Introduction
Habitat manipulation is a common strategy for enhancing multiple ecosystem services in agro-ecosystems, such as pollination and biological pest control (Gurr et al., Reference Gurr, Wratten, Landis and You2017). The targeted introduction of flowering plants into agro-ecosystems is usually recommended to boost functional biodiversity in the field (Uyttenbroeck et al., Reference Uyttenbroeck, Hatt, Paul, Boeraeve, Piqueray, Francis, Danthine, Frederich, Dufrene, Bodson and Monty2016; Campbell et al., Reference Campbell, Wilby, Sutton and Wäckers2017), e.g. by providing nectar and pollen resources, shelter, overwintering sites and alternative prey for beneficial arthropods (Wyss, Reference Wyss1995; Pfiffner and Wyss, Reference Pfiffner, Wyss, Gurr, Wratten and Altieri2004; van Rijn and Wäckers, Reference van Rijn and Wäckers2016; Daniel et al., Reference Daniel, Matray, Stoeckli, Niggli, Vacante and Kreiter2018). Enriching the ground vegetation in farmlands with flowering plants can contribute to higher abundances of beneficial arthropods, resulting in reduced pest infestation and less crop damage (e.g. Tschumi et al., Reference Tschumi, Albrecht, Entling and Jacot2015). This strategy is highly relevant for perennial crop systems such as apple orchards (Simon et al., Reference Simon, Bouvier, Debras and Sauphanor2010; Herz et al., Reference Herz, Cahenzli, Penvern, Pfiffner, Tasin and Sigsgaard2019), where permanent flower strips or structures can be implemented rather easily in the management practice (Cahenzli et al., Reference Cahenzli, Sigsgaard, Daniel, Herz, Jamar, Kelderer, Kramer Jacobsen, Kruczyńska, Matray, Porcel, Sekrecka, Świergiel, Tasin, Telfser and Pfiffner2019; Pfiffner et al., Reference Pfiffner, Cahenzli, Steinemann, Jamar, Chor Bjørnc, Porcel, Tasin, Telfser, Kelderer, Lisek and Sigsgaard2019). In general, farmers favour supporting natural enemies for better aphid regulation in apple orchards (Penvern et al., Reference Penvern, Fernique, Cardona, Herz, Ahrenfeldt, Dufils, Jamar, Korsgaard, Kruczyńska, Matray, Ozolina-Pole, Porcel, Ralle, Steinemann, Świergiel, Tasin, Telfser, Warlop and Sigsgaard2019).
The design of an insect-friendly herb layer in a particular crop system mainly depends on key pests and their targeted antagonists as well as their complex interactions in the arthropod community present (Simon et al., Reference Simon, Bouvier, Debras and Sauphanor2010). Insectary plants must be attractive and exploitable for natural enemies without promoting pests (‘tailored flower strips’) (Campbell et al., Reference Campbell, Wilby, Sutton and Wäckers2017; Gurr et al., Reference Gurr, Wratten, Landis and You2017). Thus, a ‘multitrophic approach’ in flower selection prior to habitat introduction is necessary to enhance functional biodiversity (Baggen and Gurr, Reference Baggen and Gurr1998; Lavandero et al., Reference Lavandero, Wratten, Didham and Gurr2006; Winkler et al., Reference Winkler, Wäckers, Kaufman, Larraz and van Lenteren2009a, Reference Winkler, Wäckers and Pinto2009b, Reference Winkler, Wäckers, Termorshuizen and van Lenteren2010; Géneau et al., Reference Géneau, Wäckers, Luka, Daniel and Balmer2012). The screening process for suitable plants for the nutrition of beneficial arthropods is based on floral architecture, pollen and nectar accessibility and exploitability, including nectar composition and concentration as well as insects’ mouthpart morphology (Baker and Baker, Reference Baker, Baker, Jones and Little1983; Boggs, Reference Boggs, Slansky and Rodriguez1987; Wäckers et al., Reference Wäckers, Björnsen and Dorn1996; Winkler et al., Reference Winkler, Wäckers, Kaufman, Larraz and van Lenteren2009a). Floral nectar mainly consists of three sugar components, i.e. sucrose, glucose and fructose and is secreted in various concentrations from exposed or more concealed nectaries. It serves as an energy resource essential for the fitness of most beneficial