Introduction
Birds use electric infrastructure present in their environments (Andersson et al. Reference Andersson, Wallander and Isaksson2009, Lehman et al. Reference Lehman, Kennedy and Savidge2007). But this is neither safe for birds (APLIC 2006, Tintó et al. Reference Tintó, Real and Mañosa2010) nor for the electric system (Lammers and Collopy Reference Lammers and Collopy2007). Perching on power poles is the most evident example.
Studies of perching behaviour in birds have been based on ethology (i.e. Bonser Reference Bonser1999) and ecology (i.e. Dzialak et al. Reference Dzialak, Carter and Lacki2007), with birds of prey being the most frequently studied (i.e. Accipitriformes and Strigiformes, hereafter raptors), as they use different foraging strategies and exhibit different uses of habitats and perches (Schnell Reference Schnell1968). Different strategies allow raptors to improve their chances of prey detection and capture under certain ecological conditions (O’Rourke et al. Reference O’Rourke, Pitlik, Hoover and Fernández-Juricic2010). The predators amongst these species tend to hunt by sight, searching for prey from elevated perches or hovering positions above the prey’s habitat (Andersson et al. Reference Andersson, Wallander and Isaksson2009). The availability of perches can facilitate and increase hunting efficiency due to higher visibility and less energy expenditure than hovering (Fleming and Bateman Reference Fleming and Bateman2018).
Raptors perch on a variety of substrates depending on the species, habitat, and perch availability (Bohall and Collopy Reference Bohall and Collopy1984, Sheffield et al. Reference Sheffield, Crait, Edge and Wang2001, Tomé et al. Reference Tomé, Dias, Chumbinho and Bloise2011). Among these perching substrates are different human-made structures, including house roofs, fences, wires, posts, traffic signs and utility poles, such as antennae or power pylons (Varland et al. Reference Varland, Klaas and Loughin1993, Rutz et al. Reference Rutz2006).
Power pylons have two main uses for raptors—as perches and nest substrate (Steenhof et al. Reference Steenhof, Kochert and Roppe1993, Sánchez et al. Reference Sánchez, Bueno, Rodríguez, González, Álvarez, Ramos and Caldera1996, Infante and Peris Reference Infante and Peris2003). Power pylons are amongst the perches most widely used by ma raptors (Knight and Kawashima Reference Knight and Kawashima1993). This leads to a range of problems for power lines (electricity failure; Lammers and Collopy Reference Lammers and Collopy2007), raptor (electrocution; Lehman et al. Reference Lehman, Kennedy and Savidge2007) and the environment (forest fires; Guil et al. Reference Guil, Soria, Margalida and Pérez-García2018).
Perching on power pylons entails a variable level of risk for raptors, depending not only on their own physical characteristics (Janss Reference Janss2000), and habitat (Hernández-Lambraño et al. Reference Hernández‐Lambraño, Sánchez‐Agudo and Carbonell2018, Bedrosian et al. Reference Bedrosian, Carlisle, Woodbridge, Dunk, Wallace, Dwyer, Harness, Mojica, Williams and Jones2020) but also on power pylon configuration (Guil et al. Reference Guil, Fernández-Olalla, Moreno-Opo, Mosqueda, García, Aranda, Arredondo, Guzmán, Oria, Margalida and González2011, Dwyer et al. Reference Dwyer, Harness and Donohue2014). Larger birds are usually at greater risk of electrocution, as they can come into contact with two different phases or a grounded element (such as the crossarm) and a phase (APLIC 2006). Anchor pylons, with strain insulators, and with or without other equipment, are amongst the most dangerous types of power pylons (Tintó et al. Reference Tintó, Real and Mañosa2010; Guil et al. Reference Guil, Fernández-Olalla, Moreno-Opo, Mosqueda, García, Aranda, Arredondo, Guzmán, Oria, Margalida and González2011; Hernández-Lambraño et al. Reference Hernández‐Lambraño, Sánchez‐Agudo and Carbonell2018). As pylons with strain insulators account for almost 30% of the overall (4,989 out of 15,029 pylons in our database), retrofitting them may only partially reduce the global risk of a power line.
