1. Introduction
A fundamental question in child brain research is how different cortical regions develop their functional specificity for higher cognitive functions (Johnson, Reference Johnson2011). In the early stages of development, infants show left-lateralized brain activity for language processing (Dehaene-Lambertz, Hertz-Pannier, Dubois, Mériaux, Roche, Sigman & Dehaene, Reference Dehaene-Lambertz, Hertz-Pannier, Dubois, Mériaux, Roche, Sigman and Dehaene2006; Peña, Maki, Kovac̆ić, Dehaene-Lambertz, Koizumi, Bouquet & Mehler, Reference Peña, Maki, Kovac̆ić, Dehaene-Lambertz, Koizumi, Bouquet and Mehler2003), although some work suggests both infants and young children show a more widespread, and at times bilateral, response to language in comparison to adults (Holland, Vannest, Mecoli, Jacola, Tillema, Karunanayaka, Schmithorst, Yuan, Plante & Byars, Reference Holland, Vannest, Mecoli, Jacola, Tillema, Karunanayaka, Schmithorst, Yuan, Plante and Byars2007; Imada, Zhang, Cheour, Taulu, Ahonen & Kuhl, Reference Imada, Zhang, Cheour, Taulu, Ahonen and Kuhl2006; Nuñez, Dapretto, Katzir, Starr, Bramen, Kan, Bookheimer & Sowell, Reference Nuñez, Dapretto, Katzir, Starr, Bramen, Kan, Bookheimer and Sowell2011). It is generally accepted that such developmental differences are related to linguistic experiences, as well as to neural maturational processes (Garcia-Sierra, Rivera-Gaxiola, Percaccio, Conboy, Romo, Klarman, Ortiz & Kuhl, Reference Garcia-Sierra, Rivera-Gaxiola, Percaccio, Conboy, Romo, Klarman, Ortiz and Kuhl2011; Werker & Hensch, Reference Werker and Hensch2015); however, the precise nature of these dynamics is still not well understood. The present study examines the degree to which neural specialization for language, characterized by increasingly focal left-lateralized activation as monolinguals (e.g., Knoll, Obleser, Schipke, Friederici & Brauer, Reference Knoll, Obleser, Schipke, Friederici and Brauer2012), functions similarly in early Spanish–English bilinguals, and across both of their languages when receiving early and systematic dual-language exposure.
The Dual Language System Hypothesis of early bilingual acquisition holds that children exposed to two languages from birth undergo parallel and language-specific courses for each of their languages in the first years of life (Genesee, Reference Genesee1989; De Houwer, Reference De Houwer, Fletcher and MacWhinney1995; Du, Reference Du2010). Early language acquisition in many of the world's languages (e.g., English, German, Russian, Hebrew) is marked by an extended period of mastering verb finiteness, stemming from young children's inability to compute tense and agreement simultaneously (Wexler, Reference Wexler1998; Schütze & Wexler, Reference Schütze and Wexler1996), or from the ambiguity of the finite forms (Tomasello, Reference Tomasello2000). Of particular relevance to the present study are Spanish–English bilinguals: Children acquiring English may produce ‘bare’ verb errors, such as “He bake cookies” instead of either “He bakes cookies” or “He baked cookies” (3rd person singular in present/past tenses). While children acquiring a Romance language (e.g., Spanish, Italian) may produce overgeneralizations of 3rd person singular (Grinstead, Baron, Vega-Mendoza, De la Mora, Cantú-Sánchez & Flores, Reference Grinstead, Baron, Vega-Mendoza, De la Mora, Cantú-Sánchez and Flores2013; Grinstead, Lintz, Vega-Mendoza, De la Mora, Cantú-Sánchez & Flores-Avalos, Reference Grinstead, Lintz, Vega-Mendoza, De la Mora, Cantú-Sánchez and Flores-Avalos2014), omissions of noun plural inflections, and errors on gender markings, especially for direct object clitics (Bedore & Leonard, Reference Bedore and Leonard2001, Reference Bedore and Leonard2005; Dominguez, Reference Dominguez, Montrul and Ordoñez2003; Guasti, Reference Guasti1993, Reference Guasti2002; Pratt & Grinstead, Reference Pratt and Grinstead2007; Restrepo & Gutiérrez-Clellen, Reference Restrepo and Gutiérrez-Clellen2001). The acquisition of inflectional morphology in Spanish–English bilinguals is therefore language-specific and dependent on children's experiences in each of their languages (Peña, Bedore & Kester, Reference Peña, Bedore and Kester2015).
Neurolinguistic perspectives on (dual) language processing
Neurolinguistic theoretical perspectives suggest that language processing is supported by a dual-stream model: a ventral stream supporting sound to meaning mapping and a dorsal stream supporting auditory perception to motor production (Hickok & Poeppel, Reference Hickok and Poeppel2007; Friederici, Reference Friederici2011, Reference Friederici2012; Saur, Kreher, Schnell, Kümmerer, Kellmeyer, Vry, Umarova, Musso, Glauche, Abel, Huber, Rijntjes, Hennig & Weiller, Reference Saur, Kreher, Schnell, Kümmerer, Kellmeyer, Vry, Umarova, Musso, Glauche, Abel, Huber, Rijntjes, Hennig and Weiller2008). The dorsal stream is involved in morpho-syntactic processes during sentence comprehension, where the arcuate fascile (AF) and the superior longitudinal fascicle (SLF) transfer syntactic information between the left inferior frontal gyrus (IFG) and left temporal regions (Friederici, Reference Friederici2011, Reference Friederici2012; Skeide, Brauer & Friederici, Reference Skeide, Brauer and Friederici2016; Skeide & Friederici, Reference Skeide and Friederici2016). Specifically, left IFG supports both semantic and morpho-syntactic computations (BA 44 and BA 45 respectively), posterior left superior temporal gyrus (STG) supports access to morpho-phonemic forms, and the anterior left STG supports automated phrase structure computations (Friederici, Reference Friederici2011, Reference Friederici2012; Skeide & Friederici, Reference Skeide and Friederici2016). Functional magnetic resonance imaging (fMRI) studies reveal that adult-like cortical organization for morpho-syntactic processing involves robust brain activity in left IFG for complex sentences that include morphemic features acquired later in language development and are less frequent in language structures (such as case assignment), in comparison to morphemic features acquired earlier or structures that occur more frequently in speech (such as canonical word order) (Luke, Liu, Wai, Wan & Tan, Reference Luke, Liu, Wai, Wan and Tan2002; Knoll et al., Reference Knoll, Obleser, Schipke, Friederici and Brauer2012; Skeide et al., Reference Skeide, Brauer and Friederici2016; Skeide & Friederici, Reference Skeide and Friederici2016).
Young children (as early as 24-months) can detect morpho-syntactic violations such as –ing omission in English (e.g., He is go(ing) home) by showing a P600 event related potential (ERP) response within milliseconds of the errors (Oberecker & Friederici, Reference Oberecker and Friederici2006; see also Silva-Pereyra, Rivera-Gaxiola & Kuhl, Reference Silva-Pereyra, Rivera-Gaxiola and Kuhl2005). Nevertheless, their cortical response to language remains unadult-like until 9–10 years old (Skeide & Friederici, Reference Skeide and Friederici2016; see also Nuñez et al., Reference Nuñez, Dapretto, Katzir, Starr, Bramen, Kan, Bookheimer and Sowell2011). Research with monolingual children's pattern of cortical brain activity for morpho-syntactic structures suggests a protracted trajectory that initially involves both hemispheres as well as widely spread within the left hemisphere. As children's linguistic competence improves, their neural response to morpho-syntactic constructions becomes more specialized and focal within left-hemisphere regions (German-speaking monolingual children: Skeide & Friederici, Reference Skeide and Friederici2016).