insects and thus for their body maintenance, mobility, reproduction and egg maturation (e.g. Wäckers, Reference Wäckers2004; Wäckers and van Rijn, Reference Wäckers, van Rijn, Wäckers, van Rijn and Bruin2005; Irvin et al., Reference Irvin, Scarratt, Wratten, Frampton, Chapman and Tylianakis2006; Lavandero et al., Reference Lavandero, Wratten, Didham and Gurr2006; Winkler et al., Reference Winkler, Wäckers, Kaufman, Larraz and van Lenteren2009a). On the other hand, adult lepidopteran pests may also benefit from nectar (e.g. Baggen and Gurr, Reference Baggen and Gurr1998; Irvin et al., Reference Irvin, Scarratt, Wratten, Frampton, Chapman and Tylianakis2006; Wäckers et al., Reference Wäckers, Romeis and van Rijn2007; Winkler et al., Reference Winkler, Wäckers, Termorshuizen and van Lenteren2010; Sigsgaard et al., Reference Sigsgaard, Betzer, Naulin, Eilenberg, Enkegaard, Kristensen and Michaud2013). Several laboratory studies on nutritional requirements of lepidopteran pests confirmed an enhanced adult fitness by the provision of sugars (Wäckers et al., Reference Wäckers, Romeis and van Rijn2007). Moths showed elongated lifespans, when offered carbohydrate-rich food (Savopoulou-Soultani et al., Reference Savopoulou-Soultani, Milonas and Stavridis1998; Stevens et al., Reference Stevens, Froud and Jamieson2002; Balzan and Wäckers, Reference Balzan and Wäckers2013). Savopoulou-Soultani et al. (Reference Savopoulou-Soultani, Milonas and Stavridis1998), Stevens et al. (Reference Stevens, Froud and Jamieson2002) and Atanassov and Shearer (Reference Atanassov and Shearer2005) also recorded higher fecundity of tortricid moths, provided with sugar solution, honey solution or extrafloral nectar.
The codling moth Cydia pomonella L. (Lepidoptera, Tortricidae) is a major pest in apple, pear and walnut orchards all over the world (Barnes, Reference Barnes, van der Geest and Evenhuis1991; Beers and Brunner, Reference Beers and Brunner1992; Mills, Reference Mills2005; Witzgall et al., Reference Witzgall, Stelinski, Gut and Thomson2008; Voudouris et al., Reference Voudouris, Sauphanor, Franck, Reyes, Mamuris, Tsitsipis, Vontas and Margaritopoulos2011; Blomefield and Giliomee, Reference Blomefield and Giliomee2012), causing serious economic loss, especially in organic apple production. While the biology of codling moth is generally well understood, it has so far not been investigated, whether adult codling moth may benefit from nectariferous plants in the orchard. Howell (Reference Howell1981) and Wenninger and Landolt (Reference Wenninger and Landolt2011) reported that provision of sucrose solution resulted in longer lifespan, but remarkably without affecting moths’ fecundity and fertility. Since there had been little research on flower feeding by adult C. pomonella and the resulting effect on their fitness, we examined the impact of various floral diets on longevity, fecundity and fertility of this pest insect in laboratory trials. We chose flowering plants (Coriandrum sativum L., Daucus carota L., Pastinca sativa L. and Trifolium pratense L.) that had been shown to be beneficial for natural enemies and pollinators (e.g. Stephens et al., Reference Stephens, France, Wratten and Frampton1998; Ambrosino et al., Reference Ambrosino, Luna, Jepson and Wratten2006; Vattala et al., Reference Vattala, Wratten, Phillips and Wäckers2006; Winkler et al., Reference Winkler, Wäckers, Kaufman, Larraz and van Lenteren2009a; Campbell et al., Reference Campbell, Wilby, Sutton and Wäckers2017; Foti et al., Reference Foti, Peri, Wajnberg, Colazza and Rostás2019; Herz et al., Reference Herz, Cahenzli, Penvern, Pfiffner, Tasin and Sigsgaard2019; Jado et al., Reference Jado, Araj, Abu-Irmaileh, Shields and Wratten2019). This kind of screening and resulting exclusion of plants with undesirable positive effects on codling moth from seed mixtures can help to tailor flower strips for conservation biological control in apple orchard management.