In a pylon-by-pylon approach, retrofitting anchor pylons has been shown to be insufficient to avoid electrocution risk, even when carried out following the established legal criteria (using both structural and isolation measures; Guil Reference Guil2014a). Spanish bird-regulation (Royal Decree 1432/2008) has banned phases over the crossarm and established a minimum distance of 1 m between the crossarm and the phase for anchor pylons included in areas of bird conservation. However, many options are available to achieve this distance, including the use of different materials for the insulators or the use of metal extensions. Even within the same electricity distributor there are different crossarm designs and options for achieving minimal established distances. Mortality surveys suggest that there may be huge differences among these options in terms of electrocution risk (Tintó et al. Reference Tintó, Real and Mañosa2010, Guil et al. Reference Guil, Fernández-Olalla, Moreno-Opo, Mosqueda, García, Aranda, Arredondo, Guzmán, Oria, Margalida and González2011). While the amendment of structural power pylons tends to eliminate phases over the crossarms, eliminating mortality spots in the field is pivotal, so other factors influencing mortality must be considered. An analysis of the relationship between the different power pylon strain insulator configurations and raptor perching behaviour is urgently needed to determine the safest configurations. The reason is not only for raptor conservation but also to minimise human-wildlife conflicts. To our knowledge, there are no available approaches for developing this type of analysis.
Our main goal in this study was to develop a procedure for evaluating the way raptors perch on six of the most commonly used strain insulator configurations (all of them fulfilling the requirements of the Spanish regulations), focusing on prioritising strain insulator configurations for raptor conservation, that can be used anywhere.
Methods
Work concept and design
We designed a study with three complementary phases. The first was observational, under controlled conditions. We observed the behaviour of birds in relation to six of the most commonly used strain insulator configurations in Spain. We included an analysis of where and how frequently they perched (on which strain insulator and element). The second phase consisted of developing a risk index for evaluating the perchings, based on the combination of the frequency of use of each part of each insulator strain and the hazard of this perching. The third and last phase involved the development of a procedure to evaluate the least risky strain insulators, using the previously obtained risk indices.
