of the number of TSSM eggs in the presence of GHWF compared with the treatments when they were offered separately. The high values of the life table parameters such as r, λ, and R 0 in the mixed diet (representing high longevity and fecundity) on one hand and the reduced predation rate on the target prey on the other hand may deliver contrasting results which made it difficult for us to decide whether or not to use different food sources along with target pest. In this respect, it was suggested that in spite of the presence of alternative food/prey, resulting in the satiation of the predator and finally a decline in the predation rate of predator on main pest (Holt & Lawton, Reference Holt and Lawton1994; Nomikou et al., Reference Nomikou, Janssen and Sabelis2010), the increase in longevity and reproduction rate in predators can make up this deficiency (Nomikou et al., Reference Nomikou, Janssen and Sabelis2010). Mixed diets are sometimes better food sources and can yield higher complementary nutritional values than the single ones, thus may lead to boosted fecundity and longevity in predators (Nomikou et al., Reference Nomikou, Janssen and Sabelis2003). This increased fecundity and longevity of a predator can raise the value of predator density and subsequently lower the number of prey population on plants with alternative food/prey in comparison with the food/prey-free plants. These results are in agreement with Messelink et al. (Reference Messelink, van Maanen, van Steenpaal and Janssen2008) and Swirski (Reference Swirski1967) indicating that the presence of western flower thrips along with GHWF and eriophyid mites plus castor bean pollen can improve the efficiency of predatory mites.
According to the results, it is of great importance to know when to release or how to keep the predator population in the absence of pests. This should be especially taken into account when it comes to the case of TSSM, which is not controlled by A. swirskii after the pest outbreak. Using pollen food sources or the sequence of crop infestation by different pest species may be a promising solution for the establishment of predators in order to be prepared against the next pest outbreak. Additionally, the mixed diet can provide sufficient predator–prey ratio by increasing the numerical response of predators, which in turn improves the efficiency of TSSM/GHWF control. Our findings revealed that the creation of pest species diversity in crops could benefit the crops. This issue is quite incomprehensible and risky for farmers; however, it is accepted in some cases as a ‘pest-in-first’ strategy for enhancing the performance of P. persimilis (Messelink et al., Reference Messelink, Van Maanen, Van Holstein-Saj, Sabelis and Janssen2010). Therefore, the use of other alternative food sources such as pollen along with target pest or preventing the eradication of all the pest species in the crops to make a mixed diet and maintaining the predator population in the absence of pests can be a better practical approach and more agreeable to farmers.
Acknowledgements
The financial and technical support of this research by the Department of Entomology, Tarbiat Modares University is greatly appreciated.