Critically, multiple factors must be taken into account when considering potential sources of variability in children's neural specialization for language, including learning aptitude, language experience, and neuro-physiological brain maturation (particularly white matter tracts AF and SLF) (Nuñez et al., Reference Nuñez, Dapretto, Katzir, Starr, Bramen, Kan, Bookheimer and Sowell2011; Silva-Pereyra et al., Reference Silva-Pereyra, Rivera-Gaxiola and Kuhl2005; Skeide et al., Reference Skeide, Brauer and Friederici2016; Skeide & Friederici, Reference Skeide and Friederici2016). For instance, young monolingual German-speaking children with greater linguistic competence demonstrated stronger (more adult-like) left IFG brain activation for complex sentences with errors on morphemic structures than their lower-performing peers. In contrast, lower-performing peers showed left IFG brain activity for simpler sentences with errors on canonical sentence structures (Knoll et al., Reference Knoll, Obleser, Schipke, Friederici and Brauer2012). This developmental shift in the left IFG activation pattern reflects the regions’ processing effort allocated to acquiring and developing automaticity for high-frequency regularities of the given language (Knoll et al., Reference Knoll, Obleser, Schipke, Friederici and Brauer2012). Given these monolingual outcomes, one could therefore expect that early Spanish–English dual-language learners of similar age and language proficiency as their monolingual counterparts should show similar patterns of left IFG activation when processing later- versus earlier-acquired grammatical structures, and do so for each of their languages.
A more comprehensive representation of dual linguistic systems, suggesting their independency and interdependency, has been extensively outlined by the bilingual field (Cook, Reference Cook1991, Reference Cook2003; Roeper, Reference Roeper1999; Satterfield, Reference Satterfield1999; Vihman, Reference Vihman2002). Most recently, the ‘Integrated Multilingual Model’ of bilingualism offered an advancement by proposing that bilinguals can indeed become competent in each of their languages, while also recognizing that the two linguistic systems interact (MacSwan, Reference MacSwan2017). Of the many implications of this interaction, the essential claim is that as Grosjean (Reference Grosjean1989) suggests, bilinguals are “not two monolinguals in one brain.” Rather, features of bilinguals’ both languages interact and influence each other (phonologically, lexically, syntactically), thereby shaping the bilinguals’ minds and brains differently from those of monolinguals’ (Grosjean, Reference Grosjean1989; Kroll, Dussias, Bice & Perrotti, Reference Kroll, Dussias, Bice and Perrotti2015). This view gains support from behavioral research showing that in comparison to monolinguals, bilingual adults with childhood exposure to Spanish and English were more likely to attend to word order cues (a salient feature of English) when processing Spanish, but agreement cues (a salient feature of Spanish) figured prominently when processing English (Hernandez, Bates & Avila, Reference Hernandez, Bates and Avila1994). Building on this evidence, a study by Kovelman, Baker and Petitto (Reference Kovelman, Baker and Petitto2008) asked early and proficient Spanish–English bilingual adults to make plausibility judgments for sentences with varied syntactic complexity in English and Spanish, and found that bilinguals showed greater left IFG activation than monolinguals across all sentence types in English, suggesting a neurodevelopmental adaptation for acquiring and processing two linguistic systems (Kovelman et al., Reference Kovelman, Baker and Petitto2008). Thus, child bilinguals might also show evidence of greater left IFG recruitment, or other neuro-developmental differences in cortical specialization for language that reflect differences in morpho-syntactic processing between bilinguals and monolinguals (see also Parker-Jones, Green, Grogan, Pliatsikas, Filippopolitis, Ali, Lee, Ramsden, Gazarian, Prejawa, Seghier & Price, Reference Parker-Jones, Green, Grogan, Pliatsikas, Filippopolitis, Ali, Lee, Ramsden, Gazarian, Prejawa, Seghier and Price2012; Román, González, Ventura-Campos, Rodríguez-Pujadas, Sanjuán & Avila, Reference Román, González, Ventura-Campos, Rodríguez-Pujadas, Sanjuán and Avila2015).
The largest portion of knowledge on bilingual brain organization comes from research on adults presenting uniform effects of age-of-acquisition and proficiency (Costa & Sebastián-Gallés, Reference Costa and Sebastián-Gallés2014; Felton, Vazquez, Ramos-Nuñez, Greene, Macbeth, Hernandez & Chiarello, Reference Felton, Vazquez, Ramos-Nunez, Greene, Macbeth, Hernandez and Chiarello2017; García-Pentón, Pérez Fernández, Iturria-Medina, Gillon-Dowens & Carreiras, Reference García-Pentón, Perez Fernández, Iturria-Medina, Gillon-Dowens and Carreiras2014; Garcia-Sierra et al., Reference Garcia-Sierra, Rivera-Gaxiola, Percaccio, Conboy, Romo, Klarman, Ortiz and Kuhl2011; Klein, Mok, Chen & Watkins, Reference Klein, Mok, Chen and Watkins2014; Li, Legault & Litcofsky, Reference Li, Legault and Litcofsky2014; Mechelli, Crinion, Noppeney, O'Doherty, Ashburner, Frackowiak & Price, Reference Mechelli, Crinion, Noppeney, O'Doherty, Ashburner, Frackowiak and Price2004; Petitto, Berens, Kovelman, Dubins, Jasinska & Shalinsky, Reference Petitto, Berens, Kovelman, Dubins, Jasinska and Shalinsky2012). Studies on age of acquisition (AoA) suggest that learning a second language (L2) after the age of 5 results in reduced language proficiency and non-native patterns of second language organization in the brain (Berken, Gracco, Chen, Watkins, Baum, Callahan & Klein, Reference Berken, Gracco, Chen, Watkins, Baum, Callahan and Klein2015; Perani, Paulesu, Sebastián-Galles, Dupoux, Dehaene, Bettinardi, Cappa, Fazio & Mehler, Reference Perani, Paulesu, Sebastián-Gallés, Dupoux, Dehaene, Bettinardi, Cappa, Fazio and Mehler1998; Hernandez & Li, Reference Hernandez and Li2007; Jasinska & Petitto, Reference Jasinska and Petitto2013; see also Archila-Suerte, Zevin & Hernandez, Reference Archila-Suerte, Zevin and Hernandez2015). While there is some evidence to suggest that highly proficient bilinguals with a later age of acquisition can show native-like response to their second language, the studies that demonstrate this effect typically present speakers with single word recognition tasks, semantic judgment tasks, or whole text comprehension tasks (Chee, Tan & Thiel, Reference Chee, Tan and Thiel1999; Hasegawa, Carpenter & Just, Reference Hasegawa, Carpenter and Just2002; Vingerhoets, Van Borsel, Tesink, van den Noort, Deblaere, Seurinck, Vandemaele & Achten, Reference Vingerhoets, Van Borsel, Tesink, van den Noort, Deblaere, Seurinck, Vandemaele and Achten2003; Perani et al., Reference Perani, Paulesu, Sebastián-Gallés, Dupoux, Dehaene, Bettinardi, Cappa, Fazio and Mehler1998; Roncaglia-Denissen & Kotz, Reference Roncaglia-Denissen and Kotz2016). In contrast, studies that specifically target syntactic processes typically find evidence of AoA even in highly proficient late speakers of the language. For instance, Wartenburger, Heekeren, Abutalebi, Cappa, Villringer and Perani (Reference Wartenburger, Heekeren, Abutalebi, Cappa, Villringer and Perani2003) found reduced parietal activation in highly proficient late learners in their L2 (German), relative to their L1 (Spanish). At the same time, the majority of these studies was conducted with adult bilinguals and offers a retrospective view on the effects of bilingualism on adults’ neural organization for language. Thus, the question remains open as to the developmental processes underlying the effects of bilingualism on the neural organization for language in children who are in the process of developing syntax competence and automaticity as well as the neural specificity for syntactic processing.
