Introduction
New Zealand remains the only major fruit producing country in the world that is free from economically significant pest fruit flies (Stephenson et al., Reference Stephenson, Gill, Ran and Wilson2003). Monitoring and surveillance programs have been put in place to ensure their early detection and to minimize pathway risk (Stephenson et al., Reference Stephenson, Gill, Ran and Wilson2003; Armstrong & Ball, Reference Armstrong and Ball2005). The ability to identify intercepted organisms to species level is essential for accurate interception data and assessment of pathway risk. Unfortunately, the interceptions at the borders in fruits from commercial consignments or accompanying overseas travellers are usually eggs or larvae, which cannot be identified morphologically beyond the family or genus level.
Bactrocera xanthodes (Broun) is regularly intercepted at the New Zealand border. It is part of a complex of four closely related species that together currently comprise the subgenus Notodacus, and also includes Bactrocera paraxanthodes Drew & Hancock from New Caledonia (Drew et al., Reference Drew, AlIwood, Tau, Allwood and Drew1997), Bactrocera neoxanthodes Drew & Romig from Vanuatu (Allwood et al., Reference Allwood, Tumukon, Tau, Kassim, Pacific, Allwood and Drew1997) and an undescribed new species from Samoa (Heimoana et al., Reference Heimoana, Thnupopo, Toleafoa, Fakanaiki, Allwood and Drew1997). B. xanthodes is present in Fiji, Tonga, Niue, Samoa, American Samoa, Southern part of Cook Islands, and Wallis and Futuna (Plant Health Australia, 2016). It was detected on Nauru in 1992 and Raivavae (French Polynesia) in 1998 but eradicated by male annihilation (Leblanc et al., Reference Leblanc, Vueti, Drew and Allwood2012; Plant Health Australia, 2016). B. paraxanthodes was initially described from New Caledonia, Vanuatu and Western Samoa (Drew & Hancock, Reference Drew and Hancock1995). Later data indicated that it is only present in New Caledonia (Drew et al., Reference Drew, AlIwood, Tau, Allwood and Drew1997). B. neoxanthodes (in Drew et al., Reference Drew, AlIwood, Tau, Allwood and Drew1997 as B. sp. n. No. 2) is only present in Vanuatu (Drew et al., Reference Drew, AlIwood, Tau, Allwood and Drew1997; Heimoana et al., Reference Heimoana, Thnupopo, Toleafoa, Fakanaiki, Allwood and Drew1997; Drew & Romig, Reference Drew and Romig2001). To date, no detailed studies on the undescribed species from Samoa have been conducted.
Bactrocera xanthodes is a major fruit fly pest throughout the South Pacific region, where they infest a wide range of commercial fruit plants (Hoeben et al., Reference Hoeben, Daniel, Ma and Drew1996). The hosts of B. xanthodes have been recorded from 20 plant families, including Anacardiaceae, Annonaceae, Apocynaceae, Caricaceae, Combretaceae, Euphorbiaceae, Lauraceae, Lecythidaceae, Moraceae, Passifloraceae, Rutaceae and Sapotaceae (Leblanc et al., Reference Leblanc, Vueti, Drew and Allwood2012). Host fruit survey showed that the abundant breadfruit, Artocarpus altilis (Moraceae) in the Pacific countries is a major host for B. xanthodes (Tora Vueti et al., Reference Tora Vueti, Allwood, Leweniqila, Ralulu, Balawakula, Malau, Sales, Peleti, Pacific and Drew1997a). In addition, the wild fruits, Ochrosia oppositifolia (Apocynaceae) and Barringtonia edulis (Lecythidaceae) are also the common hosts for B. xanthodes (Tora Vueti et al., Reference Tora Vueti, Ralulu, Walker, Allwood, Leweniqila, Balawakula, Pacific and Drew1997b). In comparison, for the other three species in the complex, non-commercial fruits, i.e. only wild hosts, are recorded as hosts: Schefflera sp. for B. paraxanthodes; Barringtonia edulis (Lecythidaceae) and Passiflora suberosa (Passifloraceae) for B. neoxanthodes (Drew & Romig, Reference Drew and Romig2001) and Ficus sp. (Araliaceae) for the undescribed species, B. sp. n. No. 1 (Drew et al., Reference Drew, AlIwood, Tau, Allwood and Drew1997; Leblanc et al., Reference Leblanc, Vueti, Drew and Allwood2012).
