Introduction
Carbohydrates are essential energy-producing nutrients required for both optimal larval growth and for maintenance of imago longevity in case of majority of insects. Herbivorus insects feed directly on the plant tissues, many of them exclusively on starchy seeds during their larval and/or imago stages. However, Colorado potato beetle (CPB) (Leptinotarsa decemlineata, Say) has a different dietary habit since imagoes as well as larvae can attack leaves of the plants and destroy the entire crop. CPB is a crop pest of economic interest, feeds exclusively on the members of family Solanaceae, for example, Solanum tuberosum (potato), S. melongena (egg-plant), S. lycopersicum (tomato). In potato plants, leaves are the major organs responsible for the production of carbohydrates, most commonly sucrose and starch, via photosynthesis. The starch content of potato leaves is high and varies between 2.9 and 12.9% according to the light fluctuations (Santacruz et al., Reference Santacruz, Andersson and Aman2005). Potato beetle has impressive feeding rates, and high fecundity (Harcourt, Reference Harcourt1971). One beetle is able to consume even 40 cm2 of potato leaves during the larval stage, and an additional 10 cm2 per day as an adult (Ferro et al., Reference Ferro, Logan, Voss and Elkinton1985). Digestive enzymes are hydrolases, and their nature is related to the nature of the meal that an insect can assimilate. Carnivorus insects secrete mainly proteases whereas herbivorus insets, like CPB, secrete more carbohydrases to carry out the complete hydrolysis of polysaccharides down to the constituent monosaccharides. Amylase is the main enzyme involved in the digestion and carbohydrate metabolism in insects (Horie & Watanabe, Reference Horie and Watanabe1983). Sequences of some insect α-amylases are known, but mainly α-amylases of cereal eating insects were studied like bruchid (Zabrotes subfasciatus) (Grossi de Sa & Chrispeels, Reference Grossi de Sa and Chrispeels1997), western corn rootworm (Diabrotica virgifera virgifera) (Titarenko & Chrispeels, Reference Titarenko and Chrispeels2000), mealworm beetle (Tenebrio molitor), and its larvae yellow mealworm (Buonocore et al., Reference Buonocore, Poerio, Silano and Tomasi1976). X-ray diffraction study of T. molitor larva α-amylase (PDB: 1viw) has been reported by Nahoum et al. (Reference Nahoum, Farisei, Le-Berre-Anton, Egloff, Rougé, Poerio and Payan1999) and since then it is the only insect amylase with a known 3D structure.
Despite the studies on carbohydrate digestion in insects, there is a lack of data relating the different enzyme activities and their product patterns. Some of the early product pattern studies aimed only at endo/exo characterization of insect amylases. In this point of view, amylases originated from different Sitophilus species were found rather similar to porcine pancreatic α-amylase (PPA) and not to sweet potato β-amylase. These amylases were selected as endo and exo amylase models, respectively (Baker, Reference Baker1983). In contrast, hydrolysis products of T. molitor midgut enzymes, identified as amylase, were studied on amylopectin, glycogen and β-limit dextrin. Oligosaccharide products from pentamer to dimer and glucose were separated by TLC. After a longer incubation di- and trisaccharides became the main products, the intensity of pentamer and tetramer spots decreased (Applebaum, Reference Applebaum1964). A purified tasar silkworm (Antheraea mylitta) α-amylase liberated maltose, maltotriose and maltotetraose form starch after a longer incubation (Nagaraju & Abraham, Reference Nagaraju and Abraham1995).
Some properties (e.g. pH and temperature optimum) of α-amylase of CPB were published earlier (Khorram et al., Reference Khorram, Abad, Yazdaniyan and Jafarnia2010), but the process of the enzyme action remains undiscovered. Our aim was to investigate the process of carbohydrate digestion in the gut of the CPB. For this purpose, different chromophore-containing substrates were applied in the course of our experiments. These substrates allow studying processes catalyzed by amylase alone or together with glycosidases. Product pattern were compared to known amylases, like PPA, human salivary (HSA), barley, and Bacillus licheniformis α-amylase (BLA), studied in our laboratory and published earlier (Kandra et al., Reference Kandra, Gyémánt, Farkas and Lipták1997, Reference Kandra, Gyémánt, Remenyik, Hovánszki and Lipták2002, Reference Kandra, Gyémánt, Remenyik, Ragunath and Ramasubbu2003, Reference Kandra, Hachem, Gyémánt, Kramhøft and Svensson2006).