The present studies
The present work examines the influence of early dual-language exposure on children's brain organization for processing inflectional morphology. To test this, we chose to use the experimental method of grammaticality judgments, since it fits several key criteria. First, grammaticality judgment tasks can uncover general patterns of language, whereas language production is coupled with environmental constraints (Phillips, Reference Phillips2009). For instance, Kweon and Bley-Vroman (Reference Kweon and Bley-Vroman2011) show that adults who produced ‘wanna’ violations in an elicited production task were actually sensitive to its constraints in parallel grammaticality judgment tasks. Second, while some work has used canonical sentence structures when testing children's judgments in grammaticality tasks (e.g., Jasinska & Petitto, Reference Jasinska and Petitto2013), we chose to test finiteness structures since morpho-syntactic features constitute a critical component of early language acquisition (Rice, Wexler & Hershberger, Reference Rice, Wexler and Hershberger1998). Third, children's proficiency in finiteness is typically operationalized through measures that include both elicitation and judgment procedures – this research finds that children's production of finiteness in obligatory contexts parallels their comprehension accuracy (Rice, Wexler & Redmond, Reference Rice, Wexler and Redmond1999). Critically, while typically-developing monolingual children reach adult-like accuracy in their production and judgment of finiteness, or simultaneous tense/agreement, by around age 6 (Rice, Wexler & Cleave, Reference Rice, Wexler and Cleave1995), the automaticity of these processes and their cortical organization continue to develop during the ages of 6–12, and possibly beyond (Skeide & Friederici, Reference Skeide and Friederici2016). Thus, grammaticality judgments of finiteness effectively tap into children's early language acquisition, and will allow us to situate the findings within the rich body of monolingual language acquisition and neurolinguistic literature that commonly uses grammaticality judgments tasks to inform our understanding of the impact of dual-language experiences on language acquisition.
In the present set of experiments, child participants underwent functional Near-Infrared Spectroscopy (fNIRS) neuroimaging covering bilateral frontal lobes, including inferior, middle and superior frontal regions (IFG, MFG, SFG). In Study 1, Spanish–English bilinguals and English monolinguals (ages 6–12) completed an English grammaticality judgment task, in which they heard well-structured sentences, sentences that violated the structure of relatively earlier-acquired elements of morpho-syntax in English (progressive -ing and “to be” agreement, as in “He was go to the store”), and later-acquired functional features of morpho-syntax (finiteness, or simultaneous tense/agreement as in “Yesterday he go to the store”). In Study 2, the same bilingual participants completed a comparable Spanish grammaticality judgment task, including sentences that violated relatively earlier-acquired elements of morpho-syntax in Spanish (subject-adjective gender disagreements across singular and plural subjects), and later-acquired functional features of morpho-syntax (finiteness, or simultaneous tense/agreement).
The present study contrasts two morpho-syntactic violations acquired at different times in language development. Children as young as 24-months elicit a P600 ERP response when hearing present participle –ing violations in sentences (Oberecker & Friederici, Reference Oberecker and Friederici2006). Yet English-speaking children still produce syntactic errors until age 5; specifically omissions in copular, present-tense 3rd person singular (–s) and past tense (–ed), likely stemming from children's inability to compute tense and agreement at the same time (Wexler, Reference Wexler1998). As a result, the acquisition of temporal syntactic markers is delayed and otherwise perceived as more ‘complex’ than that of the present participle –ing morpheme. Thus by setting up the earlier (easier) and later (harder) contrast as represented by –ing and –s/-ed markers, we evaluate both the emerging complexity of syntactic processing, through expressions of agreement and tense, as well as the bilingual child's maturing linguistic competence that gradually comes to rely upon the syntactic system. By manipulating the developmental time course of morphemic features into the experimental conditions, it allows us to control for some ‘violation-general’ aspects of cognition and to better isolate key aspects of morpho-syntactic processing.
To advance our understanding of how early bilingual experience influences children's neural specialization for language function, we have built our hypotheses upon the Integrated Multilingual Model (MacSwan, Reference MacSwan2017), leading to the prediction that early dual-language learners will show greater left IFG response to later- versus earlier-acquired morpho-syntactic features of English, as compared to English monolinguals (English: Study 1), and in both of their languages (Spanish: Study 2). We claim that these outcomes will demonstrate that Spanish–English bilinguals form neural indexes of morpho-syntactic competence specific to each of their languages, and do so in a way that is similar to monolinguals. We further predict that young bilinguals also present a ‘neural signature’ of early dual language processing, such as greater left IFG activation relative to monolinguals, as previously reported for adult studies (Kovelman et al., Reference Kovelman, Baker and Petitto2008) – suggesting that dual language experience is associated with neurodevelopmental adjustments for accommodating the learning of the two language systems.
2. Study 1: English morpho-syntactic processing in monolingual and bilingual children
2.1 Methods
Participants
Thirty-nine neurotypical children, with no history of developmental, learning, or hearing deficits took part in the study: 21 Spanish–English speaking bilinguals (8 females, 13 males; age range = 6.8 – 12.4 years, mean age [Mage] = 10.09, standard deviation [SD] = 1.50) and 18 English speaking monolinguals (9 females, 9 males; age range = 6.6 – 13.6 years, Mage = 9.64, SD = 1.75). Selection criteria for bilingual participants were as follows: Spanish exposure from birth, English exposure prior to age 5, which is the period demonstrating that children can be classified as ‘bilingual L1s’ where they are simultaneously acquiring two first languages; a 3 years minimum of English exposure prior to testing, adequate dual-language competence including morpho-syntactic abilities (at least 60% accuracy) as assessed by the Word Structure subtest in the Clinical Evaluation of Language Fundamentals (CELF-4; Semel, Wiig & Secord, Reference Semel, Wiig and Secord2003, Reference Semel, Wiig and Secord2006) and a standard score above 85 in English and Spanish receptive vocabulary abilities as assessed by the Kaufman Brief Intelligence Test (KBIT-2) Verbal Knowledge subtest (Kaufman & Kaufman, Reference Kaufman and Kaufman2004) and Receptive One-Word Picture Vocabulary Test Spanish Bilingual Edition (ROWPVT-4; Brownell, Reference Brownell2000).
Bilingual families were invited to two experimental sessions within a 5 week interval: one in English (Study 1) and one in Spanish (Study 2), with language order counterbalanced across participants. Of the total sample, three bilinguals completed only the Spanish language visit (Study 2), and 3 bilinguals’ English data exceeded signal-to-noise ration criterion (see fNIRS methods below). The final sample included 15 bilinguals (6 females, 9 males; age range = 6.9 – 12.4 years, M = 10.7, SD = 1.3) and all 18 monolingual participants (see above for sample details).
Parents of monolingual children reported their child's use of English as the primary language at home and at school. Parents of bilingual children reported their child's continued and systematic daily use of both of languages, with Spanish in the home and English at school and in the broader community. Six (40%) bilingual children were born outside of the U.S. in a Spanish-speaking country. All parents, with the exception of one father, were native Spanish speakers and reported consistent use of Spanish with their children. All bilingual children were first exposed to Spanish at birth and to English between birth and age 4 (M = 2.11, SD = 1.84), and had obtained a minimum of 3 years of English exposure at the time of testing (M = 8.22, SD = 2.70). Eleven (73%) bilinguals were also attending a local Spanish heritage language school once a week and completed Spanish language homework assignments for the school during the week.
Participating families, bilingual and monolingual, were recruited from the same neighborhoods in southeast Michigan (United States, U.S.), covering the same school districts with English-only schools. According to data from the World Bank (2017), both groups of families would be classified as middle-income, yet monolingual families had a higher socio-economic background than the bilingual families (see Table 1 for more details). Children were right-handed (except one bilingual child who was left-handed, yet their imaging data did not differ from other children in the bilingual group and was thus included in analyses). The studies were approved by institutional review boards; parents and children completed respective informed consent and assent forms. Families received monetary compensation and children received a small toy (e.g., a frisbee) as a thank you gift.
Table 1. Monolingual and Bilingual participants’ Mean (and Standard Deviation) Scores.

Notes. * p < 0.05 **p < 0.01
Scores for IQ and vocabulary are standardized at a mean of 100 (typical average scores range between 85 and 115); Scores for phonological awareness and memory are reported as percentage scores (%).
a Options for demographic responses on yearly household income were the following: (1) less than $5,000; (2) $5,000 - $11,999; (3) $12,000 - $15,999; (4) $16,000 - $24,999; (5) $25,000 - $34,999; (6) $35,000 - $49,999; (7) 50,000 - $74,999; (8) $75,000 - $99,999; (9) $100,000 and greater. Three sets of parents (2 in the monolingual group and 1 in the bilingual group) did not respond this question.
b Options for responses on education were the following: (1) primary school, (2) some secondary school, (3) High school diploma or equivalent (GED), (4) some college, (5) Associate's degree, (6) Bachelor's degree, (7) Master's degree, (8) Doctorate degree [Ph.D], (9) Professional degree [MD, DD, DDS, etc]. One set of parents in the monolingual group did not respond to this question.