Molecular diagnostic techniques, such as polymerase chain reacation-restriction fragment length polymorphism (PCR–RFLP) (Armstrong et al., Reference Armstrong, Cameron and Frampton1997) and DNA barcoding have been used for fruit fly identification (Hebert et al., Reference Hebert, Ratnasingham and deWaard2003; Armstrong & Ball, Reference Armstrong and Ball2005). More rapid identification has been achieved by real-time PCR assays, several of which have been developed for fruit fly species, i.e. Bactrocera tryoni complex (including Bactrocera tryoni, Bactrocera aquilionis and Bactrocera neohumeralis), Bactrocera invadens, Ceratitis capitata and Dirioxa pornia (Dhami et al., Reference Dhami, Gunawardana, Voice and Kumarasinghe2016) and are used in the routine diagnostics at New Zealand's borders. However, there is no reported real-time PCR protocol for B. xanthodes, which is common interceptions at New Zealand borders, therefore, there is the need to develop a real-time PCR assay for rapid detection of the common border interceptions. Here we report the DNA barcoding data for B. xanthodes, B. paraxanthodes and B. neoxanthodes, and also the development and validation of the real-time PCR protocols targeting the B. xanthodes species complex, including their sensitivity, specificity and blind panel test. The assay for B. xanthodes has been tested on the interceptions at New Zealand borders, and provided accurate and rapid identification of the intercepted eggs and larvae from the Pacific countries.
Materials and methods
Sampling
Eggs and larval fruit fly specimens used in this study were stored at −20°C freezer or 96% ethanol prior to DNA extraction (table 1). These specimens were intercepted at New Zealand borders from various countries. In addition, dry mounted adult specimens of B. neoxanthodes from Vanuatu, and adults of B. paraxanthodes in 96% ethanol from New Caledonia were obtained from their country collections (tables 1 and 2). Identifications of the adult specimens were done by morphological characters and confirmed by Professor Dick Drew from Griffith University, Australia.
Table 1. Information of the target species tested in the real-time PCR assays.

1 DNA extraction method of Qiagen Blood and Tissue kit (QC), PrepGEM (PG) and Chelex 100 (CH) were used for the samples.
2 The DNA sequence has 99% identities with B. neoxanthodes from Vanuatu, ~89% to B. xanthodes of other locations obtained from this study.
3 Only two larvae were intercepted from watermelon (Citrullus lanatus), no breeding population were observed, thus whether the watermelon is the original host needs to be further confirmed.
Table 2. Information of the non-target species tested in the real-time PCR assays.

DNA extraction and barcoding
Total DNA from individual specimens was extracted using the DNeasy for Blood and Tissue kit (Qiagen, Valencia, CA, USA) as per the manufacturer's instructions. An adult-leg, piece of larva or egg was used for each extraction and physical disruption was performed by micro-pestles, with DNA eluted in 100 µl of warmed AE buffer. For some samples, DNA was also extracted using crude extraction methods: 6% Chelex 100 (Sigma- Aldrich Co. Aldrich Co.) and prepGem DNA kit (ZyGem Corporation Ltd., Hamilton, New Zealand).