Materials and methods
Sample collection and preparation
Leptinotartsa decemlineata larvae (third and fourth larval phase) and imagoes were collected from the potato research field of the University of Debrecen, Faculty of Agricultural and Food Sciences and Environmental Management, Institute of Plant Protection. The digestive tract was removed from the live beetles. Gut was made available by decapitation and cutting the abdomen and the thorax of the beetle. After that the gut was able to be removed by a careful movement. The prepared digestive tract was put into 250 or 500 µl physiological saline solution (0.9% NaCl, pH 6.5), was vortexed for 30 s then centrifuged for 5 min on 10,000 g at 5°C. The supernatant was separated into a clean tube and immediately transferred to the laboratory to store at −20 or −80°C until activity measurements.
The samples from larvae and imagoes were separated and divided into three groups based on their saturation: empty, half full, and totally full gut.
Measurement of enzyme activities
2-Chloro-4-nitrophenyl-4-O-α-D-galactopyranosyl-maltoside (GalG2CNP fig. 1a, SORACHIM S.A., Lausanne, Switzerland)), as amylase substrate, 4-nitrophenyl-α-D-glucopyranoside (PNP-α-G, Sigma-Aldrich, Hungary), as α-glucosidase, and 4-nitrophenyl-β-D-glucopyranoside (PNP-β-G, Sigma), as β-glucosidase substrates were used.
Kinetic experiments were carried out at 30°C in 50 mM MES buffer pH 6.0 containing 5 mM Ca(OAc)2, 51.5 mM NaCl, and 152 mM NaN3. This buffer composition is optimal for exclusive aglycon cleavage by α-amylases (Morishita et al., Reference Morishita, Iinuma, Nakashima, Majima, Mizuguchi and Kawamura2000).

Fig. 1. Structures of α-amylase substrates a) α-(2-Chloro-4-nitrophenyl)-β-1,4-galactopyranosylmaltoside, b) 2-Chloro-4-nitrophenyl-maltoheptaoside c) 4,6-O-benzylidene-4-nitrophenyl-maltoheptaoside.
A 200 µl aliquots of 5 mM substrate and MES buffer of 290 µl were mixed in a half-micro cuvette and incubated at 37°C for 5 min. The reaction was initiated by adding 10 µl CPB gut extract into the substrate and continuous measurement of absorbance started immediately. Kinetic curves of CNP or PNP release were measured continuously at 400 nm using the Parallel Kinetics Analysis program of a JASCO V550 (JASCO Tokyo, Japan) spectrophotometer. Progress curves were plotted and fitted to a straight line. All experiments were repeated three or five times. Normalized ΔA min−1 values, proportional to initial rate, were considered to be enzyme activities.
HPLC action pattern determination
Agilent 1260 Infinity II (Quaternary pump, Vial sampler, Diode Array Detector) liquid chromatograph and Accucore aQ column (150 × 2.1 mm, 2.6 µm, Thermo) were used for separations. The column and vial sampler temperature was maintained at 30°C for all of the analysis. Eluent A was HPLC grade water, eluent B was acetonitrile (Sigma-Aldrich). Flow rate was 0.4 ml min−1, compounds bearing chromophore aglycon were detected by diode array detector at 302 nm. Injection volume was 5 µl.
Reaction of 2-chloro-4-nitrophenyl-β-maltoheptaoside (CNPG7, fig. 1b) (1 mg ml−1) was analyzed using 5 µl gut extract of CPB in 1 ml solution of CNPG7 substrate. Isocratic elution was used for separation of products applying an eluent containing 7% (V/V) acetonitrile in water. Samples were injected every 16 min.