To develop the observational phase, we decided to observe perching behaviour in controlled conditions using flying pens. We selected six wildlife rescue centres (WRCs hereafter) in central Spain. At each of these centres, one or more large enough flying pens were used to develop the perch trials (Figure 1). We defined five a priori groups of raptors based on species size (partially after Soldatini et al. Reference Soldatini, Albores-Barajas, Lovato, Andreon, Torricelli, Montemaggiori, Corsa and Georgalas2011 and García, Reference García2013, Reference García2017; Table 1) and aimed to test at least 30 individuals in each group.
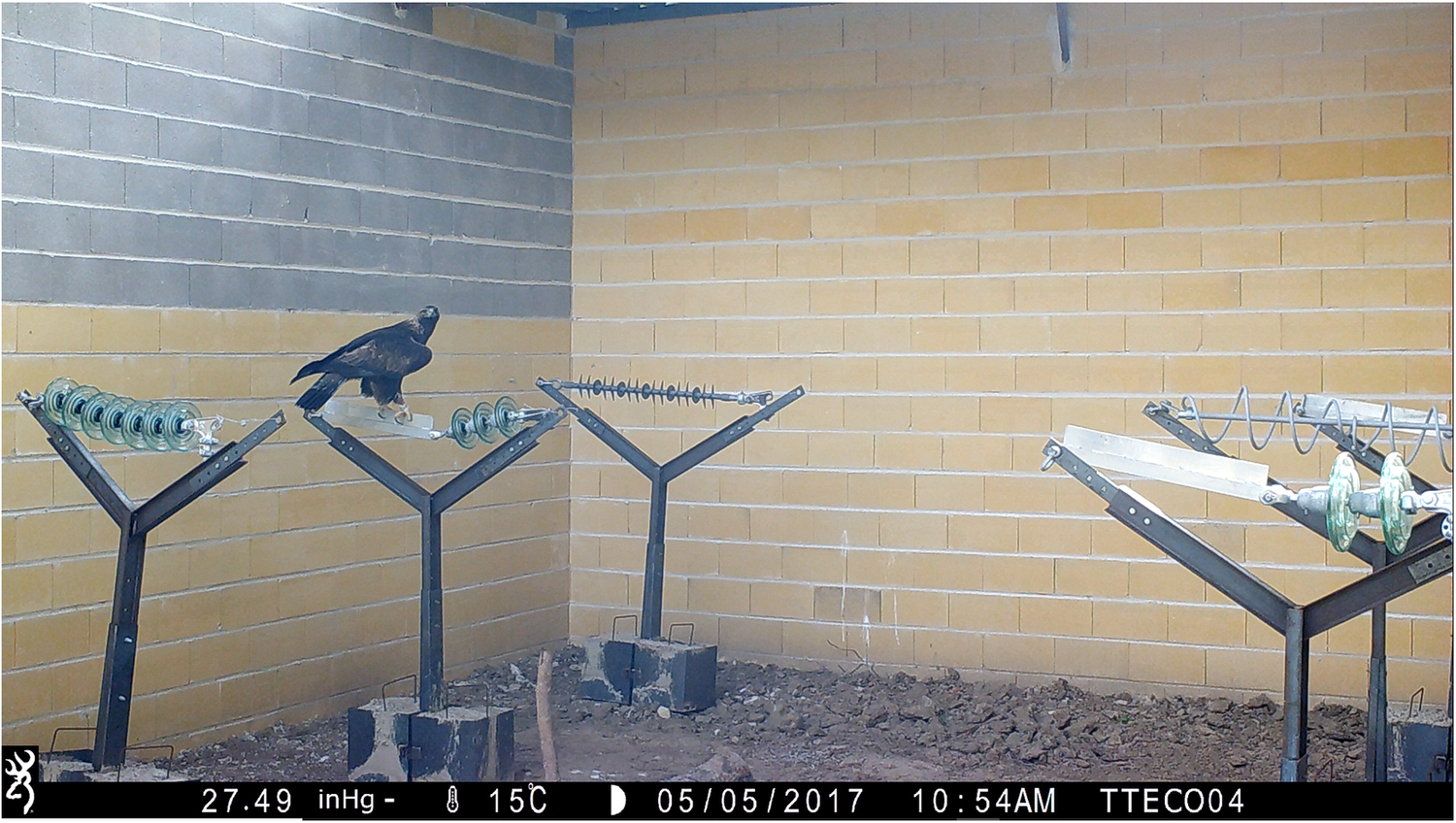
Figure 1. General set up of a perching trial in GREFA. An Aquila chrysaetos perching on the metal extension of a 3 U40 strain insulator
Table 1. A priori groups of raptors and species included. Shown is the number of specimens involved in the perch trials (N), the centres where they were perch trialed, the number of complete days for the perch trials (Days), the number of perchings recorded (Perches), (MN), the semi-metacarpal length in centimetres (SL1), the standard deviation for semi-metacarpal length (SD1), the semi-wingspan length (SL2) and the standard deviation for semi-wingspan length (SD2). If no specimens were measured for a complete species, we assigned it the class average (in bold). *There are two measured specimens for the semi-metacarpal length and four for the wingspan

To build the risk index and the procedure, we used approach of Allan (Reference Allan2006). We defined a hazard and calculated the frequency of use. First, we assigned a hazard to each perch in each element of each insulator strain by each species that varied according to the bird’s dimensions, and the distance to both ends of the strain insulator (Bedrosian et al. Reference Bedrosian, Carlisle, Woodbridge, Dunk, Wallace, Dwyer, Harness, Mojica, Williams and Jones2020). In a second step, we considered the frequency of each kind of perching, compared to the group overall.