All the DNA extracted was used for PCR amplification with primers, LCO1490 and HCO2198 (Folmer et al., Reference Folmer, Black, Hoeh, Lutz and Vrijenhoek1994). For all the PCR reactions, each 20 µl reaction consisted of 1 × GoTaq master mix (Promega, Madison, WI), 250 nM of each primer, 0.5 µg µl−1 Bovine Serum Albumin (BSA, Sigma-Aldrich Co.), and 2 µl of DNA extract. Cycling conditions were: initial denaturation at 94°C for 2 min, 40 cycles of 94°C for 15 s, 52°C for 30 s and 72°C for 45 s, followed by a final extension step of 7 min at 72°C. However, no amplification was observed with the DNA extracted from the dried aged specimens of B. neoxanthodes. Testing on 18S qPCR assay, positive amplification was observed, indicating that DNA was extracted from the specimens, thus two sets of primers were designed to amplify 200–300 bp PCR products each targeting different regions of the COI sequences (DQ116354-DQ116356): BNX211F: TACCTCCTTCCCTGACCCTG; BNX345R: GTGAGAAAATTGCAAGATCAACGG; BNX345F: GCATCCGTTGATCTTGCAA and BNX_607R: TCAAAATAGGTGCTGATAAAGAATAGG. The same PCR compositions and cycling conditions as above were applied using the two pairs of primers. All the PCR products and DNA sequences were analyzed as per Li et al. (Reference Li, Fan, Waite, Gunawardana, George and Kumarasinghe2015). The DNA sequences were submitted to BOLD under the project of Barcode of Bactrocera Specimens (BBS) at BOLD and with accession numbers MF742414-MF742457 for B. xanthodes, MF742409-MF742411 for B. neoxanthodes and MF742412-MF742413 for B. paraxanthodes under GenBank.
Real-time PCR design
DNA sequences of COI from B. xanthodes, B. neoxanthodes, B. paraxanthodes and the closely related species were aligned in Geneious 7.1.5 (Biomatters, Auckland, New Zealand). Regions that showed differences specific to B. xanthodes, B. paraxanthodes and B. neoxanthodes were selected manually and used as the target region for the specific probes and primers. The primers and probe were manually designed and their suitability examined in the web-based RealTimeDesign qPCR assay design software from Biosearch. Their secondary structures and thermodynamic properties were checked using Geneious and OligoAnalyzer 3.1 (OligoAnalyzer, 2012). The specificity of the designed primers and probes were also checked in Geneious and BLAST searches in GenBank database (Altschul et al., Reference Altschul, Gish, Miller, Myers and Lipman1990).
Real-time PCR optimization
All real-time PCR reactions were set up on a CFX96™ Touch Real-time platform (BioRad). Optimization were conducted on a temperature gradient of 55–66°C, using primer concentrations (200 and 300 nM), probe gradients (125–300 nM), and with or without the addition of Mg2+ (1.5 mM). To select an appropriate real-time mastermix for the assay, the real-time PCR tests were run with mastermixes: PerfeCTa® qPCR ToughMix® (Quanta Bioscience) and SsoAdvanced™ Universal Probes Supermix (BioRad). The real-time PCR was also tested in singleplex and multiplex formats and with 18S ribosomal RNA (rRNA) gene internal control real-time PCR (Applied Biosystems, CA, USA).
Specificity
The specificity of the real-time PCR assay was tested using the DNA extraction from different Bactrocera species both the target (table 1) and non-target (table 2) species. These samples included B. xanthodes, B. neoxanthodes, B. paraxanthodes, B. passiflorae, B. cucumis, B. dorsalis, B. jarvisi, B. latifrons, B. tryoni and other flies (table 2).