Measures of language, literacy, and cognitive development
Parents completed a detailed Language Background and Use questionnaire (LBU; Kovelman et al., Reference Kovelman, Baker and Petitto2008) about their child's cognitive, language and motor development, plus any family history of learning impairments. Parents also completed questions on their educational level and household income from the John D. and Catherine T. MacArthur Foundation Research Network on Socioeconomic Status and Health questionnaire (retrieved from: www.macses.ucsf.edu).
Phonological awareness
In English, children completed the Elision subtest from the Comprehensive Test of Phonological Processing (CTOPP; Wagner, Torgesen & Rashotte, Reference Wagner, Torgesen and Rashotte1999). During testing, the experimenter asked the child to say a word, and then to repeat it without saying a portion of it. For example, “Say winter, now say winter without saying /t/,” the correct response is “winner.” Similarly, in Spanish, the children completed Elision subtest from the Test of Phonological Processing in Spanish (TOPPS) (Francis, Carlo, August, Kenyon, Malabonga, Caglarcan & Louguit, Reference Francis, Carlo, August, Kenyon, Malabonga, Caglarcan and Louguit2001). Participants earned 1 point for correct items; the task included 6 practice items and 20 testing items. Testing began on the first item and stopped when the ceiling item was reached (or 3 consecutive errors). Percentage scores (out of a total of 20 items) are reported.
Phonological short-term memory
In English, children completed the CTOPP Nonword subtest (Wagner et al., Reference Wagner, Torgesen and Rashotte1999). The experimenter played recordings of made-up words and asked the participant to repeat it as he/she heard it, for example, “nigong;” non-words ranged in length from 3 to 15 phonemic sounds, 3 practice items and 18 testing items were presented. Similarly, in Spanish, children completed the TOPPS Nonword subtest (Francis et al., Reference Francis, Carlo, August, Kenyon, Malabonga, Caglarcan and Louguit2001). Testing stopped when ceiling level was reached (3 consecutive errors); percentage scores (out of a total of 18 items) are reported.
Vocabulary
In English, children completed the KBIT-2 Verbal Knowledge subtest (Kaufman & Kaufman, Reference Kaufman and Kaufman2004). During testing, the experimenter presented the child with a matrix of 6 images and a question or a word, the participant pointed to the best representing picture. In Spanish, the children completed the ROWPVT-4 Spanish Bilingual Edition (Brownell, Reference Brownell2000), which is a standardized assessment normed with Spanish–English bilinguals. Similar to the English Vocabulary assessment, the experimenter presented the child with a matrix of 4 images and a word. Basal and ceiling levels were established for both measures; standard scores (M = 100, SD = 15) are reported.
Morpho-syntax
CELF-4 Word Structure subtest (Semel et al., Reference Semel, Wiig and Secord2003, Reference Semel, Wiig and Secord2006) was used to assess morpho-syntax in both languages. The assessments measure participants’ ability to apply morphology rules and appropriate pronouns. The English measure included 32 items and the Spanish measure included 29 items; percentage scores are reported.
Non-verbal intelligence
KBIT-2 Matrices subtest (Kaufman & Kaufman, Reference Kaufman and Kaufman2004), which measures the ability to find spatial and abstract relationships among a set of images and patterns, was used to assess non-verbal IQ. During testing, children selected the missing piece in a ‘puzzle’ (out of 4 options). Basal and ceiling levels were established; standard scores (M = 100, SD = 15) are reported.
English neuroimaging measure: Auditory grammaticality judgment task
The task was modeled after the comprehension portion of the Test of Early Grammatical Impairment (TEGI; Rice & Wexler, Reference Rice and Wexler2001; Rice et al., Reference Rice, Wexler and Redmond1999). Children heard correctly structured sentences, sentences with errors on early-acquired morphemes, and sentences with errors on later-acquired morphemes. During sentences with errors on Later acquired morphemic features, children heard sentences with 3 types of errors: past-tense omission (regular or irregular verbs; e.g., “Yesterday, he try to win” or “Yesterday, he eat supper”), present-tense 3rd person singular omission (e.g., “She always copy her brother”), and copular omissions before adjectival and prepositional phrases (e.g., “We very wet and cold” or “She behind the yellow door”). During sentences with errors on Early-acquired morphemic features, children heard sentences that are generally judged ungrammatical by children (Rice et al., Reference Rice, Wexler and Redmond1999), including: present participle “-ing” omission (e.g., “He is wash the car”) and subject/verb agreement errors (e.g., “Dad are washing the car”). During Correct sentences, children heard well-structured sentences that were similar to the sentences in the Early and Later acquired morpheme conditions, including: past tense (e.g., “Last week, I saw Dad”), 3rd person singular (e.g., “She always copies my answers”), copular (e.g., “We are outside the tent”), use of -ing (e.g., “He is helping his brother”), and use of agreement (e.g., “Dad is washing the car”).
Stimuli consisted of five-word sentences matched across conditions for verb and noun, age of acquisition, written frequency, concreteness, imageability, and familiarity (data from MRC Psycholinguistic database, Coltheart, Reference Coltheart1981; one-way ANOVA, ps > 0.05 within each condition; ad-hoc t-tests comparing the conditions were also non-significant, ps > 0.05). The sentences were all recorded by the same adult female native speaker of American English and were equalized for RMS amplitude using Praat (Boersma & Weenink, Reference Boersma and Weenink2008). Average sentence duration was 1.61s (SD = 0.16).
The task was an event-related design with a total of 20 trials per condition (randomized using OptSeq2; Dale, Reference Dale1999). During each trial, children saw a cartoon image of an alien for 4-seconds at the onset of the sentence, followed by a 2-second display of a question mark in the center of the screen that allowed additional time for the participant to respond. The task contained 25% Correct sentences, 25% Early morpheme errors, 25% Later morpheme errors, and 25% jittered Rest periods. During the jittered Rest periods, participants saw a fixation cross in the center of the screen. The task lasted ~7.5 minutes and was presented using E-Prime 2 (Psychology Software Tools, Inc.) on a 23-inch Philips 230E Wide LCD screen connected to a Dell Optiplex 780 desktop computer; sound played via two Creative Inspire T12 2.0 multimedia speakers. A two-button response box (Current Designs, Inc.) was connected to the desktop computer to record participants’ responses. Trials were deemed incorrect if the participant pressed the incorrect button, or did not respond. Performance was assessed by accuracy and response time.
Procedure
Following an initial phone screening, participants were invited for a testing session to a child-friendly fNIRS brain-imaging laboratory. During the present study, children interacted solely with English-speaking experimenters. Following the consenting procedure, participants first underwent fNIRS brain imaging and then completed assessments of English language and cognition. During this fNIRS session, participants also completed two unrelated measures of cognition. The session lasted up to 2 hours with short breaks in-between.
Experimenters first set the fNIRS cap and optodes in place, and pictures of the probe placement were taken. Prior to completing the neuroimaging task, children were told a story about an alien that was learning to speak English and needed help learning when he was making a mistake. Children were instructed to help the alien by pressing buttons in a button-box: if a sentence was correct and did not have any mistakes, children used their right hand to press the right button, and, if the sentence had mistakes, children used their left hand to press the left button. Children were instructed to respond as quickly and as accurately as possible, without waiting for the question mark to appear if they knew the answer before then. A brief practice consisting of six sentences (which were not used during testing) was administered. During practice trials, the experimenter provided feedback; however, no feedback was provided during testing.
Functional NIRS data acquisition, processing, and analyses
The study used a TechEN-CW6 system with 690 and 830 nm wavelengths. The set-up included 4 emitters of near-infrared light and 12 detectors spaced ~2.7 cm apart, yielding 16 data channels sampled at 10 Hz (8 channels per hemisphere; see Figure 1). Sensors were mounted into a custom-built head cap constructed from polyester cloth, with attached grommets to hold the emitters and detectors during data collection. The probes covered bilateral prefrontal cortex including IFG, MFG and SFG. The probe localization was established and applied consistently for each participant using the international 10-10 transcranial system positioning (Jurcak, Tsuzuki & Dan, Reference Jurcak, Tsuzuki and Dan2007); Inion, Nasion, Fz, FpZ, Cz, auricular left and right, and F7/8 were measured for each participant, and the two lower bilateral sources were anchored at F7 and F8.