To develop a procedure for evaluating the risk indices, we established criteria considering both the electrocution risk index and this index particularised for the most electrocution-prone and endangered group of species in Spain, the great eagles (Aquila genus; Madroño et al. Reference Madroño, González and Atienza2004, Guil et al. Reference Guil, Colomer, Moreno-Opo and Margalida2015). Electrocution is a crucial factor especially for the most threatened great eagles, Spanish Imperial Eagle Aquila adalberti and Bonelli’s Eagle A. fasciata (Real et al. Reference Real, Grande, Mañosa and Sánchez-Zapata2001, González et al. Reference González, Margalida, Manosa, Sánchez, Oria, Molina, Aranda and Prada2007).
Observational phase: Birds and measurements
Birds used in this study were from different WRCs. All of the birds were adults, with the exception of four Spanish Imperial Eagles in their first year. All the birds were considered healthy and fully capable of flight. As electrocution risk is related to bird size (Janss Reference Janss2000), the hazard was designed to consider the differences in raptor dimensions. Two different measures were considered (partially after Dwyer et al. Reference Dwyer, Kratz, Harness and Little2015): spine to metacarpal (hereafter semi-metacarpal) and spine to the 9th primary remex (hereafter semi-wingspan). Measures from the spine were appraised because the aim of the index was to analyse the risk when the raptor perched on two different elements independently.
Raptors were measured with a flexible tape. We measured only one wing, usually the right wing. We placed each bird on its back, fully extended the wings, and recorded (1) the distance between the location where the flesh ended and the spine (semi-metacarpal) and (2) the distance between the spine and the 9th primary remex end (semi-wingspan). Measurement results are shown in Table 1.
Observational phase: The perch trials
The trial consisted of the introduction of at least one flying raptor to a flying pen with six different strain insulator configurations for at least two days (48 hours). We counted the number of days per perch trial; initial and final day were only considered as half-days. All flying pens were ample enough to guarantee safe raptor flight considering the minimum standards for wild raptor rehabilitation (Miller Reference Miller2012). The use of different flying pens in a WRC depended on the presence of unrecoverable established raptors: we generally used one or two previously empty flying pens, but the trials were also developed in flying pens where there were unrecoverable established raptors, to minimise disturbances to these specimens. In these cases, we only considered the flying raptors as part of the trials.
Strain insulator configurations were installed on Y-shaped metallic poles except in two flying pens in Fundación para la Investigación en Etología y Biodiversidad (FIEB hereafter) and in “Chaparrillo 1” where the strain insulators were fixed directly on the posts with a V-shaped metallic structure (see Figures 1 and 2). Each model of strain insulator configuration was randomly located in the flying pen and was not repeated within the different flying pens of the same WRC. And all models were available simultaneously. To assess perching behaviour, we used camera traps: at least two BTC-5HDE Browning Trail Cameras in each flying pen, using TimeLapse+ mode, so the camera shot in 10-min intervals and with each detected movement (for more details see Appendix S1 in the online supplementary material).

Figure 2. Strain insulator configurations assayed in this study
Perching selection was based on the number of perches each bird made in each different part of a different strain insulator VS the total number of perches of the bird, in each trial. When there were no distinguishable birds in the same flying pen, we followed a protocol to assign those perchings (Appendix S1). To assess the perching of the birds, we considered that each animal perched at least one time in the flying pen. Perching was assigned to the ground if the bird was not detected on an insulator. We also considered alternative perches (such as trees) as ground perchings.
Observational phase: The strain insulator configurations
We tested the following strain insulator configurations, representing the more frequently used standard models that meet the requirements of Spanish legislation. All these models are different designs fulfilling the same function. And all of these strain insulators are used in electric power distribution lines (up to 66 kV):
-
• CAON-C3670. Anti-perching composite insulator model C3670EBAV_AR (total length of 123,5 cm)
-
• 7 U40 glass. 7 glass insulators type U40 (total length of 100,3 cm)
-
• Composite-CS40. Composite insulator type CS40 and metal extension with anti-perching sheet APA 16-590 (total length of 131,8 cm)
-
• 3 U40 glass. 3 glass insulators type U40 and metal extension with anti-perching sheet APA 16-590 (total length of 117,8 cm)
-
• PECA-1000. Anti-perching composite insulator model PECA-1000 (total length of 135,5 cm)
-
• 2 U70 glass. 2 glass insulators type U70 and metal extension with anti-perching sheet APA 16-590 (total length of 109,4cm)
Risk index phase
As a first step, for building hazard, we characterised the risk of each perching as a combination of the bird size and the exact location on the strain insulator. We defined five levels of hazard (after Allan Reference Allan2006; see Figure 3 and a full diagram in Figure S1):
-
• Extreme hazard: if the bird perched on an element where the distance between each extreme of the strain insulator is smaller than double the semi-metacarpal length.