Sensitivity
To evaluate the analytical sensitivity of the developed assay, 710, 708 and 709 bp of the COI gene, respectively for B. xanthodes, B. paraxanthodes and B. neoxanthodes, were used to prepare plasmid standards of known copy numbers. The amplicon was cloned using the TOPO®TA vector cloning kit (Invitrogen, Carlsbad, CA, USA) as per the manufacturer's instructions. Cloning was performed for one DNA sample from each species, and the clones containing the correct insert were selected for preparing the plasmid DNA standards. Plasmid DNA was extracted using the Wizard® Plus SV Miniprep (Promega, Madison, WI, USA). The plasmid DNA was quantified using a μDrop plate in MultiSkan GO DNA quantification system (Thermo Scientific, USA) and normalized to a concentration of 108 copies/ µl−1. A dilution series of the plasmid from 107–100 copies was created using TE buffer (10 mM TriHCl and 1 mM EDTA, Invitrogen). Analytical sensitivity of the assay was determined using the dilution series with each concentration in triplicate per reaction. Linear regression was performed between the detection threshold (Cq) and the log10 of the copy number, measuring the fit as r 2. Amplification efficiencies for individual reactions were calculated using the formula, E = 10|1/slope| and converted to E% by (E-1) × 100 in the R environment version 3.1.1 (R Development Core Team, 2011).
Blind panel test
A total of 26 specimens were provided to the operators with no knowledge of the sample origin and identity (table 4). The samples were tested against the real–time assay in singleplex for B. xanthodes, B. paraxanthodes and B. neoxanthodes, respectively. It was also tested in the multiplex for B. xanthodes, B. paraxanthodes and B. neoxanthodes. All the samples were tested in duplicate wells, positive and non- template controls were included.
Results
DNA barcoding
DNA barcoding of Bactrocera xanthodes and B. paraxanthodes were obtained using Folmer's primers, LCO1490 and HCO2198 (Folmer et al., Reference Folmer, Black, Hoeh, Lutz and Vrijenhoek1994). For B. neoxanthodes, DNA barcoding was achieved by using BNX211F-345R and BNX345F-607R primer pairs which amplified two overlapping regions of the COI gene and resulted in 460 bp sequences. Sequence comparison of the three species showed that B. xanthodes is distant from the other two species of the complex by about 12% while B. neoxanthodes and B. paraxanthodes are more closely related, with around 5% difference in their COI sequences.
Those DNA barcoding sequences are AT-rich, with average 58.8% for B. xanthodes, 60.1% for B. paraxanthodes and 58.3% for B. neoxanthodes. The sequences of B. xanthodes were around 98% similar to each other and to three B. xanthodes sequences in Genbank (DQ116351-DQ226353), but ~88% similar to another three sequences in Genbank, DQ116354-DQ116356 (Armstrong & Ball, Reference Armstrong and Ball2005). The later three sequences from Armstrong & Ball Reference Armstrong and Ball2005, were labelled as B. paraxanthodes in the supplemental figure and Appendix table 3 in Armstrong & Ball's publication (Reference Armstrong and Ball2005) though they are recorded as B. xanthodes in GenBank. Surprisingly, the sequences from B. neoxanthodes obtained in this study, are identical to the above three sequences (DQ116354-DQ116356) in the overlap region of the 458 bp. On the other hand, the sequences of B. paraxanthodes obtained in this study were quite different to those three sequences (DQ116354-DQ116356) by ~12%. Therefore the results suggested that the three sequences (DQ116354-DQ116356) in BOLD and GenBank are more likely derived from B. neoxanthodes, but not B. paraxanthodes or B. xanthodes.
Real-time PCR design
The B. xanthodes COI sequences obtained in this study were used for alignment and design of the real-time PCR assay, and the unreliable sequences were discarded from this analysis. Initially, real-time PCR assay was designed to test whether two SNPs near the 3’-end of the probe binding sites (table 3) could distinguish B. xanthodes and B. neoxanthodes (fig. 1). However, when testing the two probes (Bxand_1P vs. Bxand_V_1P) using the DNA extracted from the two species, amplification curves were observed with similar Cq values for either one of the probes. Further tests also showed that the assay could detect B. paraxanthodes even though there were two SNPs in the probe and one SNP in the reverse primer binding sites, respectively (fig. 1). Therefore, this real-time PCR assay can be used as a generic analysis for the detection of the three described species of the B. xanthodes complex.