Figure 1. Functional NIRS probe configuration. In order to visualize and estimate the brain regions maximally covered by the channels, we estimated approximate MNI coordinates using the geometric structure of our measurement setting for the 16 optodes (emitters and detectors). We used reference points to equally distribute 1,000 points along the distance of each channel (between each source and detector pair). The voxel points were the distance partitioned to 1,000 sections for a distance of ~2.7cm on a 3D image brain template provided by https://irc.cchmc.org/software/pedbrain.php. The corresponding brain regions (Brodmann areas, BA) were then estimated using xjView database (http://www.alivelearn.net/xjview) in MATLAB. The brain areas covered by the 1000 points distributed along each channel are recognized as the brain areas covered by that channel. If a channel covered more than one area, the area indices were arranged in sequence according to the proportion of the 1000 points falling within the given areas. We used this brain template with interpolated optodes and the “patch” function in MATLAB to generate 3D images to display the imaging results. (A) Dots correspond to optode placements at a distance of ~2.7 cm, over an average brain template (blue = sources of light; green = detectors; black = approximate area of the brain covered by the fNIRS measurement). (B) Probe-set and channel configuration for right and left hemispheres, respectively. (C) Brain regions maximally overlaid by the probe arrangement in the order of greatest probability for each channel (BA = Brodmann Area).
Data preprocessing was completed using MATLAB-based software, including Homer2 software retrieved from the NITRC database (Huppert, Diamond, Franceschini & Boas, Reference Huppert, Diamond, Franceschini and Boas2009), NIRS Toolbox (Huppert & Barker, Reference Huppert and Barker2015), and several customized scripts (Hu, Hong, Ge & Jeong, Reference Hu, Hong, Ge and Jeong2010). First, data visualization was done using Homer2, in which the 690 and 830nm wavelengths timeseries data were examined to exclude participants whose signal quality was below 3 molar units and did not reveal cardiac signal for over 50% of 690 data channels, likely due to a large amount of motion artifacts and/or hair obstruction (3 bilinguals).
Using NIRS Toolbox, raw time course data was converted into units of optical density change (ΔOD). Using the modified Beer-Lambert law, we converted the data to hemoglobin concentration signal change yielding oxygenated hemoglobin (HbO) and deoxygenated hemoglobin (HbR) values. In order to correct for motion artifacts and serial correlations, we carried out a pre-whitening filter on participants’ hemoglobin data time series. At the first-level, each participant's hemoglobin concentration data was analyzed using an autoregressive general linear model that assumed the dual-gamma canonical hemodynamic response function (Friston, Ashburner, Kiebel, Nichols & Penny, Reference Friston, Ashburner, Kiebel, Nichols and Penny2006). The first-level GLM analysis estimated beta values, which are indices of percent signal change, for each condition and each participant: errors of Early morphemic features, errors of Later morphemic features, and Correct sentences. Second-level group analyses were conducted using a second-level GLM that included conditions and groups (monolingual, bilingual) as fixed effects, participants were treated as a random effect variable, and hemoglobin beta values (HbO and HbR) as the predicting dependent variables. HbR analyses are reported in Table S1 (Supplementary Materials). The regressions estimated betas for each condition (Later, Early, and Corrects), as well as the following contrasts: Later > Early, Early > Later, Later > Corrects, Corrects > Later, Early > Corrects, and Corrects > Early. Within-group condition comparisons aimed to identify positive activations for each of the groups, and between-group comparisons aimed to identify group differences; both types of analyses were carried out using two-tailed t-tests with an alpha-threshold of p < 0.05.
2.2 Results
Task performance and language abilities are reported in Table 1 for the entire group of bilinguals and monolinguals, including participants whose neuroimaging data did not pass the criterion thresholds. The children did not differ in age, IQ, English language abilities or cognitive abilities (p > .05; see Table 1). Families from monolingual children were from significantly higher socio-economic backgrounds than bilingual children, as measured by household income and parents’ education. Comparisons between bilingual children's English and Spanish language abilities revealed that most language scores were comparable; however, bilingual children performed significantly better during the English than Spanish morpho-syntax assessment, as would be expected of children in English-dominant educational environments (see Table 1).
English grammaticality judgment task performance
A linear mixed-effects model in IBM SPSS Statistics 24 was performed to examine accuracy task performance, group and condition factors were treated as fixed effects and participants were specified as a random factor. The random intercept model was centered at the bilingual group and Correct sentences condition. The model of best fit was selected using Akaike's information criterion (AIC) goodness-of-fit index where the smallest AIC value was preferred (-286.09). The model revealed a significant effect of condition in sentence type (F(2, 46.52) = 20.16, p < .001) that stemmed from participants’ better performance in Corrects than sentences with errors of Later-acquired morphemic features (β = −8.37%, SE = 2.20, t = −3.80, p < .001), but not sentences with errors of Early-acquired morphemic features (β = 1.05%, SE = 1.17, t = 0.90, p = .373); see Table 1. We did not find a significant effect of language group (F(1, 42.96) = 0.68, p = .796), or a language group by sentence condition-type interaction (F(2, 46.52) = 0.31, p = .734).
A similar linear mixed-effects model was performed to examine response time task performance, group and condition factors were treated as fixed effects and participants were specified as a random factor. The random intercept model was centered at the bilingual group and Correct sentences condition. The model of best fit was selected using Akaike's information criterion (AIC) goodness-of-fit index (1320.67). The model revealed a significant effect of condition in sentence type (F(2, 31.53) = 39.16, p < .001) that stemmed from participants’ faster performance in sentences with errors of Earlier-acquired morphemic features than Corrects (β = −293.15 ms, SE = 66.79, t = −4.39, p < .001), but not sentences with errors of Later-acquired morphemic features (β = 47.40 ms, SE = 59.74, t = 0.79, p = .433); see Table 1. We did not find a significant effect of language group (F(1, 30.37) = 2.87, p = .10), or a language group by sentence condition-type interaction (F(2, 31.53) = 0.18, p = .83).
Functional neuroimaging data results: Within-group comparisons
During the Later > Early contrast (see Figure 2A and 4A), monolinguals activated left channel 4, right channels 1 and 4–6, while bilinguals activated left channel 1. The reverse contrast Early > Later did not yield significant activity for either group. Within-group contrasts comparing Later-acquired errors and correct sentences (see Figure 2B; Later > Corrects and Corrects > Later) also did not reveal significant activity for either monolingual or bilingual groups. During Corrects > Early (see Figure 2C), monolinguals activated right channels 1–2 and 4–6, while bilinguals activated right channels 4 and 7. The reverse contrast Early > Corrects did not reveal significant activity for either group.

Figure 2. Oxygenated hemoglobin beta values (as percent of signal change for contrasts) during the English grammaticality judgment task. Within-group brain activation for bilinguals and monolinguals during sentences with (A) errors on Later-acquired morpho-syntactic features relative to Early-acquired, (B) errors on Later-acquired relative to Correct sentences, and (C) Correct sentences relative to Early-acquired morpho-syntactic features.

Figure 3. Between-group comparison for the English grammaticality judgment task among bilinguals’ and monolinguals’ brain activation during (A) errors on Later-acquired morpho-syntactic features relative to Early-acquired, and (B) Later-acquired relative to Correct sentences.
