Introduction
Our understanding of Southern Hemisphere Jurassic phytogeography and palaeoclimates is still limited compared to other periods of Earth history. This is mainly due to the overall meagre Jurassic plant fossil record from Gondwana (e.g. Cantrill Reference Cantrill2000, McLoughlin Reference McLoughlin2001, Rees & Cleal Reference Rees and Cleal2004, Cantrill & Hunter Reference Cantrill and Hunter2005). The major exceptions are the fossil floras from Hope Bay and Botany Bay, Antarctic Peninsula, which certainly rank among the richest and most diverse Mesozoic floras of the Southern Hemisphere (e.g. Gee Reference Gee1989, Rees & Cleal Reference Rees and Cleal2004). These long known plant fossil assemblages have become a standard reference for comparisons and biostratigraphic correlations throughout Gondwana. However, many Jurassic plant taxa of Gondwana are widespread and long ranging (McLoughlin Reference McLoughlin2001, Rees & Cleal Reference Rees and Cleal2004, Cantrill & Hunter Reference Cantrill and Hunter2005). As a result, the Hope Bay and Botany Bay floras have been variously dated as Early Jurassic (e.g. Rees & Cleal Reference Rees and Cleal2004), Middle Jurassic (e.g. Halle Reference Halle1913), or Late Jurassic to Early Cretaceous (e.g. Gee Reference Gee1989). The most recent review of the Hope Bay and Botany Bay floras (Rees & Cleal Reference Rees and Cleal2004) excluded an age younger that Middle Jurassic, and favoured an Early Jurassic age based mainly on the presence of typical Late Triassic to Early Jurassic taxa such as Goeppertella spp., Sagenopteris nilssoniana (Brongniart) Ward, and Archangelskya furcata (Halle) Herbst emend. Rees et Cleal (Rees & Cleal Reference Rees and Cleal2004). However, recent radiometric datings have firmly placed the Botany Bay Group floras into the Middle Jurassic (Hunter et al. Reference Hunter, Cantrill, Flowerdew and Millar2005).
Further Antarctic Jurassic macrofloras are mainly restricted to West Antarctica, including Cape Sobral and Jason Peninsula in Graham Land (e.g. Riley et al. Reference Riley, Crame, Thomson and Cantrill1997) as well as Alexander Island (Cantrill & Hunter Reference Cantrill and Hunter2005), the Merrick and Sweeney mountains in eastern Ellsworth Land (e.g. Cantrill & Hunter Reference Cantrill and Hunter2005), and possibly also the South Orkney Islands (Cantrill Reference Cantrill2000). Similar to other Jurassic floras of Gondwana, these plant fossil assemblages show a rather uniform composition of ubiquitous ferns (e.g. Equisetum L./Equisetites Sternberg, Todites Seward, Cladophlebis Brongniart, Matonidium Schenk, Coniopteris Brongniart), seed ferns (Pachypteris Brongniart, Sagenopteris Presl, Archangelskya Herbst), bennettitaleans (Otozamites Braun, Zamites Brongniart, Ptilophyllum Morris), and conifers (Brachyphyllum Brongniart, Pagiophyllum Heer, Elatocladus Halle) (e.g. Vakhrameev Reference Vakhrameev1991, McLoughlin Reference McLoughlin2001, Rees & Cleal Reference Rees and Cleal2004, Cantrill & Hunter Reference Cantrill and Hunter2005). Jurassic macrofloras from West Antarctica consist of altered compression/impression assemblages generally lacking cuticles, which limits their palaeobiological and palaeoecological significance.
Only a few small collections of Jurassic plant fossils have been reported from East Antarctica, which was situated in the continental interior of southern Gondwana during the Early Jurassic. Otozamites antarcticus Plumstead, a poorly defined species based on only a single specimen, and the putative podocarpacean conifer Nothodacrium warrenii Townrow have been described from Carapace Nunatak, south Victoria Land (Plumstead Reference Plumstead1962, Townrow Reference Townrow1967a). Permineralized deposits at Storm Peak in the Queen Alexandra Range and at Mount Carson in north Victoria Land have yielded the anatomically preserved dipterid fern Polyphacelus stormensis Yao, Taylor et Taylor emend. Bomfleur et Kerp (Yao et al. Reference Yao, Taylor and Taylor1991, Bomfleur & Kerp Reference Bomfleur and Kerp2010). Furthermore, rafts of tuffitic siltstones in the Mawson breccias of south Victoria Land have been reported to contain liverworts, ferns, and conifers (Cantrill Reference Cantrill2000, Cantrill & Hunter Reference Cantrill and Hunter2005), but these have not been formally described to date.