The hydrolysis of 4,6-O-benzylidene-4-nitrophenyl-β-maltoheptaoside (BzG7PNP fig. 1c) (1 mg ml−1) was examined in a similar way, but using gradient elution. Gradient steps were: 0 min 5% B; 4 min 5% B; 5 min 7% B; 13.30 min 7% B; 14 min 30% B; 30 min 30% B; 5 min post time. Samples were injected every 35 min.
Statistical analysis
Descriptive statistics were used to summarize α-amylase activity measurements. Median, mean, and quartiles for ΔA min−1 data of the gut extracts obtained from larva and imago samples were calculated and represented as box plot using Origin 7 (OriginLab Corp. USA).
Results and discussion
In CPB, the potential carbohydrate digestive enzymes are α-amylases, α-, and β-glucosidases. Generally accepted methods for the insect enzyme extraction apply the extract of the whole imago or larva, as an enzyme source (Rothe, Reference Rothe, Eisenthal and Danson2002). Other authors recommend the preliminary preparation of insect gut and then the extraction of insect enzymes for activity and inhibition studies (Bandani et al., Reference Bandani, Kazzazi and Mehrabadi2009; Khorram et al., Reference Khorram, Abad, Yazdaniyan and Jafarnia2010), or combine the two methods depending on the size of insect (Ashouri et al., Reference Ashouri, Pourabad, Bandani and Fatehi2016). Gut extracts of larva or imago stage CPBs were used in our experiments. In this study, we applied defined substrates of low molecular weight because their reaction patterns can be exactly determined. GalG2-CNP substrate made a simple and quick α-amylase activity determination possible and measured exclusively the α-amylase activity from insect gut extract. In contrast, starch substrate and dinitrosalicylic acid method, applied by Ashouri et al. (Reference Ashouri, Pourabad, Bandani and Fatehi2016) and Khorram et al. (Reference Khorram, Abad, Yazdaniyan and Jafarnia2010), measured not only α-amylase but also α-glucosidase activity, as well.
Determination of α-amylase activity in gut extracts