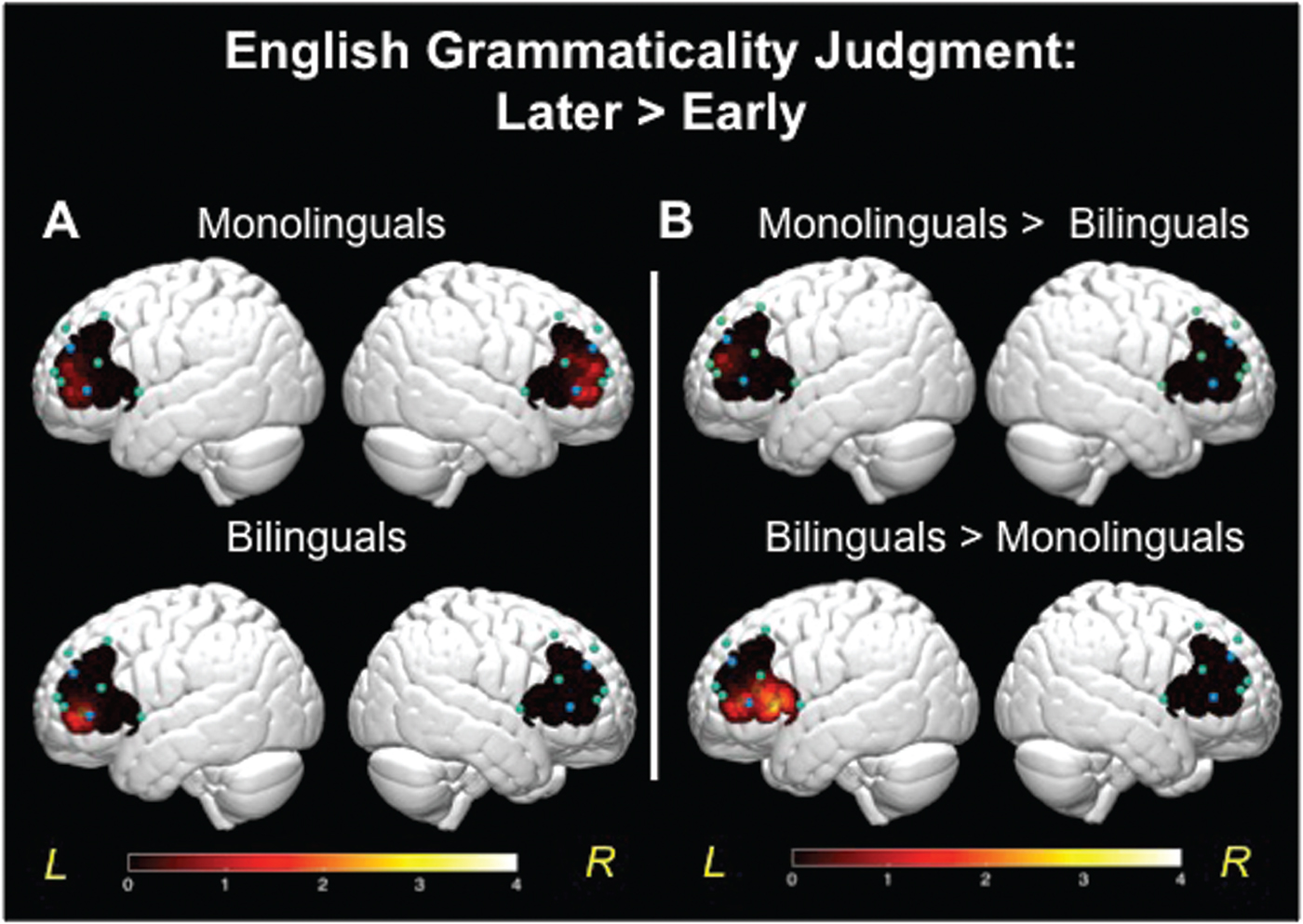
Figure 4. 3-D brain images. (A) T-values mapped for comparison of brain activation for monolinguals (top row) and bilinguals (bottom row). Higher values on the scale indicate greater brain activity during the sentences with errors on Later-acquired morphosyntax, relative to errors on Early-acquired. (B) T-value map for comparison of brain activation between bilinguals and monolinguals: top figure shows greater activity for monolinguals, bottom figure shows greater activity for bilinguals. The color bar reflects significant t-values.
Functional neuroimaging data results: Between-group comparisons
See Figure 3. The between-group comparison for Later > Early revealed that monolinguals had greater activation in left channel 4 relative to bilinguals, while bilinguals showed greater activation in left channels 1–3 relative to monolinguals (see Figure 3A and 4B). As shown in Figure S1 (Supplementary Materials), the double-subtraction group differences for the Later > Earlier errors contrast stemmed from both conditions, the bilinguals had more activation during the Later condition and less activation during the Early condition. The between-group comparison for Later > Corrects (Figure 3B) revealed that monolinguals had greater activation in right channel 4 relative to bilinguals. While bilinguals showed greater activation in left channels 1–2, as well as right channels 2–3 and 5, relative to monolinguals. As shown in Figure S1, group differences in channels 1–2 stem from bilinguals’ stronger activation during the Later-acquired morphemic features condition than Correct sentences, while monolinguals had similar levels of activation for the two. The between-group comparison for remaining contrasts including Early > Later, Corrects > Later, Early > Corrects, and Corrects > Early did not reveal significant differences between monolinguals and bilinguals in brain activity.
3. Study 2: Spanish morpho-syntactic processing in bilingual children
Study 2 was designed as a companion to Study 1, to investigate whether the impact of early bilingualism on neural specialization for language processing was specific to morpho-syntactic error detection in one or both languages. Since bilinguals in the present sample were more competent in English than Spanish, it also allows the examination of whether any differences in brain activity are due to language abilities. Importantly, this notion is uniquely tested within the bilingual group rather than contrasting to monolinguals whose linguistic and socio-cultural experiences vary from bilinguals growing up in the United States. Study 2 tested the same group of Spanish–English bilinguals in a Spanish grammaticality judgment task. If bilingual children show a pattern of brain activity that is more widespread (less specialized, e.g., more bilateral) for their Spanish than English, then this would suggest that children's brain activity is likely driven by language abilities. In contrast, if bilingual children show similar levels of specialization for the two languages, then this would suggest that children's neural specialization for language is also driven by the combined dual-language experiences. In such a case, evidence for the latter hypothesis would support the notion that the ‘neural signature’ of bilingual language experiences can modulate the neurodevelopmental course of syntactic processing and organization in the young brain, and it is quantitatively different from that of monolinguals.
3.1 Methods
Participants
The present study includes data from 18 Spanish–English bilinguals who fit the same selection criteria as the children in Study 1, (8 females, 10 males; age range = 8.4 – 11.8 years, mean age [M] = 10, standard deviation [SD] = 1.13). The children completed the language, literacy, and cognitive assessments listed in Study 1.
Spanish auditory grammaticality judgment imaging task
Similar to the English task in Study 1, the children completed a Spanish grammaticality judgment task with the same number of sentences (items) containing violations of Early- and Later-acquired inflectional morphemes as well as correct sentences. Later-acquired condition included erroneous use of 3rd person singular instead of obligatory 1st or 2nd person singular past and present forms (e.g., 1st person present tense “Yo junta monedas amarillas [I collects yellow coins],” 2nd person past tense “Ayer, tú peino las muñecas [Yesterday, you comb (hair of) the dolls],” 3rd person present tense “El niño dibujo una casa [The boy draw a house]”). The Early-acquired condition included errors of subject-adjective gender agreement for singular and plural subject forms (e.g., female and male singular “La flor blanco es para mamá [The white flower is for mom]”, “El perro cansadas tiene sed [The tired dog was thirsty]”, female and male plural “Los gatos pequeñas toman leche [The small cats drink milk]”, “La taza llenos tiene café [The full cup has coffee]”). During Correct sentences, children heard well-structured sentences, including correct 1st and 2nd person assignment (e.g., “A diario, yo toco el piano [Everyday, I play the piano]” ,“Ayer, tú sacaste la basura [Yesterday, you took out the trash]”), as well as subject-adjective gender agreements (e.g., “La gata negra asustó a mamá [the black cat scared mom]” ,“Los lápices nuevos están en casa [the new pencils are at home]”).
Procedure, data collection and analyses
The testing protocol and procedure were similar to Study 1, with the exception that during the Spanish/Study 2 visit, the experimenters only used Spanish to interact with the children. Similar to Study 1, the analyses were carried out using two-tailed t-tests with an alpha-threshold of p < 0.05.