Fig. 1. Alignment of the primers and probe regions for the real-time PCR assay targeting the Bactrocera xanthodes, B. neoxanthodes and B. paraxanthodes. The bold black letters indicate the SNPs in the species to the listed primers/probe.
Table 3. The detailed information about the real-time PCR assay for the detection the B. xanthodes species complex and the cycling parameters.

Since B. xanthodes is the most common interception from the B. xanthodes complex at the New Zealand border, it is more applicable if the real-time PCR assay targeted this species specifically. Thus specific probes and primers for each of the species (fig. 2) were designed to distinguish the three species. In the design, at least three SNPs in the probe with additional SNPs in the primers binding sites were selected to maximize the specificity of each assay (fig. 2). The primers and probes for each of the assays and their PCR conditions are listed in table 3.

Fig. 2. Alignment of the primers and probe regions of COI sequences from the Bactrocera xanthodes complex. The alignment listed all three real-time PCR assays, (a) B. xanthodes; (b) B. neoxanthodes and (c) B. paraxanthodes. The bold black letters indicate the SNPs in the species to the listed primers/probe.
Real-time PCR optimization
The real-time PCR assay tested with different temperatures showed that similar Cq values were obtained from the temperatures at 56–62°C. Higher Cq values were obtained for temperatures above 62°C, thus the optimal annealing/extension temperature was chosen as 62°C. The cycling condition is 95°C for 2 min, followed by 40 cycles of 95°C for 15 s and 62°C for 60 s.
The real-time assay was demonstrated to perform consistently well using SsoAdvanced™ Universal Probes Supermix (BioRad) and PerfeCTa® qPCR ToughMix® (Quanta Bioscience). Similar Cq were also obtained for each sample tested although slight intensity increases were observed in the Mastermixes when additional MgCl2 was added. No significant difference in the Cq values were observed when the assay was run in singleplex with different concentrations of primers. To maximize the real-time PCR efficiency for each real-time PCR assay, the recommended concentrations for each primer and probe are listed in table 3 and the assay can be run as a singleplex or as a multiplex formats.
Sensitivity of the real-time PCR assay
The linear dynamic range for the assay was tested on three plasmid DNAs containing the COI inserts and extended from 107 to 100 copies µl−1, respectively. The 95% confidence limits of the linear dynamic range are plotted in fig. 3, with a strong correlation coefficient (r 2 = 0.997–0.998). The limit of detection (LOD) for the assay was estimated to be ten copies of the target DNA. A template concentration of one copy µl−1 was sporadically detected in all the three assays with an average Cq value around 35 cycles. The calibration curves shown in fig. 3 were able to detect 100% of the samples at the ten copies µl−1.

Fig. 3. Sensitivity test of the real-time PCR assay for the identification of the Bactrocera xanthodes complex. Plasmid containing COI insert of B. xanthodes, B. neoxanthodes and B. paraxanthodes were series diluted, respectively and tested in each real-time PCR assay separately. The concentrations of the plasmid DNA and the Cq values were used to create calibration curves for sensitivity calculations. The standard curve built from Cq values against the log copy number (range = 107–100 copies) of COI insert (n = 3). The 95% confidence intervals of the slopes were plotted with a gray line for BX (B. xanthodes), a white line with dots for BNX (B. neoxanthodes) and a black line for BPX (B. paraxanthodes). The r 2 = 0.997 for BNX, 0.998 for BX and BPX were obtained for each assay, respectively.
Specificity of the real-time PCR
All the Tephritidae samples listed in table 1 were successfully amplified by the real-time PCR assays and no amplifications were observed in the non-target species (table 2). The assays were able to accurately identify B. xanthodes, B. neoxanthodes and B. paraxanthodes samples, respectively. No cross-reaction was observed among the three target species and their closely related species, B. passiflorare from Fiji, B. melanotus from the Cook Islands and other Bactrocera species from other Pacific countries (tables 2 and 4). No cross-reaction was observed from other commonly intercepted fruit fly species (tables 2 and 4), including Tephritidae species from genera Bactrocera: B. correcta, B. cucumis, B. dorsalis, B. jarvisi, B. neohumeralis, B. oleae, B. tryoni, B. zonata; Anastrepha: Anastrepha sp. and Anastrepha striata; and Dirioxa: Dirioxa pornia.