Statistical evaluation of amylase activity (ΔA min−1) was made in larva (L3–L4) or imago state and according to gut saturation. The median and range of α-amylase activity in different development states were displayed in fig. 2. Significant activity was measured in larvae only in the case of gut filled with nutrients. Mean values obtained from other groups did not differ significantly from each other, although, variability of amylase activity was found to be considerably high. Extracts with higher activity were used for further measurements and we always used the same extract to determine a given parameter.
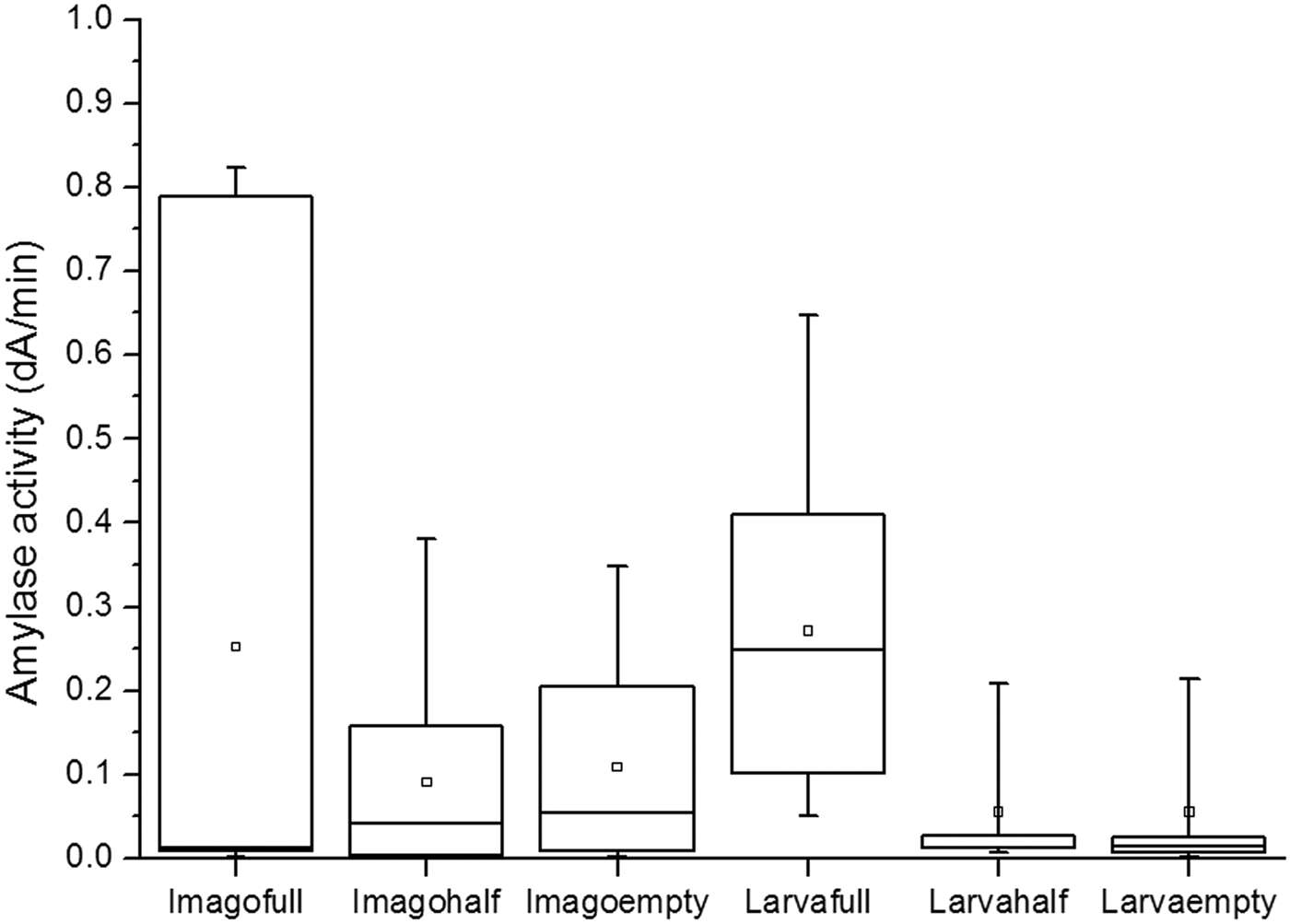
Fig. 2. Statistical analysis of α-amylase activity values of gut extracts from L. decemlineata. Box represents second and third quartiles, line shows the median, □ character represents the mean of the data, minimum and maximum are depicted by lines.
Thermal stability and pH optimum of α-amylase activity of gut extract measured on GalG2−CNP substrate
Since the optimum temperature is not a true property of enzymes, we determined thermal stability of LDAmy by first exposing the gut extracts to a range of temperatures (20–70°C) for 30 min, and subsequently measuring its activity at 30°C according to the regulation of the International Union of Biochemistry and Molecular Biology. As we found amylolytic enzymes of CPB are most stable in the range of 30–40°C (Fig. S1). When exposed to temperatures above 60°C, the enzyme activity was almost completely lost. The optimal temperature for LDAmy in the study of Khorram et al. (Reference Khorram, Abad, Yazdaniyan and Jafarnia2010) was 37°C, although the range of study was rather narrow 25–45°C. Insects’ α-amylases are active over a broad range of temperatures from 30 to 60°C, where a sharp decrease in enzyme activity occurs. Some Coleoptera insect enzymes like amylases from Cryptolaemus montrouzieri (Ahmadi et al., Reference Ahmadi, Khani and Ghadamyari2012), Lasioderma serricorne (Sajjadian et al., Reference Sajjadian, Hosseininaveh and Vatanparast2012) have higher optima temperature of 50°C as compared to LDAmy.