During the 9th German Antarctic North Victorialand Expedition (GANOVEX IX 2005/2006) plant fossils were collected from three outcrops in the newly described Early Jurassic Shafer Peak Formation in north Victoria Land, Transantarctic Mountains (Fig. 1; Schöner et al. Reference Schöner, Viereck-Götte, Schneider and Bomfleur2007, Bomfleur & Kerp Reference Bomfleur and Kerp2010). The material constitutes the so far richest, most diverse, and best-preserved collection of Jurassic plant compressions and impressions from East Antarctica. Here, we present a detailed account on the systematic composition of these floras and discuss their palaeoclimatological and palaeoenvironmental implications.
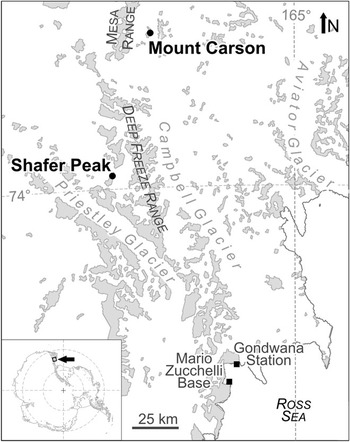
Fig. 1 Locality map showing the macrofossil sites in southern north Victoria Land, East Antarctica. Light grey colours indicate areas of rock outcrop.
Source strata, material and methods
The Shafer Peak Formation is a c. 50 m thick succession of silicic tuffitic silt- and fine-grained sandstones that occurs between the siliciclastic Section Peak Formation and the overlying late Early Jurassic Kirkpatrick lavas in north Victoria Land, East Antarctica (Schöner et al. Reference Schöner, Viereck-Götte, Schneider and Bomfleur2007). Typical sedimentary features include climbing-ripple lamination, horizontal lamination, and accumulations of clay-gall rip-up clasts. The Shafer Peak Formation is interpreted as a succession of fluvially reworked, silicic volcanic ashes, and presumably is a regional equivalent of the Hanson Formation in the central Transantarctic Mountains (Fig. 2). Similar silicic tuffitic deposits have also been reported from south Victoria Land (Elliot & Fleming Reference Elliot and Fleming2008). Locally, mafic volcaniclastic deposits are intercalated at different levels within the Shafer Peak Formation; these derive from local hydrovolcanic explosion/eruption centres (Viereck-Götte et al. Reference Viereck-Götte, Schöner, Bomfleur and Schneider2007).

Fig. 2 Simplified correlation chart showing the main lithologies and stratigraphic relationships between Triassic–Jurassic rock units in different regions of the Transantarctic Mountains (modified from Schöner et al. Reference Schöner, Viereck-Götte, Schneider and Bomfleur2007).
Plant fossils were collected from three outcrops in the Shafer Peak Formation at Shafer Peak and at Mount Carson east of the Mesa Range (Fig. 3). At all sites, macrofossils occur as impressions and generally coalified compressions in light grey to beige coloured, tuffitic silt- and fine-grained sandstones. Due to the silty to sandy matrix preservation of morphological details is usually poor.

Fig. 3 Schematic lithological columns showing main lithologies and the positions of plant-bearing horizons at the new plant fossil localities in north Victoria Land, East Antarctica.
At Shafer Peak, plant fossils were collected from a 1.4 m thick, bluff forming series of tuffitic siltstones about 10 m below the base of the Kirkpatrick lavas (Fig. 3). The assemblage consists of up to 40 cm long leaves and leaf fragments associated with abundant plant debris and pieces of charcoal. Although plant axes are generally buried parallel to bedding, the attached foliage (i.e. of bennettitalean leaves) may be oriented obliquely or vertically to bedding. Five bennettitalean leaves from Shafer Peak yielded fragments of cuticle. In addition, organic residues of bulk macerations of this bed contained a few dispersed plant cuticles, including fragments of conifer shoots and isolated needles.