Materials and methods
Insects and plants
Codling moths for experiments were taken from a non-diapausing laboratory strain, reared on a semi-artificial diet according to Bathon (Reference Bathon1981). Five males and five females were killed with ethyl acetate to examine proboscis length. After storing the insects in 90% lactic acid solution for approximately 60 h to soften tissues, the proboscis was carefully pulled out of the caput and embedded in Hoyer's Medium (D. Henshaw, Entomological Equipment, UK), using needles to fix the unrolled proboscis. Afterwards, microphotographs were taken under the stereomicroscope (Zeiss, Stemi 508) and measurement of the whole proboscis length was made from these pictures by using the software Zen-Lite (blue edition) (Zeiss Deutschland). Plants were selected based on flower morphology as well as on their proven suitability for beneficial insects. Except for T. pratense, all plant species used are categorized as ‘open-nectar plants’ (Klotz et al., Reference Klotz, Kühn, Durka and Briemle2002; Campbell et al., Reference Campbell, Wilby, Sutton and Wäckers2017) with exposed nectaries, hence probably accessible for C. pomonella. The Fabaceaen plant T. pratense served as a negative control, since the concealed nectar glands in its flowers are not within reach of the moth proboscis (table 1). Seeds supplied by ‘Appels Wilde Samen GmbH ©’, Darmstadt, Germany, were sown in potting soil (Archut, Fruhstorfer Erde®, Typ P) and grown in the greenhouse under standard conditions. In addition, parsnip was cultivated on an adjacent experimental field and flower heads were collected from there.
Table 1. Plant species used in the experiments, characterized by flower morphology and nectar production according to Klotz et al. (Reference Klotz, Kühn, Durka and Briemle2002)

The reported sugar concentration of nectar was determined in the present study.
We measured the sucrose equivalent of floral nectar by use of a handheld refractometer (Bellingham & Stanley Eclipse 45-82, Bellingham + Stanley Ltd., Kent, UK). Four samples with 15 single flowers each were processed at 20 °C for each plant species. Nectar was taken via microcapillaries (Ringcaps© Hirschmann) directly from the prepared nectar glands from flowers freshly taken from the greenhouse or field. In case of D. carota, it was necessary to transfer a sample of flowers into a 2 ml Eppendorf tube followed by centrifugation at 14,000 rpm for 60 s to obtain a minimum nectar volume (3 μl) for measurement.
Experimental design
We conducted cage experiments in a climate chamber (Percival CLF Plant Climatics, Model: I-36VLC8) at 23 °C, with 75% RH and a photoperiod of 16:8 (L:D) to determine lifespan, realized fecundity and fertility of adult codling moth under different floral diet treatments. Pupae were taken from the rearing strain, sexed under the stereomicroscope (Zeiss, Stemi 508) and weighed on a precision balance (Ohaus, Pioneer, PA214C). Each pupa was held separately in an individual medical cup up to the date of emergence. After emergence, a pair of moths (<24 h old) were transferred into white plastic cups (Polarcup©, with 500 ml volume and height of 9.5 cm) covered with cotton gauze and fixed with an elastic, according to Wenninger and Landolt (Reference Wenninger and Landolt2011) and Winkler et al. (Reference Winkler, Wäckers, Kaufman, Larraz and van Lenteren2009a). Each cup contained a water dispenser and one to three nectariferous inflorescences of D. carota, P. sativa, C. sativum or T. pratense, maintained in water vessels. Trifolium pratense was considered as a negative control because its nectar is probably not accessible for the codling moth's proboscis according to our measurements. Sucrose solution (25%, w/w) served as a positive control. A small piece of filter paper in each cup absorbed excess moisture released by the plant parts. New flowers were provided at least every 2 days to refresh nectar supply. Survival of moths was checked daily or occasionally every second day and dead individuals were removed and weighed. Once a week, moths were transferred into new cups and deposited eggs in the old vial were counted. These egg-bearing cups were incubated for further 7 days at 23 °C to enable larval hatching. During this period, cups were checked regularly; all hatched larvae were counted and subsequently removed. The experiments were conducted with at least 20 replicates per treatment for two consecutive years.