The α-amylase from L. decemlineata was most active at pH = 6.0 (Fig. S2) similarly to the reported optimum activity of many coleopteran larval amylases in the acidic range between 4.8 and 7.0 (Podoler & Applebaum, Reference Podoler and Applebaum1971; Buonocore et al., Reference Buonocore, Poerio, Silano and Tomasi1976; Terra & Ferreira, Reference Terra and Ferreira1994). In contrast, many lepidopteran insect species including Bombix mori (pH 9.2), Spodoptera littoralis (9.5), Mammestra brassicae (pH 9.5), Erinnyis ello (pH 9.8), and A. mylitta (9.5) are known to be active in the alkaline pH range (Nagaraju & Abraham, Reference Nagaraju and Abraham1995).
Product pattern studies
The present studies were aimed at determining product pattern of L. decemlineata gut enzymes on oligosaccharide substrate to get information about the carbohydrate digestion of potato beetle. A chromophore containing maltooligosaccharide CNPG7 was selected as a substrate for HPLC measurements, since β-glycosidic linkage of aglycon is stable and is not hydrolyzed by α-amylases and α-glucosidases potentially present in gut extract. The UV absorption of CNP aglycon at 302 nm allowed the detection of substrate and reducing end products after reversed phase HPLC separation. Similar substrates have been applied earlier for action pattern studies of different amylases in our laboratory (Kandra et al., Reference Kandra, Gyémánt, Farkas and Lipták1997, Reference Kandra, Gyémánt, Remenyik, Hovánszki and Lipták2002, Reference Kandra, Gyémánt, Remenyik, Ragunath and Ramasubbu2003, Reference Kandra, Hachem, Gyémánt, Kramhøft and Svensson2006).
It was not surprising that the cleavage profile was different from that we found for mammalian amylases. The LDAmy was not purified from the other gut enzymes and therefore we could realize the presence of other carbohydrate digestive enzymes; namely α- and β-glucosidases. The α-glucosidases, which are exo-enzymes, cleave one glucose unit from the non-reducing end of the substrate leading to the formation of CNPG6 and CNPG5 products. The all products of α-amylase and α-glucosidase catalyzed reactions are substrates of further α-glucosidase cleavage. CNPG appeared in the chromatogram, but level was low even after complete hydrolysis of the CNPG7 substrate (fig. 