-
• Very high hazard: if the bird perched on an element where the distance between the centre of the strain insulator to one extreme of the insulator is smaller than the semi-metacarpal length and the distance to the other extreme of the insulator is smaller than the semi-wingspan.
-
• High hazard: if the bird perched on an element where the distance between each extreme of the strain insulator is smaller than double the semi-wingspan length or if the bird perched on an element where the distance between one extreme is smaller than the semi-metacarpal length and the other is smaller than the semi-wingspan+15 cm (according to INSHT 2011).
-
• Moderate hazard: if the bird perched on an element where the distance between each extreme of the strain insulator is smaller than double the semi-wingspan length+15 cm.
-
• Low hazard: the rest of the cases.
We estimated the distances between the perching site and the extremes of the strain insulator. For most of the perches, we considered the centre of the element, with three exceptions:
-
• Insulators: We distinguished this in thirds, one proximal (nearer to the strain clamp), one central and one distal. In each case, we considered the bird to be perched on the centre of each third. The exception is the insulator composed of two U70 glass insulators, for which we considered each insulator independently.
-
• Wire: If the bird perched on the wire, we considered it to be perched on the strain clamp.
-
• If the bird perched on two elements simultaneously, each length was calculated independently.
For calculating the frequency of use (FoU hereafter), we considered the number of perchings for a group on a certain insulator and hazard level and divided it with the global number of perchings of the group. This is:

Where:
-
• FoU is the frequency of use of a certain insulator and a hazard level
-
• xij is the number of perchings of a certain group in the insulator j with hazard level i

Figure 3. Extreme hazard of a vulture on a CAON-C3670 strain insulator. MC is used for metacarpal position. Original vulture picture from Eprdox at Wikimedia Commons.
Statistical analysis
We analysed different factors that influenced the use of each strain insulator, to assess how results were conditioned by the experimental design. We considered the number of perchings on each strain insulator, per species and perch trial as the dependent variable, and as independent variables:
-
• Type of WRC: whether or not there were alternative perches
-
• WRC: nested in the type of WRC, we considered:
-
◦ With alternative perches: Chaparrillo, CRAS, and Sierra de Fuentes
-
◦ Without alternative perches: CERI, FIEB, and GREFA
-
-
• Group, with five levels: vultures, great eagles, eagle owls, medium raptors, and small raptors
-
• Species, nested in group
-
• Strain insulator, with seven levels: one per insulator and the ground
-
• Number of days each specimen spent in each perch trial (cofactor)
-
• The total number of different images taken, multiplied by the number of birds of the same species in each perch trial (offset)
We used a negative binomial generalised linear model, with a log-link function. We also calculated deviance using the modEvA package (Barbosa et al. Reference Barbosa, Real, Muñoz and Brown2013) in R 3.4. (R Core Team 2017).
Procedure for prioritising strain insulator use
For general prioritising, we conceived a step-by-step procedure for prioritising the use of the different strain insulators. This is necessary because there are variations in the risk index between different raptor species. When a power pole is retrofitted, prioritisation of strain insulators should consider all raptor species, but attend to conservation priorities. First, we prioritised the absence of extreme hazard perchings, following Allan (Reference Allan2006). We then considered great eagles as the most sensitive group, both due to their susceptibility (Guil et al. Reference Guil, Colomer, Moreno-Opo and Margalida2015) and their conservation status in Spain (Madroño et al. Reference Madroño, González and Atienza2004. Thus, we developed the following procedure:
-
1. Absence of extreme hazard perchings for the target group; in our case, the great eagles
-
2. Lower FoU for very high hazard and for the target group (great eagles), if differences for a couple of strain insulators in step 1 are less than 5%
-
3. Lower FoU for extreme hazard for all the groups, if differences in step 2 are less than 5%
-
4. Lower FoU for very high final hazard for all the groups, if differences in step 3 are less than 5%
While these criteria can be changed for other purposes, when prioritising per species or group we conceived a similar procedure but with the specific data of each species or group:
-
1. Absence of extreme hazard perchings (for the species or group).
-
2. Lower FoU for very high hazard (for the species or group).
-
3. Lower FoU overall for all the groups.
Results
The perch trials
From May 2017 to February 2018, we carried out 83 analysed perch trials with raptors, at the following recovery centres: FIEB (15 perch trials), GREFA (18 perch trials), Chaparrillo (16 perch trials), Sª de Fuentes (13 perch trials), CERI (13 perch trials) and CRAS (8 perch trials), in chronological order (Table 1).
Overall, we analysed 475 complete survey days (as some perch trials included different species and groups), with an approximate number of 258,960 analysed pictures that comprised 6,766 perchings on strain insulators, with 365 different combinations of WRC, perch trial, species and strain insulator (Table 2 and Table S1).
Table 2. Main aspects of the perch trials, including the number of different perch trials, the number of specimens, the number of surveyed days and the number of observed perchings.

Raptor measures
We measured 100 raptors for semi-metacarpal length and 102 for semi-wingspan (representing 58% of all raptors). We measured 50% of the vultures used in the perch trials, 72.7% of the great eagles, 72.4% of the eagle owls, 45.5% of the medium-sized raptors and 71.4% of the small-sized raptors of those used in the perch trials (Table 1).
The statistical analysis
The negative binomial linear model explained 40.62% of the deviance. The key aspects considered in the model are the type of centre (whether or not there were alternative perches), the centre, the raptor group, the strain insulator, the number of days for each perch trial and an offset consisting in the total number of different images taken, multiplied by the number of birds of the same species in each perch trial (Table S2).
The risk index
Based on the results in the previous section, we considered as independent events the number of perchings of each species on each element of each strain insulator. The 6,766 perchings abovementioned comprised 1,454 different perching scenarios that were evaluated using the risk index. The overall final risk index for a group is the sum of the results per species (Figure 4).

Figure 4. Comparison of FoU, per species group, strain insulator and hazard levels.
Prioritising strain insulator configurations
According to the previously defined criteria and following the procedure described in Table S7, we prioritised the strain insulators as follows:
-
1. PECA-1000 strain insulator (absence of extreme hazard perchings and lower FoU of very high hazard for great eagles, compared to CAON-C3670 strain insulator)
-
2. CAON-C3670 strain insulator (absence of extreme hazard perchings)
-
3. 3 U40 glass strain insulator (lower FoU for very high hazard for all the groups)
-
4. 7 U40 glass strain insulator (intermediate FoU for very high hazard for all the groups)
-
5. 2 U70 glass strain insulator (higher FoU for very high hazard for all the groups)
-
6. Composite-CS40 strain insulator (higher FoU for very high hazard for great eagles)
Discussion
Access to energy is key for human development and has been recognized in the UN’s Sustainable Development Goal 7 (Ensure access to affordable, reliable, sustainable and modern energy for all). Reliable power networks are crucial to achieving SDG 7, but the environmental aspects of power networks are not considered (United Nations 2018). Bird-safe power networks have been proven to be more efficient (Guil Reference Guil2014b). This efficiency is linked to many factors, including interactions with birds.