Three further small collections of plant remains are from sections of the Shafer Peak Formation exposed along the ridges extending east and north of Mount Carson. At the eastern ridge, plant remains occur as strongly fragmented foliage and axes in a poorly bedded tuffitic layer (CE15) near the base of the sedimentary succession (Fig. 3). The basal 25 cm of this plant bearing bed are very rich in lenticular, medium grey clay galls up to 3 cm in diameter. On the northern ridge of Mount Carson, plant macrofossils were collected from two horizons (Fig. 3). The lower plant bearing bed in the middle part of the section is a tuffitic siltstone bed (CN08) that yielded a few isolated sphenophyte fragments. Some of the fragmentary axes are preserved three-dimensionally, and are filled with dark grey claystone. The second plant bearing bed higher up in the section (CN14) is a massive, light grey tuffitic siltstone containing isolated, up to 5 cm long plant fragments as well as conchostracans, beetle elytra, small pieces of charcoal, and juvenile mafic volcanic clasts. In this bed, fossils are frequently buried in random orientation, even perpendicular to the bedding.
Plant fossils were analysed with a Leica MZ16 stereomicroscope. Macrophotographs were taken with a Nikon D100 digital SLR camera; in some instances cross polarization (polarized light source and a corresponding camera filter) was used in order to enhance contrast. Microphotographs were taken with a Nikon DS-LM digital camera system.
Bulk samples and selected hand specimens were processed via slightly modified standard techniques (e.g. Kerp Reference Kerp1990). Because the tuffitic matrix is strongly cemented and very resistant, treatment with 48% hydrofluoric acid (HF) for up to several months was required to isolate the plant remains from the mineral matrix. Residues were then carefully neutralized by repeatedly adding distilled water and decanting. Although this method is more time consuming than the conventional use of sieves, mechanical destruction can be greatly reduced. It would probably have been impossible to obtain the very brittle bennettitalean cuticles from Shafer Peak using other methods. Cuticles were macerated using Schulze’s reagent (40% HNO3 + a few crystals of KClO3) for about three hours, and then treated with low concentration potassium hydroxide (2% KOH) for a few seconds. The cuticles were then carefully rinsed in water, dehydrated in pure glycerol, and finally mounted in permanent glycerine jelly slides.
The material is housed in the collection of the Forschungsstelle für Paläobotanik am Institut für Geologie und Paläontologie der Westfälische Wilhelms-Universität Münster, under accession numbers GIX-CE15-001–040, GIX-CN08-001–002, GIX-CN14-001–048, GIX-SHC032-001–029 (hand specimens) and GIX-SHC-000(001–032) (bulk material).
Results
Systematic descriptions
Phylum LYCOPHYTA
Order ISOETALES
Family ISOETACEAE
Genus Isoetites Muenster
Type species. Isoetites crociformis Muenster, 1842
Isoetites sp.
Fig. 4a–c

Fig. 4 Impression/compression material of Isoetites sp. (a–c) and Equisetites sp. (d–i) from the Lower Jurassic of north Victoria Land, East Antarctica. a. Isolated leaf fragments of Isoetites sp.; note co-abundant greyish clay galls. GIX-CE15-018a, eastern ridge of Mount Carson. Scale bar = 1 cm. b. Detail of Fig. 4a showing a microphyll with single central vein. Scale bar = 2 mm. c. Leaf base of a microphyll of Isoetites sp. GIX-CE15-023, eastern ridge of Mount Carson. Scale bar = 2 mm. d. Two axes of Equisetites sp. GIX-CE15-003, eastern ridge of Mount Carson. Scale bar = 5 mm. e. Rhizome fragment of Equisetites sp. with five aerial shoots. GIX-CE15-020a, eastern ridge of Mount Carson. Scale bar = 5 mm. f. Isolated leaf sheath of Equisetites sp. GIX-CN14-001b, northern ridge of Mount Carson. Scale bar = 5 mm. g. Clay-filled cast of an equisetalean stem fragment. GIX-CN08-002a, northern ridge of Mount Carson. Scale bar = 5 mm. h. Rhizome fragment of Equisetites sp. with attached basal portion of an aerial stem; note whorled arrangement of roots. GIX-CE15-017, eastern ridge of Mount Carson. Scale bar = 5 mm. i. Isolated nodal diaphragm of Equisetites sp. GIX-CN14-004, northern ridge of Mount Carson. Scale bar = 5 mm.