Table 4. Sample information used in the blind panel test of the real-time PCR assay for the B. xanthodes complex.
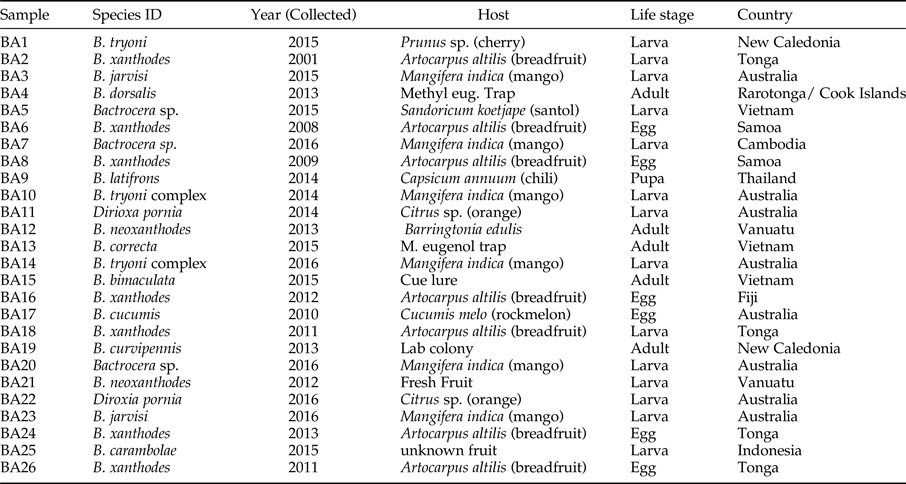
Blind panel test
All the 26 samples in the blind panel test were tested in the real-time PCR protocols. All the target samples were tested positive with Cq values <30. No amplification was observed in all the non-target species (table 4).
Discussion
DNA barcoding of B. xanthodes complex
This study provided essential DNA barcode information for B. xanthodes, B. neoxanthodes and B. paraxanthodes, which will assist in the identification and differentiation of the three species. Up to now, there are only a handful of sequences labelled as B. xanthodes in BOLD and GenBank. However, sequence comparison among these sequences shows around 12% nucleotide difference. Three such ‘B. xanthodes’ sequences in GenBank and BOLD are listed as B. paraxanthodes in the supplemental materials in the publication of Armstrong & Ball (Reference Armstrong and Ball2005) and the specimens were from Vanuatu. This information has raised the suspicion of the true identities of these sequences because B. paraxanthodes is only described from New Caledonia (Drew et al., Reference Drew, AlIwood, Tau, Allwood and Drew1997) but B. neoxanthodes from Vanuatu (Drew & Romig Reference Drew and Romig2001). Besides the divergences of the COI sequences submitted as B. xanthodes, there are errors in some of the submitted B. xanthodes sequences in GenBank. The sequences listed under accession numbers KT864778-KT864780 (Dhami et al., Reference Dhami, Gunawardana, Voice and Kumarasinghe2016) in Genbank are recorded as B. xanthodes, but comprehensive analysis reveals that only KT864778 is B. xanthodes, the other two (KT864779 and KT864780) are sequences of B. passiflorae. On the other hand, KT864761-KT864763 deposited as B. passiflorae shared 100% sequence identities with B. xanthodes (Dhami et al., Reference Dhami, Gunawardana, Voice and Kumarasinghe2016). Since the six sequences were submitted by the same submitters, there is the possibility of mislabelling of the sequences. In addition, sequence KT595001, submitted as B. xanthodes for voucher ms0896 (Leblanc et al., Reference Leblanc, San Jose, Barr and Rubinoff2015), has only ~40% sequence identity to B. xanthodes. The specimen was collected from Rurutu (French Polynesia) (Leblanc et al., Reference Leblanc, San Jose, Barr and Rubinoff2015), which, to our knowledge, has no record of B. xanthodes though it had been detected in Raivavae (French Polynesia) but subsequently eradicated (Leblanc et al., Reference Leblanc, Vueti, Drew and Allwood2012; Plant Health Australia, 2016). A BLAST search of this COI sequence (KT595001) showed that the closet matches are 87% to several Bactrocera species, thus the true identity for this specimen needs to be determined. Therefore, this study provided the reference DNA barcode sequences for B. xanthodes from Fiji, Samoa and Tonga, B. neoxanthodes from Vanuatu and B. paraxanthodes from New Caledonia, which further clarify the COI sequence difference among the three species.