3). However, CNPG product was substrate for β-glucosidase, since the chromophore aglycon was β-linked, and the cleavage of aglycon resulted in yellow color formation after a longer incubation verifying the presence of β-glucosidase. The main products were dimer and trimer after a longer incubation. This product distribution is very similar to that what had previously determined by Applebaum (Reference Applebaum1964) for T. molitor midgut enzymes. This substrate was useful to illustrate the sequential action of enzymes during the digestion process.
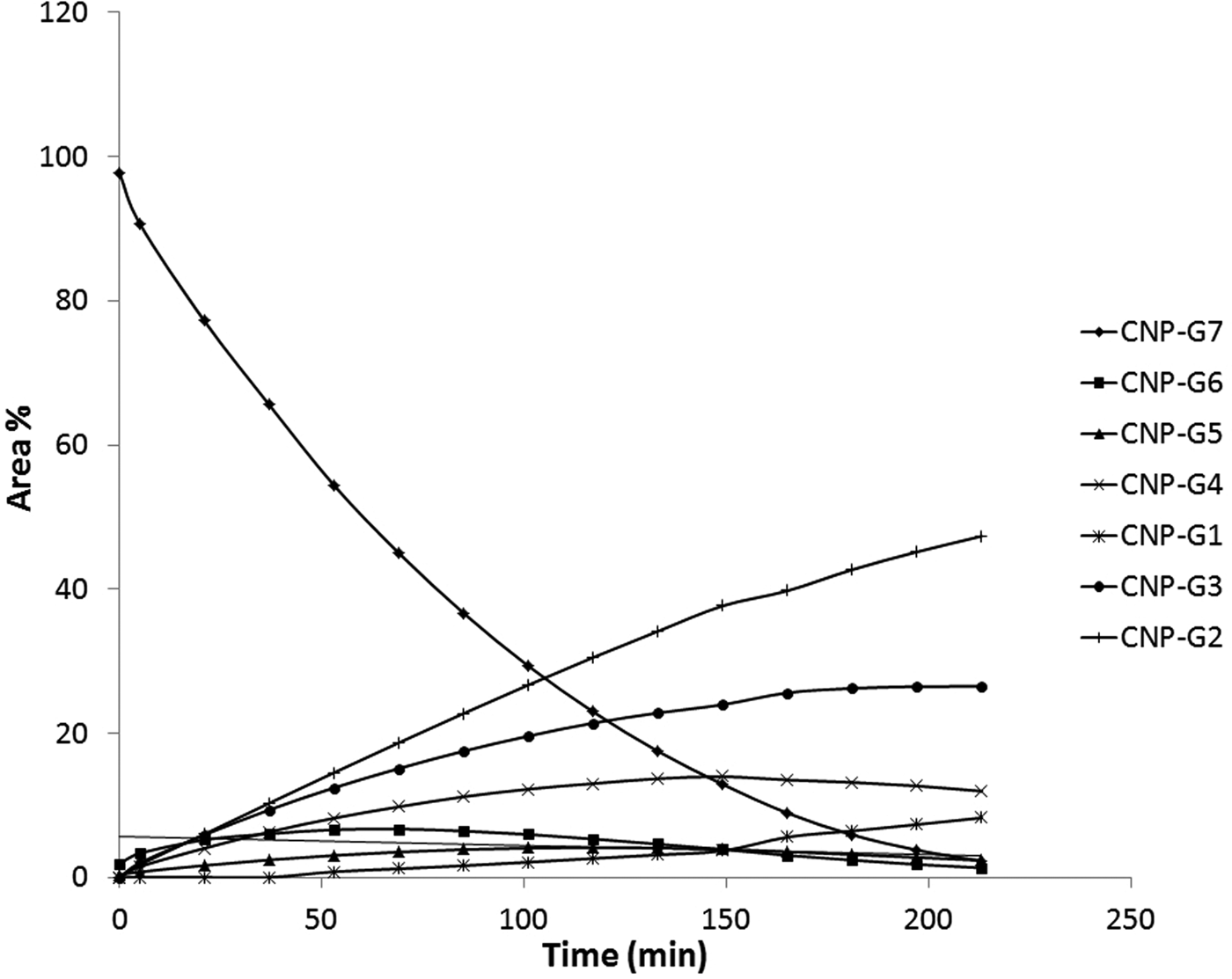
Fig. 3. Kinetic curves of CNPG7 hydrolysis reaction catalysed by potato beetle gut extract. Data were obtained from RP-HPLC analysis of reaction mixture at different times.
The product ratios at 5 min have been selected from data of fig. 3, where secondary hydrolysis was not significant, to compare the present results to that of we published earlier. CNPG6 and CNPG5 as probably α-glucosidase products were neglected in this calculation. In addition to mammalian α-amylases, representatives of bacterial and plant α-amylases were listed in table 1.
Table 1. Percentage product ratio of CNPG7 hydrolysis catalyzed by α-amylases of different origin.

bold values indicates similar results.
The main products (CNPG2–CNPG4) of CNPG7 hydrolysis give a very similar profile to that of hydrolysis catalyzed by PPA and HSA, and significantly different from products of bacterial and plant enzymes. We can assume that the substrate binding site of LDAmy should be very similar to mammalian α-amylases, first of all to the well-known five subsite model of PPA.
The presence of glucosidases was also verified in separate experiments using the usual chromophore-containing monomer substrates PNP-α-G and PNP-β-G and spectrophotometry at 400 nm. The relative activity values, calculated from slope of kinetic curves plotted by kinetic measurement program of JASCO V550 device/apparatus, showed similar order of magnitude of reaction rates (Fig. S3). CPB β-glucosidase was classified as aryl β-glucosidase (Class B) since it has an activity on PNP-β-G (Terra & Ferreira, Reference Terra, Ferreira and Gilbert2012). Exact proportion of enzymes was not calculated, since optimum conditions were determined only for α-amylase.