Data analysis
All statistical analyses were performed with the software R (R Core Team, 2018, version 3.5.1). Moths that escaped or died accidentally during the experiment were excluded from the analysis. Survival probabilities of codling moth in various treatments were determined by Kaplan–Meier estimates and survival curves were drawn for each treatment (Kaplan and Meier, Reference Kaplan and Meier1958), using the R packages ‘survival’ and ‘survminer’. Results obtained in the two experimental periods were pooled for further analysis, because laboratory conditions and insect/plant material were similar. The number of days survived was fitted as a function of diet and sex, using the surv and survfit link (survfit(surv(longevity) ~ diet + sex)). A Log-Rank statistic was calculated and pairwise post-hoc comparison tests, adjusted with Bonferroni correction, were performed, using the functions survdiff and pairwise_survdiff, respectively. Generalized Linear Models (GLMs) for count data were applied to assess the effects of different diets on realized lifetime fecundity and egg fertility. The total number of eggs or larvae per female ( = fecundity or fertility) was modelled as a function of diet and, in case of fecundity, also of pupal weight. Negative binomial distributions were adapted due to overdispersion of data. The Akaike information criterion was considered as reference for model selection and residuals were inspected visually. Likelihood-Ratio-Tests (LRT) following model simplification tested the significance of explanatory variables. Post-hoc multicomparison tests (Tukey method) were applied to detect differences between the diet treatments. In addition, a series of GLMs with Poisson or γ distribution analysed the influence of pupae weight on moth longevity and fecundity under different diet regimes, as well as the impact of adult nutrition on moth body weight, following the procedure of model selection as described above. Adult food indices (AFIs), according to Wäckers et al. (Reference Wäckers, Romeis and van Rijn2007) and Balzan and Wäckers (Reference Balzan and Wäckers2013), were determined to evaluate the effects of diet on general fitness of codling moth. AFIs were calculated as the ratio between average survival time in days or average total sum of eggs per individual at various diets divided by the respective values at the baseline treatment with T. pratense (negative control).
Results
Accessibility of flowers for codling moth
All included plants produced highly viscous nectar with sugar concentrations from 61 to 71% (table 1) in comparison to the 25% sucrose solution (positive control). Except for T. pratense, all other plants offered nectar in open disk flowers (table 1). Male moths had a significant longer proboscis than females (male: mean (±standard error (SE)): 978 μm (±12.6 SE), female: 938 μm (±12.1 SE), t = 2.314 with 8 degrees of freedom (df), P = 0.0494). The nectar-bearing region of the 12–15 mm long tubular-shaped Fabacean flower of T. pratense was therefore out of reach for codling moth males and females, while the exposed nectaries of the three Apiaceae (C. sativum, D. carota, P. sativa) were well accessible.
Impact of floral diet on lifespan of codling moth
Survival probability of females and males was significantly influenced by diet and sex (χ2 = 228, df = 9, P ≤ 2 × 10−16) (figs 1 and 2). In general, male moths lived longer than female C. pomonella, regardless of the dietary treatment. Both sexes lived longest under the sucrose treatment with a mean of 29.00 (±1.17 SE) days for female and 31.36 (±0.92 SE) days for male moths. In addition, the provision of P. sativa flowers resulted in an extended lifespan for both sexes (female: 19.90 days (±1.68 SE), male: 28.35 days (±1.56 SE)) in comparison to the other tested plant species. Moths offered flowers of C. sativum showed a slightly prolonged survival time (female: 16.71 days (±0.96 SE), male: 25.29 days (±1.03 SE)). Floral nectar of D. carota had little effect on moth longevity (female: 14.70 days (±0.64 SE), male: 22.75 days (±0.97 SE)) and did not differ statistically from the T. pratense (control) treatment (female: 12.29 days (±0.55 SE), male: 19.57 days (±1.17 SE)). The pupal weight of moth was highly influenced by sex: male pupae (42.25 mg (±0.48 SE) weighed less than female pupae (54.11 mg (±0.67 SE), t = 14.55, P < 2 × 10−16). Survival time of males increased with pupal weight and was also influenced by diet (pupal weight: LRT = 10.370, df = 1, P = 0.001281, diet: LRT = 85.090, df = 4, P < 2 × 10−16), but the interaction was not significant. Longevity of female moths was independent of pupal weight (LRT = 0.06, df = 1, P = 0.8065) and only affected by dietary treatments (LRT = 133.79, df = 4, P < 2 × 10−16). Adult moths’ body weight was mainly linked to sex, since male moths were significantly lighter than females (t = 7.431, P < 3.06 × 10−12), regardless of the dietary treatment. Moth body weight at the time of death decreased with increasing age except for individuals treated with sucrose solution.

Figure 1. Survival of C. pomonella females treated with flowers of C.s. = Coriandrum sativum, D.c. = Daucus carota, P.s. = Pastinaca sativa, T.p. = Trifolium pratense or S = sucrose solution, N = 20–21 per treatment.