The barcode library submitted to BOLD, including 44 B. xanthodes, 3 B. neoxanthodes and 2 B. paraxanthodes sequences, which further enriched the DNA barcode sequences for the species complex. Most of the B. xanthodes specimens were intercepted from breadfruit except two larvae which were detected in watermelon (Citrullus lanatus) from Tonga (table 1). Watermelon as a host for B. xanthodes was first reported at the New Zealand border in 1985 (Cowley et al., Reference Cowley, Baker, Englberger and Lang1991) and was questioned at the time. Although the two larvae were intercepted from the watermelon recently, whether watermelon is the host needs further investigation. In addition, this study has provided the DNA barcodes for B. paraxanthodes from New Caledonia and B. neoxanthodes species from Vanuatu. Therefore this study extended the DNA barcode capability for the identifications of B. xanthodes species complex. Unfortunately, no specimen/s and further study on the undescribed B. sp. No 1 (Drew et al., Reference Drew, AlIwood, Tau, Allwood and Drew1997) were available, therefore we are unable to provide any further information about this potential species. Further research on B. sp. No 1 (Drew et al., Reference Drew, AlIwood, Tau, Allwood and Drew1997) will be conducted if the specimen/s is available, which will reveal the relatedness of this species in the B. xanthodes complex.
The optimization and application of the real-time PCR assays
There are real-time PCR assays developed for rapid detection of a number of fruit fly species, however, no real-time PCR protocol for B. xanthodes complex have been reported. For the first time, this study developed and validated the real-time TaqMan PCR assays for the detection of the B. xanthodes complex and for the each of the three described species of the complex. Real-time PCR assays for the detection of B. xanthodes, B. neoxanthodes and B. paraxanthodes and to distinguish each species individually were developed and validated. The assay targeting the three species can be used if suspecting either of them, whereas the assay targeting each can be used separately. Tephritidae samples are regularly intercepted at the New Zealand border every year and all are immature life stages, mainly eggs and larvae, and cannot be identified to genus or species level morphologically. Most of B. xanthodes interceptions were from cooked breadfruit, thus the immature stage samples could not be reared to adult for morphological identification. Molecular identification is needed to identify the samples, which typically consists of PCR, gel electrophoresis and sequencing, and is time consuming. The real-time PCR assays developed in this study provide a rapid means for identifying the interceptions, are conformant with the MIQE guidelines for qualitative assays (Bustin et al., Reference Bustin, Benes, Garson, Hellemans, Huggett, Kubista, Mueller, Nolan, Pfaffl, Shipley, Vandesompele and Wittwer2009). High specificity and sensitivity are much desired in border diagnostics and the assays showed high efficiency and sensitivity in detecting the target species. In the specificity test, the closely related species and the common interceptions of Bactrocera species (tables 1 and 2) were used for validation of the real-time PCR assays and no cross-reactions were observed. The assays also demonstrated their sensitivities by being able to detect ten copies µl−1 of target DNA (fig. 1).