In addition, a 4,6-O-benzylidene-modified PNPG7 oligomer, which is very stable toward hydrolysis by exo-glycosidases (Cornaggia et al., Reference Cornaggia, Ivory, Mangan and McCleary2016), was applied for monitoring the real and exclusive α-amylase action pattern. These results create an opportunity to draw conclusions about the structure of the LDAmy active site not previously mapped.
Initial rate calculation was made using area data of HPLC measurements in the early stage of reaction at lower than 10% transformation of BzG7PNP substrate (fig. 4), which was guaranteed by lower concentration of gut extract in reaction mixture. PNPG6 and PNPG5 products were not formed during the reaction, verifying that α-glucosidase does not cleave the benzylidene-modified substrate. The relationship between time and peak area of products was linear in the studied time interval and reaction rate was calculated as a slope of linear fitting. The ratio of reaction rates gave the cleavage frequency of the LDAmy on heptamer substrate.
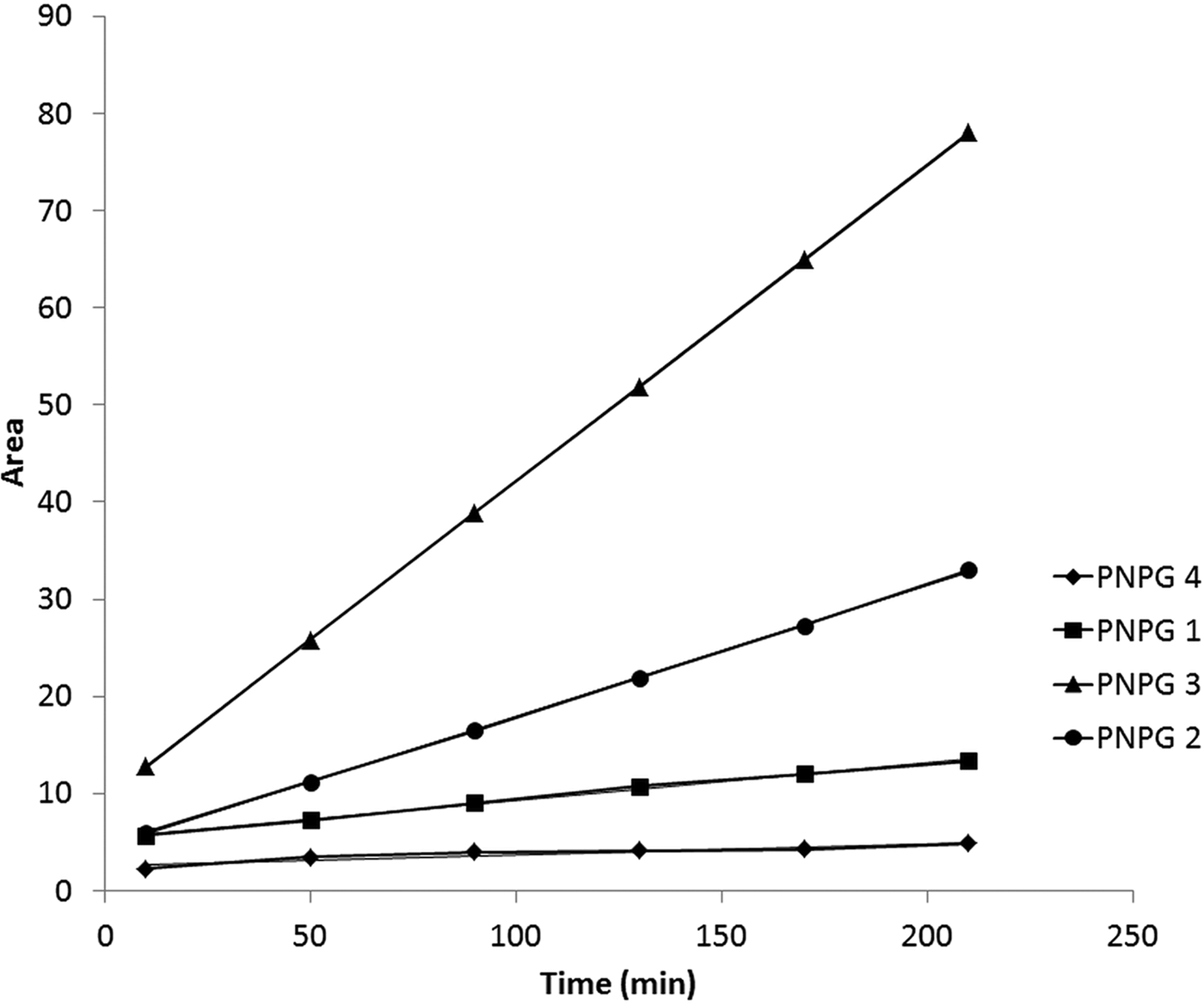
Fig. 4. Initial rate measurements of α-amylase catalyzed hydrolysis reaction on BzG7PNP substrate measured by HPLC (< 10 % transformation).