The design of crossarms and strain insulators impacts electrocution rates (Guil et al. Reference Guil, Fernández-Olalla, Moreno-Opo, Mosqueda, García, Aranda, Arredondo, Guzmán, Oria, Margalida and González2011, Slater et al. Reference Slater, Dwyer and Murgatroyd2020). The absence of phases over the crossarm is a determining factor (Janss and Ferrer Reference Janss and Ferrer1999), but based on our data, when there are no phases over the crossarm, differences in the strain insulators can cause a 12-fold increase in electrocution rates. To our knowledge, this is the first standardised protocol developed for prioritising the use of strain insulators. Our results clearly indicate that the use of some strain insulators (PECA-1000 and CAON C3670, both over 1.2 m long) can provide an adequate aerial power supply while ensuring the safety of large raptors. All the other strain insulators present extreme hazards, at least for vultures, and should thus be discarded. However, these methods can be used to compare different elements to avoid conflicts between birds and power lines, especially prior to the expansion of large power networks with steel and concrete pylons. The knowledge gained from this work can be used worldwide by electricity utilities to select strain insulators for new construction or for retrofitting, to minimise raptor electrocution risk. As even when using the safest strain insulators there are still hazards for vultures, these might be minimised through using isolation covers for strain clamps.
The use of electric pylons and conductors as perches by raptors is common in most of the species studied (Schnell Reference Schnell1968). Moreover, it is known that poles are used based on the size of the species and their behavioural ecology (Bohall and Collopy Reference Bohall and Collopy1984). From a general perspective, small raptors can perch on both pylons and conductors (Varland et al. Reference Varland, Klaas and Loughin1993, Tomé et al. Reference Tomé, Dias, Chumbinho and Bloise2011). In contrast, larger raptor species tend to perch directly on the highest section of the pylons, particularly the crossarms (Janss and Ferrer Reference Janss and Ferrer1999), and are thus more susceptible to electrocution. Based on an assessment of the literature, these species have a worse conservation status, partly due to power line conflicts (Real et al. Reference Real, Grande, Mañosa and Sánchez-Zapata2001, González et al. Reference González, Margalida, Manosa, Sánchez, Oria, Molina, Aranda and Prada2007). Thus, large raptor species should be a key study target (McClure et al. Reference McClure, Westrip, Johnson, Schulwitz, Virani, Davies, Symes, Wheatley, Thorstrom, Amar, Buij, Jones, Williams, Buechley and Butchart2018). However, electrocution also affects other species (Guil et al. Reference Guil, Colomer, Moreno-Opo and Margalida2015), affecting their conservation status (Madroño et al. Reference Madroño, González and Atienza2004), and thus we developed a methodological approach valid for every species.
There were many aspects of the trials that may have conditioned the results. First, the selected strain insulators configurations; we selected those configurations because they were the most frequently used in Spain, fulfilling all the requirements of the current national regulations (both electrical and for bird protection). We do not consider that the development of new strain insulators would make our results out-of-date. On the contrary, they should be validated as bird-safe following the methodology developed.
The use of flying pens instead of field surveys allowed us to gather a consistent volume of data in a short period of time. In the only similar study combining flying pens and field surveys to our knowledge (Janss and Ferrer Reference Janss and Ferrer1999), which analysed perch deterrents, field surveys were only used to test whether or not some devices influenced mortality rates. We did not use camera traps to analyse birds perching on power pylons in the field as picture quality (and thus species identification) varied greatly with time of day and the incidence of light. Automated identification could be helpful in future projects (Norouzzadeh et al. Reference Norouzzadeh, Nguyen, Kosmala, Swanson, Palmer, Packer and Clune2018, O’Brien and Kinnaird Reference O’Brien and Kinnaird2008). Another important aspect of the trial design was the use of camera trapping instead of direct observation (see Dwyer et al. Reference Dwyer, Tincher, Harness and Kratz2016a, Reference Dwyer, Tincher, Harness and Kratz2016b). The use of WRCs also made the assessment of the species most affected by electrocution in Spain easier (Seamans et al. Reference Seamans, Barras and Bernhardt2007, Guil et al. Reference Guil, Colomer, Moreno-Opo and Margalida2015).