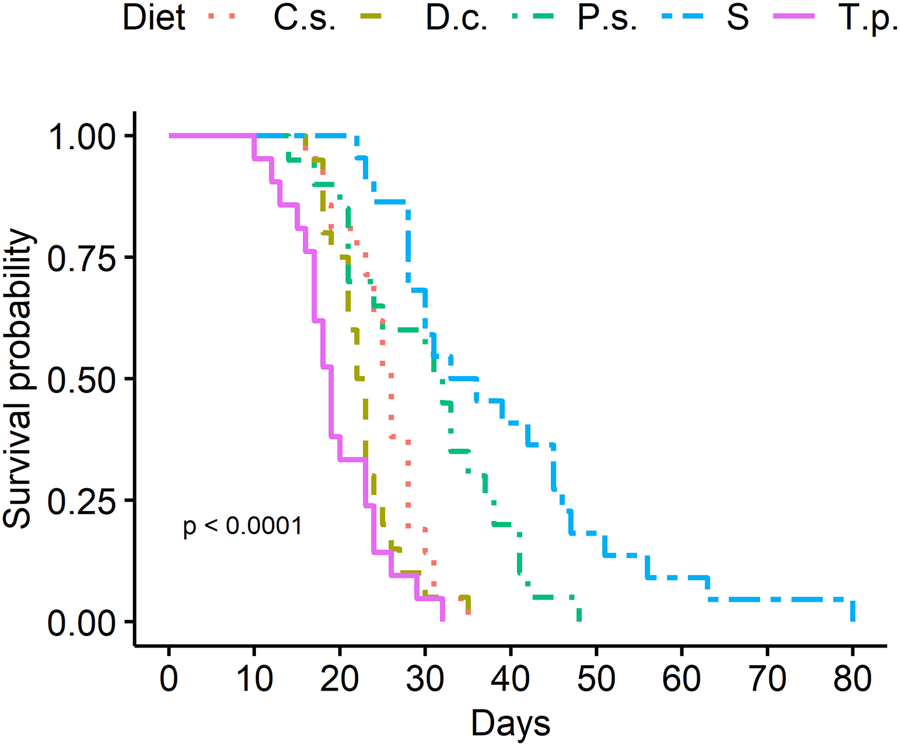
Figure 2. Survival of C. pomonella males treated with flowers of C.s. = Coriandrum sativum, D.c. = Daucus carota, P.s. = Pastinaca sativa, T.p. = Trifolium pratense or S = sucrose solution, N = 20–21 per treatment.
Impact of floral diet on reproduction of codling moth
Lifetime fecundity of female C. pomonella increased with pupal weight (LRT = 18.269, df = 1, P = 1.918 × 10−5) and was also affected by diet to some extent (LRT = 13.849, P ≤ 0.0077), though the interaction between diet and pupal weight was not significant (LRT = 7.4068, df = 4, P = 0.1159). Although females treated with T. pratense laid on average the lowest number of eggs (142.86 (±13.71 SE)) in comparison to females provided with the nectar of C. sativum (161.62 (±20.27 SE)), D. carota (163.55 (±13.07 SE)) and P. sativa (170.95 (±17.24 SE)), these differences were not significant due to high variation between individuals (fig. 3). Adults fed with sucrose laid significantly more eggs on average (215.05 (±18.53 SE)) in comparison to T. pratense (z-value = 3.316, P = 0.0081) and C. sativum (z-value = 2.791, P = 0.0420), but not with respect to the other floral diets. In general, more than half of the total egg load was laid within the first week after emergence in all treatments. Females supplied with T. pratense laid 89% of all their eggs within the first week, followed by D. carota (81%) and C. sativum-fed (72%) moths. Adults provided with P. sativa and sucrose solution laid 62 or 57% of the total amount within the first week, respectively.