3.2 Results
Spanish grammaticality judgment task performance
A linear mixed-effects model in IBM SPSS Statistics 24 was performed to examine accuracy task performance, condition sentence types were treated as a fixed effect and participants were specified as a random factor. The random intercept model was centered at the Correct sentences condition. The model of best fit was selected using Akaike's information criterion (AIC) goodness-of-fit index where the smallest AIC value was preferred (-24.44). The model did not reveal a significant effect of condition in sentence type (F(2, 39.51) = 2.56, p = .09). A similar linear mixed-effects model was performed to examine response time task performance; the model of best fit was selected using Akaike's information criterion (AIC) goodness-of-fit index (842.60). The model did not reveal a significant effect of condition in sentence type (F(2, 35.77) = .04, p = .96). See Table 1 for task performance.
Functional neuroimaging data results
See Figure S2 (Supplementary Materials) for estimated betas for each condition. During the Later condition, children activated left channels 1, 4–8, and right channels 4–8. During the Early condition, children activated left channels 7–8 and right channels 1–3 and 8. During Corrects, children activated right channels 1–2 and 6.
During the Later > Early contrast, children activated left channels 1 and 4 (Figure 5A and Figure 6). The reverse contrast Early > Later did not yield significant activity. During the Later > Corrects contrast, children activated left channels 1 and 4, as well as right channels 4 and 8 (Figure 5B). The reverse contrast Corrects > Later revealed children activated right channel 2 (Figure 5C). The Early > Corrects and Corrects > Early contrasts did not yield significant activity.

Figure 5. Bilinguals’ oxygenated hemoglobin beta values (as percent of signal change for contrasts) during the Spanish grammaticality judgment task. Within-group brain activation during sentences with (A) errors on Later-acquired morpho-syntactic features relative to Early-acquired, (B) errors on Later-acquired relative to Correct sentences, and (C) Correct sentences relative to Early-acquired morpho-syntactic features.

Figure 6. 3-D brain image. Significant t-values mapped for comparison of brain activation during the Spanish grammaticality judgment task. Presented here is the contrast between errors on Later-acquired morphosyntactic features relative to errors on Early-acquired. Higher values on the scale indicate greater brain activity.
4. Discussion
The study investigated how early and systematic bilingual exposure influences children's functional organization for syntactic processing. The Integrated Multilingual Perspective (MacSwan, Reference MacSwan2017) on bilingual processing advances the notion that young bilinguals learn each of their languages in a monolingual-like manner, yet both languages interact, and develop neurocognitive mechanisms to support their interactive dual-language processes (see also Grosjean, Reference Grosjean1989; Cook, Reference Cook1991, Reference Cook2003). The left IFG region is critical to the development of inflectional morphology processing, where complex morpho-syntactic features recruit greater activity (Friederici, Reference Friederici2011, Reference Friederici2012). Thus, we predicted greater left IFG activation for later- versus earlier-acquired aspects of morpho-syntax. We hypothesized that bilinguals’ frontal lobe cortical specialization for language should correspond directly to their language abilities, predicting that, since we tested highly competent bilinguals, their brain activation patterns for both languages should not differ substantially from monolinguals. The findings confirm this prediction: Spanish–English bilinguals and English monolinguals in the present study had similar English language abilities, experimental task accuracy, and greater left IFG activation for later- versus earlier-acquired aspects of morpho-syntax.
Furthermore, while bilingual children demonstrated greater competence in English than Spanish, they also showed greater left frontal activation for later- versus earlier acquired aspects of morpho-syntax in Spanish, in regions similar to those of English activation where bilinguals showed greater activity than monolinguals. These results are consistent with our second exploratory hypothesis that young bilinguals present evidence of early neurodevelopmental adaptation to bilingualism by showing overall greater left IFG activation than monolinguals, and to both of their languages although their language abilities were greater for English than Spanish. Taken together, the findings suggest that early bilingual learners can achieve language competence comparable to monolinguals, neural response specificity in left IFG regions is shown to be similar across their two languages, and stronger in bilinguals relative to monolinguals. The findings advance the proposed theoretical perspectives of bilingual acquisition by revealing fundamental similarities between bilingual and monolingual children's neurocognitive bases for inflectional morphology at the core of their language systems. The findings also advance theoretical perspectives that link enriched linguistic experiences with more advanced neural specialization for language processing (Raizada, Richards, Meltzoff & Kuhl, Reference Raizada, Richards, Meltzoff and Kuhl2008).
Bilinguals demonstrated task performance equivalent to monolinguals as well as greater activation for the later- than earlier-acquired morpho-syntactic structures of tense and agreement. Notably, the participant groups were comparable in age, English language abilities, and IQ. Nevertheless, the differences between the two syntactic conditions were more pronounced in bilinguals’ left IFG region (Figure 3), while monolinguals exhibited a more distributed and bilateral response. Prior work has shown that, as sentence complexity increases, the engagement of right frontal regions also increases – which is often attributed to the increase in generalized working memory and attention demands (Just, Carpenter, Keller, Eddy & Thulborn, Reference Just, Carpenter, Keller, Eddy and Thulborn1996; Caplan, Alpert & Waters, Reference Caplan, Alpert and Waters1998; Keller, Carpenter & Just, Reference Keller, Carpenter and Just2001). Individuals with greater language abilities (e.g., larger vocabulary) often show less distributed neural activity in comparison to those with lower language abilities (Knoll et al., Reference Knoll, Obleser, Schipke, Friederici and Brauer2012; Raizada et al., Reference Raizada, Richards, Meltzoff and Kuhl2008; see also Prat, Keller & Just, Reference Prat, Keller and Just2007), suggesting more efficient use of language-specific neural resources in those with better linguistic competence (Prat & Just, Reference Prat and Just2010). Yet functionality for morpho-syntactic features develop slowly with age, even 9-year-old children recruit broad left frontal activity, as compared to adults, even when highly accurate in detecting morpho-syntactic errors (Skeide et al., Reference Skeide, Brauer and Friederici2016). Monolingual children in the present results support previous findings showing monolingual adults activate bilateral frontal regions when listening to complex sentences (Just et al., Reference Just, Carpenter, Keller, Eddy and Thulborn1996; Caplan et al., Reference Caplan, Alpert and Waters1998; Keller et al., Reference Keller, Carpenter and Just2001). As can be seen in Figure 5, bilinguals’ greater activation for later-acquired structures, and lower activation to earlier-acquired structures, is generally limited to left IFG activation – in English (Study 1) and in Spanish (Study 2), and supports the notion that early bilingual exposure paves the way for language-specific acquisition and high-level language competency. In sum, bilinguals show a reduced spread of activation as compared to monolinguals, suggestive of better specialization of neural response to syntax in left IFG – the key region for syntax acquisition and processing (Knoll et al., Reference Knoll, Obleser, Schipke, Friederici and Brauer2012).
Two interrelated explanations for these effects surface. First, this specialization may be the advantageous result of an overall linguistic enrichment provided by early dual-language experiences. For instance, research finds greater left IFG activation during a rhyme versus tone judgment task in children from higher-income families, which is typically associated with more enriched linguistic input from caregivers (Raizada et al., Reference Raizada, Richards, Meltzoff and Kuhl2008). Moreover, research often finds that bilingual children have higher sensitivity to linguistic structures, as reflected in being better learners of new artificial languages as compared to monolinguals (Kuo & Anderson, Reference Kuo and Anderson2010). This result likely stems from the need to consider a greater number of possible interpretations for sounds, meanings, and structures across two linguistic systems from early life, thereby heightening children's structural sensitivity to language (Kuo & Anderson, Reference Kuo and Anderson2010). Left frontal lobe regions are known to support the computation of linguistic structures as well as children's ability to alternate between their two languages, the mechanism that likely builds upon any language users’ ability to alternate between language registers (home language, school language etc.). Therefore, if one were to consider bilingual and monolingual states along a continuum of enriched linguistic experiences in which children consider more diverse language and language register usage (MacSwan, Reference MacSwan2017; Grosjean, Reference Grosjean1989; Cook, Reference Cook1991, Reference Cook2003), the present findings further support the idea that cortical specialization for language varies as a function of children's overall linguistic experiences.