Our results showed that the number of perches per group was based on size and mobility, with more perches occurring per individual and per day in small-sized raptors, as stated in previous studies (Avery and Genchi Reference Avery and Genchi2004). In the statistical analysis, there are greater differences between the groups of the largest raptors (vultures, great eagles, and eagle owls) and no differences between the medium-sized and small raptors. A portion of the species component, attending to our sample size per species, may also be individual behaviour (Bowler and Benton Reference Bowler and Benton2005, DeVault et al. Reference DeVault, Blackwell, Seamans, Lima and Fernández-Juricic2015), which is studied more in larger animals. Within groups, we only found a clear relationship between species size and number of perches for the small raptors. As species were also an influencing factor in the statistical analysis, we found groups to be a more robust approach for risk analyses. Our logic is that birds in functional groups tend to be of similar size, so their perching behaviour on power pylons should tend to be more homogeneous than between species.
WRC was an important factor in explaining the number of perches, and we were able to group them according to whether or not they had alternative perches. The presence of alternative perches reduced the use of the strain insulators, as a crossarm would also likely do. The results of some studies suggest that anti-perching devices may even increase the electrocution rate of some species (Janss and Ferrer Reference Janss and Ferrer1999, Pérez-García et al. Reference Pérez-García, Morales-Reyes, Naves-Alegre, Sánchez-Zapata and Sebastián-González2019), and this might partially be related to the use of insulators as perching sites. In each WRC, the perch trials included a different species pool, and mainly on a progressive schedule. Thus, the interpretation of the different WRCs as influential factors appears to be complex. Centralised studies with a variable pool of raptor species may address this problem in future work.
Developing risk indicators and risk assessment procedures is a complex process (Tarling and Burrows Reference Tarling and Burrows2004). While every risk scenario may seem clear to a given set of authors, other researchers may consider different scenarios. We tried to follow a precautionary principle when assigning prioritising criteria (Klinke and Renn Reference Klinke and Renn2001), first to minimise the extreme hazards (Allan Reference Allan2006) and then to minimise very high hazards for the most endangered and electrocution-prone group, the great eagles (Madroño et al. Reference Madroño, González and Atienza2004, Guil et al. Reference Guil, Colomer, Moreno-Opo and Margalida2015). We also aimed to minimise risks for the other raptors, through a lower level of the indicator. We considered that with this approach had the precautionary principle as a guiding principle.
Our study supports a methodology of decision-making exportable to most power companies and to other countries facing similar challenges. They can apply the criteria or adapt the strain insulator that is most appropriate for the environment and species present in the field. In our case, where larger species are concentrated with higher electrocution rates (Guil et al. Reference Guil, Colomer, Moreno-Opo and Margalida2015), larger insulators have been prioritised.
A monitoring protocol should be developed for perch trialling strain insulators and seeking to minimise their effects, as bird behaviour may vary in field conditions and adaptive management is a key element for improving retrofitting. Those protocols should ideally be developed involving power suppliers, to make the obtained results comparable where problems caused by power lines to birds are homogeneous (CMS 2016). For every country, prototypes of strain insulators should be approved based on minimising risk to birds, particularly in priority areas.
Supplementary Materials
To view supplementary material for this article, please visit http://dx.doi.org/10.1017/S0959270921000253.
Acknowledgements
The present study was supported by the contract 17MNES001 “Encomienda de gestión para la evaluación de diversas tipologías de cadenas de amarre como zonas de posada de distintos grupos de rapaces” granted to the Tragsa Group by the General Directorate of Quality, Environmental Evaluation and Natural Environment from the Spanish Ministry for the Ecological Transition. We would like to acknowledge the collaboration of the WRC noted in the study, FIEB (Fundación para la Investigación en Etología y Biodiversidad), El Chaparrillo (WRC Ciudad Real), CERI (Centro de Estudios de Rapaces Ibéricas, Toledo), CRAS-Madrid (Centro de Recupración de Animales Silvestres, Madrid) and Los Hornos (WRC Cáceres) for lending their facilities and assisting us with the bird management. The manuscript was greatly improved by constructive comments from Steffen Oppel, Antoni Margalida and two anonymous referees, as did comments from J. M. Pérez-García and R. Moreno-Opo on earlier versions. All handling procedures were approved by the FIEB Ethics Committee (reference number CEEA FGC-04/2017) and performed in accordance with the Spanish Policy for Animal Protection RD 1201/2005, which conforms to European Union Directive 86/609 regarding the protection of animals used in scientific experiments.