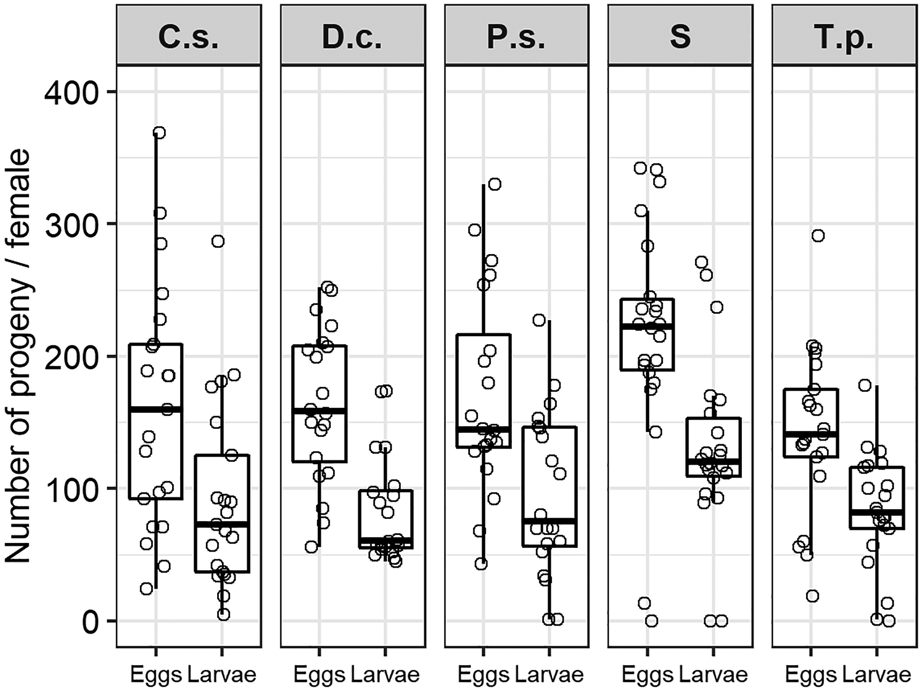
Figure 3. Realized lifetime fecundity and fertility of C. pomonella treated with flowers of C.s. = Coriandrum sativum, D.c. = Daucus carota, P.s. = Pastinaca sativa, T.p. = Trifolium pratense or S = sucrose solution. There is no statistical difference between diet treatments regarding the number of larvae hatched.
Lifetime fertility of C. pomonella was highly correlated to the number of eggs laid per female (r = 0.89). The total number of hatched larvae per female was not significantly affected by adult diet treatments (LRT = 5.2484, df = 4, P = 0.2627) (fig. 3). Hatching rate ranged from 51% in the D. carota-treatment up to 61% in the sucrose-treatment. Hatching rate was nearly identical in P. sativa (56%) and C. sativum-treatments (57%) and quite similar to both negative and positive control (T. pratense: 58% vs. sucrose solution: 61%).
Impact of adult diet on fitness of codling moth
Codling moth males and females are not able to feed nectar from T. pratense flowers and their longevity and fecundity at this treatment served as a baseline indicator for their fitness (AFI = 1, table 2). Although having access to exposed nectaries of C. sativum and D. carota, fecundity of C. pomonella increased only slightly by less than 15% (AFFI 1.13–1.14, table 2). In contrast, fecundity increased by 50% (table 2) when having access ad libitum to sucrose solution (25%) and survival time of both sexes doubled. Pastinca sativa enhanced lifespan (like C. sativum), but affected the number of laid eggs less notably (AFFI 1.20).
Table 2. Adult food indices (AFI) according to Wäckers et al. (Reference Wäckers, Romeis and van Rijn2007) and Balzan and Wäckers (Reference Balzan and Wäckers2013)

The AFI-value is calculated by the ratio of longevity (days) or fecundity (number of eggs/female) of C. pomonella females (F) and males (M) exposed to nectar accessible plants or sucrose solution (25%) compared to the control based on T. pratense (AFI = 1).
Discussion
We examined the impact of floral diet on the adult fitness of C. pomonella as a key pest in apple production. The short proboscis of C. pomonella did not reach the hidden nectar in zygomorph flowers of T. pratense, but the moths were able to feed from open disk flowers. Nectar intake from the umbellifers C. sativum and especially P. sativa significantly increased the survival of male and female C. pomonella, but affected reproduction (lifetime fecundity or fertility) much less. These plants produce a nectar rich in sugar (60–70%) with high viscosity, which makes it difficult for moths to absorb (May, Reference May1985). Therefore, the ingestible amount of nectar may have been limited, whereas the fluid 25% sucrose solution was easily consumed, even providing ad libitum as diet surplus for the insects. In general, nectar viscosity is a function of sugar concentration and also humidity (van Rijn and Wäckers, Reference van Rijn and Wäckers2016), especially when secreted from exposed nectaries. Winkler et al. (Reference Winkler, Wäckers, Kaufman, Larraz and van Lenteren2009a) reported that Pieris rapae L. was able to imbibe nectar from several plants at 90% rel. humidity, but not when plants were provided at 45% rel. humidity. The conditions in our experiment ensured a rel. humidity of 75%, which prevented rapid water loss in the nectar. However, we cannot rule out the possibility that the use of cut inflorescences or the renewal of flower heads every 2 days may have restricted the nectar supply of these plants (in particular D. carota and C. sativum) more than under natural conditions. For example, in a study by Wade and Wratten (Reference Wade and Wratten2007), the life expectancy of a parasitoid was shorter when cut rather than intact coriander flowers were used. We also observed that individual nectar droplets in single florets of P. sativa were larger than those in flowers of C. sativum or D. carota. Thus, the volume of nectar droplets secreted can also determine whether certain flowers are more profitable to moths than others. In addition, nectar volume and quality will be influenced by plant growing conditions, microclimate, daily secretion rate, and other external and internal factors (Corbet, Reference Corbet2003) that were not considered in our study. Moth survival time was highest by provision of the sugar solution and individuals even tended to gain body weight. Howell (Reference Howell1981) and Wenninger and Landolt (Reference Wenninger and Landolt2011) described similar results and assumed that C. pomonella