The results did not shed light on significant differences between children's language competence (vocabulary, syntax, or grammaticality judgment task performance), linguistic input (as measured through questionnaires), and brain function. The findings are nonetheless commensurate with previous research showing that while AoA and length of exposure play a significant role in language competence as well as bilinguals’ brain organization in general, these effects are smaller for those with early and systematic bilingual exposure to English within the United States immigration context (prior to age 9: Archila-Suerte et al., Reference Archila-Suerte, Zevin and Hernandez2015; prior to age 4: Bedore, Peña, Griffin & Hixon, Reference Bedore, Peña, Griffin and Hixon2016; see also Consonni, Cafiero, Marin, Tettamanti, ladanza, Fabbro & Perani, Reference Consonni, Cafiero, Marin, Tettamanti, ladanza, Fabbro and Perani2013; Wartenburger et al., Reference Wartenburger, Heekeren, Abutalebi, Cappa, Villringer and Perani2003). Similar to some of the previous work (e.g., Roncaglia-Denissen & Kotz, Reference Roncaglia-Denissen and Kotz2016), the present study suggests that high dual language competence, regardless of AoA, bilinguals can activate similar brain areas when processing morpho-syntax in both languages (see also Chee et al., Reference Chee, Tan and Thiel1999; Hasegawa et al., Reference Hasegawa, Carpenter and Just2002; Perani et al., Reference Perani, Paulesu, Sebastián-Gallés, Dupoux, Dehaene, Bettinardi, Cappa, Fazio and Mehler1998; Vingerhoets et al., Reference Vingerhoets, Van Borsel, Tesink, van den Noort, Deblaere, Seurinck, Vandemaele and Achten2003). A limitation of this work is the inclusion of only one bilingual group with early, systematic and high dual-language proficiency. The present findings nevertheless provide a set of principled findings that can now be used for future works with bilinguals that may have a wider range of dual-language proficiency abilities, language typologies, and contexts of acquisition. Although interpretations for the present data represent global bilingual versus monolingual distinctions on a small scale, they support the idea that for children who are exposed earlier to a new language, as well as growing up in environments where both languages are encouraged, may yield not only normative development (Bedore et al., Reference Bedore, Peña, Griffin and Hixon2016) but also high levels of language/linguistic competence, which may advance children's overall language faculty (Kuo & Anderson, Reference Kuo and Anderson2010).
Second, the findings may stem from language-specific experiences with Spanish and English. Morpho-syntactic inflectional variation in English is almost entirely limited to -ing, -ed and -s morphemes. In contrast, Spanish provides a rich variety of morpho-syntactic agreement contexts that include gender and number agreement for nouns, as well as a rich variation for tense and number agreement for verbs. Spanish–English bilinguals attend to morpho-syntactic structures of English sentences at a greater rate than monolingual English speakers (Hernandez et al., Reference Hernandez, Bates and Avila1994). Although both language tasks were completed in different sessions and fNIRS methodology is limited in its spatial resolution and cap positioning, children show an increased neural response to sentence processing in similar and predicted left IFG regions across their two languages. These results suggest that left frontal cortical regions support bilinguals’ ability to compute inflectional morphology for their two languages. Nevertheless, the current data identify the high specificity and selectivity of left IFG response in Spanish–English bilinguals, and provide a principled explanation for the heightened neural response to morpho-syntactic variations in English. This interpretation is consistent with the interrelated and bilingual language transfer hypotheses of bilingualism (Abutalebi & Green, Reference Abutalebi and Green2016; Cummins, Reference Cummins2001; Green & Abutalebi, Reference Green and Abutalebi2013; Kuo & Anderson, Reference Kuo and Anderson2010), but requires further adjudication with studies that consider other bilingual language configurations.
The findings are generally consistent with the Integrated Multilingual Model (MacSwan, Reference MacSwan2017) that integrates the idea that bilinguals can have language-specific development as well as shared cross-linguistic interaction that yields bilingual-specific signature processing. As is consistent with behavioral data for children acquiring ‘two first languages’ (e.g., Petitto & Kovelman, Reference Petitto and Kovelman2003), bilinguals and monolinguals showed similar levels of language proficiency and higher left IFG activation for later- versus earlier- acquired inflectional morphemes. Second, this increase in left IFG activation was observed in similar left IFG regions across the bilinguals’ two languages, suggesting a possible interaction in bilinguals’ neural representation for their two languages. Finally, the Adaptive Control model (Abutalebi & Green, Reference Abutalebi and Green2016; Green & Abutalebi, Reference Green and Abutalebi2013) suggests that daily demands of dual-language experiences impact linguistic and cognitive processes; bilinguals’ two languages are assumed to be co-active even when only one language is in use (Kroll et al., Reference Kroll, Dussias, Bice and Perrotti2015). Another possible explanation is that the increased attentional and mnemonic demand for processing two often co-active languages might alter the functionality of left IFG regions that specifically support the linguistic, mnemonic, and attentional demands of language processing. These same child participants also completed a visuo-spatial attention task during which the bilinguals showed greater left IFG activation than the monolinguals (Arredondo, Hu, Satterfield & Kovelman, Reference Arredondo, Hu, Satterfield and Kovelman2016). Taken together, the systematic use of two languages during the key years of brain plasticity for language organization may enhance the computational capabilities of the left IFG region and its engagement in dual-language development in young learners.
The present study's method is limited by the inclusion of ungrammatical sentences that the children are unlikely to hear from adult native speakers English in English or adult native speakers of Spanish in Spanish. However, we note that errors of morpheme omission are typical of child language until about age 6 (and sometimes beyond as is the case for individuals with language impairments). Yet children within the age range of our participants (6-11 years) often interact with slightly younger children at school, home, play dates (or L2 speakers such as bilingual children's parents) and may hear such morpheme omissions in their regular environment. Lastly, one of the potential caveats is the number of experimental items of 20 sentences per condition. This number of experimental items (and thus the duration of testing) was based on attention span for young children, as well as prior neuroimaging work suggesting that it is possible to obtain significant findings with children using around 16 experimental sentences per condition (e.g., Nuñez et al., Reference Nuñez, Dapretto, Katzir, Starr, Bramen, Kan, Bookheimer and Sowell2011).
The use of a grammatical judgment task in the present study allows us to not only build on the findings within the literature on typical language development, but also to use neuroimaging to understand the neurobiological mechanisms of language processing. Several prior and current studies show that children and adolescents still produce a protracted P600 ERP response on syntactic violations in contrast to adults. This outcome is identified as a possible indicator of syntactic re-analysis, or increased memory load associated with holding incomplete syntactic dependencies and derivations in memory (Osterhout & Holcomb, Reference Osterhout and Holcomb1992; Gouvea, Phillips, Kazanina & Poeppel, Reference Gouvea, Phillips, Kazanina and Poeppel2010). The present findings critically diverge from traditional theories of language processing that presume that bilinguals have reduced sensitivity to syntactic information and might compensate (i.e., achieve monolingual-like accuracy) by placing greater reliance on semantic and pragmatic cues in the interpretation of linguistic information (as evidenced through the inconsistency of ELAN response found across bilingual studies of morpho-syntactic processing, e.g., Frenck-Mestre, Carrasco-Ortiz, McLaughlin, Osterhout & Foucart, Reference Frenck-Mestre, Carrasco-Ortiz, McLaughlin, Osterhout and Foucart2010). In conclusion, these results uncover important data on young bilinguals’ strong reliance on their morpho-syntactic system.
5. Conclusions
The present study aimed to shed light on whether early bilingual exposure influences children's cortical organization for processing features of morpho-syntax. In our increasingly multilingual and multicultural society, it is important to consider the impact of bilingualism on the development of language, and how exposure to more than one language from a young age might shape an individual's brain both structurally and functionally. In the recent past, bilingualism was thought to impede cognitive and linguistic development, effectively ‘confusing’ the child's brain with multiple languages. We now know that this is not the case. The present findings add to the growing corpus of evidence that supports a ‘neural signature’ of bilingualism, quantitatively different from that of monolinguals, and carries implications for the future of educational policy in a multilingual world.
Supplementary material
To view supplementary material for this article, please visit https://doi.org/10.1017/S1366728918000512