BzG7PNP substrate was used earlier for action pattern determination of other α-amylases (PPA, HSA, BLA) and results were compared using data of table 2.
Table 2. Product pattern of BzG7PNP hydrolysis catalyzed by α-amylases of different origin.

bold values indicates similar results.
The action pattern of LDAmy on BzG7PNP was similar to what had been specified earlier for PPA. It confirms our earlier assumption of five-subsite structure and verified that after 5 min reaction CNPG7 hydrolysis products were formed mainly by α-amylase action, effect of glucosidases was negligible.
The action pattern of two coleopteran recombinant α-amylases, Callosobruchus chinensis α-amylase (CcAmy) and Tribolium castaneum α-amylase (TcAmy), has been studied on starch substrate recently (Channale et al., Reference Channale, Bhide, Yadav, Kashyap, Pawar, Maheshwari, Ramasamy and Giri2016). Hydrolysis products from glucose to maltotetraose were present in different ratio: CcAmy catalysis resulting in the shorter (DP 1–3), while TcAmy the longer (DP 2–4) oligomers.
Complete hydrolysis of BzG7PNP was monitored by RP-HPLC and gradient elution. At the start of the reaction, the heptamer substrate was hydrolyzed by α-amylase enzyme resulting in mainly trimer and dimer products. After a longer incubation amount of trimer product began to decrease and dimer became the main product due to the presence of α-glucosidase (fig. 5), since chromophore-containing reducing end products are substrates of α-glucosidase.

Fig. 5. Kinetic curves of reducing-end products from BzG7PNP hydrolysis catalyzed by Colorado potato beetle gut extract. Data were obtained from RP-HPLC analysis of reaction mixture at different time.
In conclusion, the applied oligomer substrates were suitable for exploring of carbohydrate digestion process of CPB, which was summarized in fig. 6. The α-amylase as endo-acting enzyme liberates dimer, trimer, and tetramer products from an oligosaccharide substrate in parallel reactions. Oligosaccharides are substrates of α-glucosidase and hydrolysis resulting in shorter and shorter products in consecutive process. The β-glycosidase was able to hydrolyze β-glycosidic linkage of aglycon but acts only on monomer substrate. Because of the central role of α-amylase in carbohydrate digestion of CPB, the mapping of the active site should provide a rational basis for development of new inhibitor molecules with increased affinity for LDAmy. The observed similarity between LDAmy and PPA raises the possibility of using known PPA inhibitors to inhibit LDAmy. Lack of inhibitor specificity in this case does not necessarily mean a problem, since the potato beetle consumes the other part of the plant than the humans, in contrast with cereal insect pests.

Fig. 6. Schematic representation of digestion process in gut of Leptinotarsa decemlineata.
Supplementary material
The supplementary material for this article can be found at https://doi.org/10.1017/S0007485319000099.
Acknowledgements
The research was supported by the EU and co-financed by the European Regional Development Fund under the project GINOP-2.3.2-15-2016-00008.