is generally attracted by sweet food sources. Male moths in particular benefited from sugar sources (Cisneros and Barnes, Reference Cisneros and Barnes1974; Schumacher et al., Reference Schumacher, Weyeneth, Weber and Dorn1997; Wenninger and Landolt, Reference Wenninger and Landolt2011). An increased energy level favours further mobility and dispersal (Irvin et al., Reference Irvin, Scarratt, Wratten, Frampton, Chapman and Tylianakis2006; Wenninger and Landolt, Reference Wenninger and Landolt2011), which results in more mating opportunities for the males, increasing populations and benefits for upcoming generations (Howell, Reference Howell1981; Wenninger and Landolt, Reference Wenninger and Landolt2011). In female moths, access to 25% sucrose solution led to the longest lifespan (double AFLI) and also the highest number of offspring (50% more eggs, indicated by AFFI), while floral nectar had no distinctive effect on lifetime fecundity and fertility. Due to highly variable egg load (fig. 3), which is obviously typical for this species (Geier and Briese, Reference Geier and Briese1978) and which may more depend on nutrients acquired during larval development, no statistical differences occurred between floral treatments. In an early study, Howell (Reference Howell1981) reported that, when codling moth fed on various carbohydrate solutions in the laboratory, diet did not affect fecundity or hatching rate of eggs, but increased longevity. Howell (Reference Howell1981) and other researchers (e.g. Geier, Reference Geier1963; Deseö, Reference Deseö1971; Hagley, Reference Hagley1972; Savopoulou-Soultani et al., Reference Savopoulou-Soultani, Milonas and Stavridis1998) also found that the main oviposition of C. pomonella and other tortricid pests occurred in the early stage of moth life. An analogous oviposition pattern was observed in our study and the absolute number of eggs laid in the first week was quite similar in all treatments (coriander: 2431, carrot: 2649, parsnip: 2115, sucrose: 2700, red clover: 2672 eggs in total). Consequently, a prolonged lifespan does not necessarily result in a significant higher lifetime fecundity of codling moth, supporting the assumption that adult C. pomonella do not need additional food to reproduce adequately, as stated by Geier (Reference Geier1963), Howell (Reference Howell1981) and Benz (Reference Benz, van der Geest and Evenhuis1991). Nevertheless, the rate of egg deposition within the first week differed between the various dietary treatments and the oviposition period was longer than 3 weeks when parsnip flowers or sucrose were offered. Atanassov and Shearer (Reference Atanassov and Shearer2005) also observed an extended egg deposition period of Grapholita molesta Busck supplied with accessible extrafloral nectar and Boggs (Reference Boggs1997) and Cahenzli and Erhardt (Reference Cahenzli and Erhardt2012) found a similar pattern in some butterfly species as well. Perhaps the moths perceive suitable food sources and are able to adapt their oviposition pattern to the availability of food sources in the surroundings. An adequate or even over supply of carbohydrates could also prevent the exploitation of other body reserves and allow their use for further egg maturation. However, adequate nutrition may be more relevant to other life history traits than reproduction or longevity: under natural conditions, mobility and dispersal are also important for females to find suitable host plants (Gu et al., Reference Gu, Hughes and Dorn2006), and regular sugar intake may be even more important for maintaining these functions.
Instead, lifetime fecundity of female codling moth was clearly influenced by pupal weight, since the amount of deposited eggs was higher for moths emerging from heavier pupae. Geier (Reference Geier1963), Deseö (Reference Deseö1971) and Cisneros and Barnes (Reference Cisneros and Barnes1974) have already described a positive correlation between pupal weight and fecundity. Pupal weight is caused by a combination of intrinsic and extrinsic factors, such as genetic disposition, sex, climate conditions, diapausing and larval diet (Geier, Reference Geier1963; Cisneros and Barnes, Reference Cisneros and Barnes1974; Boggs, Reference Boggs1997). Since the quality and quantity of larval food certainly has a major influence on pupal weight and thus on the fecundity of the moths, we cannot rule out the possibility that artificially reared moths show different nutritional requirements or physiological characteristics than natural populations.