The application of molecular identification for the diagnostics of Bactrocera species has increased in recent years (Armstrong et al., Reference Armstrong, Cameron and Frampton1997; Jamnongluk et al., Reference Jamnongluk, Baimai and Kittayapong2003; Armstrong & Ball, Reference Armstrong and Ball2005; Chua et al., Reference Chua, Chong and Lim2010; Asokan et al., Reference Asokan, Rebijith, Singh, Sidhu, Siddharthan, Karanth, Ellango and Ramamurthy2011; Blacket et al., Reference Blacket, Semeraro and Malipatil2012; Jiang et al., Reference Jiang, Li, Deng, Wu, Liu and Buahom2013, Reference Jiang, Liang, Zhang and Li2014; Meeyen et al., Reference Meeyen, Sopaladawan and Pramual2014; Dhami et al., Reference Dhami, Gunawardana, Voice and Kumarasinghe2016; Kunprom & Pramual, Reference Kunprom and Pramual2016). Among those molecular diagnostics, PCR-RFLP have been used for the detection of a number of fruit fly species (Armstrong et al., Reference Armstrong, Cameron and Frampton1997; Chua et al., Reference Chua, Chong and Lim2010) and could be used for B. xanthodes species (Armstrong et al., Reference Armstrong, Cameron and Frampton1997). However, the PCR-RFLP tests can be vulnerable to false negatives if not all population-level variation had been accounted for, and also false positives if the sequences for closely related species are not available during the method development. For the PCR-RFLP assay for B. xanthodes, no sequences were available for the closely related species, B. neoxanthodes and B. paraxanthodes when the assays were developed, thus further validation is needed to avoid false positives. Furthermore, DNA barcoding can provide an accurate identification for B xanthodes to species level, however, this approach is time-consuming and typically takes up to 4 days to receive the results and often depends on an external facility to provide sequence data. In addition, species-specific PCR was also used for the identification of Bactrocera species, such as B. tau, zonata and correcta (Asokan et al., Reference Asokan, Rebijith, Singh, Sidhu, Siddharthan, Karanth, Ellango and Ramamurthy2011; Jiang et al., Reference Jiang, Li, Deng, Wu, Liu and Buahom2013), but no B. xanthodes-specific PCR has been reported. This method normally targets smaller PCR fragment, which will effectively amplify degraded DNA, however it involves the post PCR steps, such as gel electrophoresis. In comparison, the real-time PCR protocol can provide rapid identification and no post PCR steps are needed, thus it can provide a rapid identification. Therefore the assay developed for targeting B. xanthodes complex in this study will allow for rapid detection and greatly assist the morphological diagnostics at New Zealand borders.
Conclusion
Here, we report the DNA barcoding for the fruit fly species, B. xanthodes, B. neoxanthodes and B. paraxanthodes. This is the first DNA barcoding sequences of the COI gene for B. neoxanthodes and B. paraxanthodes, which enriches the reference sequences for Bactrocera species in BOLD and GenBank databases. The real-time PCR assays developed for B. xanthodes species complex in this study are suitable for routine application by diagnostic and research agencies, for facilitating exports and imports. This assay has been fully optimized for immediate deployment at New Zealand border and has the potential to be applied in the detection of the target species in other countries around the world.
Acknowledgements
The authors thank Aurelie Chan from Veterinary, Food and Phytosanitary Inspection Service, Directorate of Veterinary, Food and Rural Affairs, New Caledonia for providing the B. paraxanthodes and several other Bactrocera species; Merriam Toalak and late Bule Sylverio from Biosecurity Vanuatu, PMB 9086, Port Vila, Vanuatu for providing B. neoxanthodes specimens. They thank Dr Olivia Reynolds from NSW Department of Primary Industries, Menangle, New South Wales, Australia for providing the B. tryoni specimens for testing the PCR assays. They also thank Joe Tang from MPI for reviewing the manuscript and providing the valuable suggestions and thank the editor and two anonymous reviewers for their comments and suggestions to improve the manuscript.