The number of hatched larvae in this study depended strongly on the number of deposited eggs, but not on diet, confirming the results of Wenninger and Landolt (Reference Wenninger and Landolt2011), who found no differences in total egg fertility in their feeding experiments with C. pomonella. We observed no decreasing fertility of eggs with increasing female age in contrast to results from Deseö (Reference Deseö1971) and Hagley (Reference Hagley1972), and hatching rate was not different between diet treatments.
The increase of pest fitness due to the presence of suitable floral resources in the field depends on a complex of biotic and abiotic factors, as e.g. the occurring plant species and composition, orchard management, climate conditions, abundance of antagonists, as well as feeding behaviour of the key pest (Simon et al., Reference Simon, Bouvier, Debras and Sauphanor2010). Although adult C. pomonella successfully consumed nectar from all open disk flowers in our study, it has to be clarified if moths actively forage and benefit from floral nectar in nature. Codling moths generally mate and oviposit at night. Whether they disperse from tree to tree or also occur in ground vegetation to visit flowers during the day for nectar consumption is not known. Other potential food sources may be more important and may come from aphid honeydew, extrafloral nectaries on host plants, tree sap or even fruit debris available in the canopy.
According to calculated AFFI and AFLI in our trials, T. pratense, D. carota, C. sativum and somewhat less so, P. sativa, seemed to be appropriate ‘low-risk’ candidates for field use. However, further studies involving single and mixed flowering plants in apple orchards will be necessary to investigate the feeding behaviour of codling moth and its main natural enemies such as parasitoids. Various field studies on the effects of flower strips for biocontrol in apple orchards did not yield consistent results (Herz et al., Reference Herz, Cahenzli, Penvern, Pfiffner, Tasin and Sigsgaard2019). For instance, less fruit damage caused by codling moth was observed in plots with flowering plants by Altieri and Schmidt (Reference Altieri and Schmidt1985), as well as recently by Cahenzli et al. (Reference Cahenzli, Sigsgaard, Daniel, Herz, Jamar, Kelderer, Kramer Jacobsen, Kruczyńska, Matray, Porcel, Sekrecka, Świergiel, Tasin, Telfser and Pfiffner2019), contrary to the findings of Brown (Reference Brown2001), who reported an increase of damaged apples. In addition to testing further nectaferious plant species, other sugar sources occurring in the orchard, such as honeydew or apple flesh, may be taken into account, since Wiesmann (Reference Wiesmann1935) observed that adult C. pomonella fed on aphid honeydew in the field. It is likely that moths are able to utilize sugar components of honeydew (Baker and Baker, Reference Baker, Baker, Jones and Little1983). Perhaps codling moth benefits even more from honeydew available in the apple tree canopy than from floral nectar, similar to the olive moth Prays oleae Bernard, which showed highest longevity and reproduction by provision of insects' honeydew (Villa et al., Reference Villa, Marrão, Mexia, Bento and Pereira2017).
In this study, we found that survival, but not reproduction of adult codling moth increased when having the opportunity to feed on the nectar of several umbellifers. These findings underline the need for a careful selection of plant species in seed mixtures to obtain ‘tailored’ flower strips, favourable only for beneficials and not for pests. Our results may help to improve the concept and success of conservation biological control in apple orchards by cautious management of functional biodiversity.
Acknowledgements
The authors gratefully acknowledge the financial support from the project ‘Innovative design and management to boost functional biodiversity of organic orchards – ECOORCHARD’, CORE Organic Plus (28698), cofinanced by the European Commission as well as by the Federal Ministry of Food and Agriculture with the Federal Programme for Ecological Farming and Other Forms of Sustainable Agriculture (BÖLN) – FKZ: 2814OE005 (Germany). We are indebted to Nils Drexler, who conducted laboratory experiments in 2017, as part of his BSc-Thesis. Also, we would like to express our gratitude to Milena Runge, Stefanie Hemmerling and Simon Feiertag for their help in conducting the experiments and providing plant and insect material. We would like to thank Dr Doreen Gabriel, Julius Kühn Institute, Institute for Crop and Soil Science, Braunschweig for helpful support in statistics.