Material: GIX-CE15-010, 018, 023.
Description: Sterile microphyllous leaves, needle-like elongate, straight to slightly tapering in apical direction, entire-margined, up to 60 mm long by 1.5 mm wide (Fig. 4a & b); leaf base slightly swollen, with subtriangular to transverse rhombic shape (Fig. 4c).
Remarks: The leaf shape, dimension, and the presence of a single central vein in the detached leaves indicate affinities with the Isoetales. The specimens resemble isoetalean microphylls from the Mesozoic of Australia, such as, Isoetites abundans Tosolini et McLoughlin (McLoughlin et al. Reference McLoughlin, Tosolini, Nagalingum and Drinnan2002) and Isoetites sp. (Jansson et al. Reference Jansson, McLoughlin, Vajda and Pole2008). However, the absence of further diagnostic features such as rhizomorphs and fertile details hamper a definite assignment and more detailed comparisons.
Phylum SPHENOPHYTA
Order EQUISETALES
Family EQUISETACEAE
Genus Equisetites Sternberg
Type species. Equisetites muensteri Sternberg, 1833
Equisetites sp.
Fig. 4d–i
Material: GIX-CE15-003, 017, 020, 026; GIX-CN08-002a+b, 003; GIX-CN14-001a+b, 002, 004, 005, 014, 034.
Description: Fragments of rhizomes, unbranched aerial shoots, isolated leaf sheaths, and nodal diaphragms. Rhizome fragments up to 2.5 cm long and 4 mm wide, smooth, transversely divided into nodes and internodes, with aerial shoots and adventitious roots arising from the nodes (Fig. 4e & h). Aerial stem fragments up to 3 cm long and 3 mm wide, unbranched, consisting of up to five nodes and internodes (Fig. 4d). Length of internodes decreasing from 5 mm at the base to 3 mm at the apex. Surface of internode in basal portions smooth. Leaf sheaths increasingly overlapping the superpositioned nodes (Fig. 4d), with fine longitudinal striations, terminating in a ring of free leaf tips accounting for c. one-sixth to one-fifth of the leaf length. Free leaf tips with parallel margins and an obtuse rounded apex. Compressions of isolated leaf sheaths with more or less square outline, with 8 to 13 free leaf tips and corresponding longitudinal furrows preserved (Fig. 4f & g). Compressions of isolated nodal diaphragms flat, circular, up to 8 mm in diameter, with even and smooth surface; outer margin of nodal diaphragm with a sloping rim of c. 0.5 mm width, evenly divided by radial grooves, presumably corresponding to the number of free leaf tips (Fig. 4i).
Remarks: We retain the present specimens in the morphogenus Equisetites following Watson & Batten (Reference Watson and Batten1990) because they cannot be assigned to the living genus Equisetum. Sphenophytes are common elements of Jurassic floras of southern Gondwana. The most widespread species is Equisetum laterale Phillips emend. Gould. Within the Antarctic, Equisetum laterale has been described from the Antarctic Peninsula (e.g. Gee Reference Gee1989, Rees & Cleal Reference Rees and Cleal2004) and the Latady Basin (Cantrill & Hunter Reference Cantrill and Hunter2005). The present material is easily distinguishable from E. laterale by the nodal diaphragms; E. laterale has nodal diaphragms composed of a central hub with radiating spokes resulting in a wagon-wheel-like appearance (Halle Reference Halle1913), whereas the surface of the diaphragms is smooth and even in our specimens (Fig. 4i). The fragmentary nature and rather poor preservation of the material hamper further comparisons and confident species assignment.
Phylum PTERIDOPHYTA
Order GLEICHENIALES
Family DIPTERIDACEAE
Genus Clathropteris Brongniart
Type species. Clathropteris meniscoides Brongniart, 1828
Clathropteris meniscoides

Fig. 5 Fern fossils from the Lower Jurassic of north Victoria Land, East Antarctica. a. Clathropteris meniscoides. GIX-SHC32-013, Shafer Peak. Scale bar = 1 cm. b. Matonidium sp. cf. M. goeppertii. GIX-CN14-046b, northern ridge of Mount Carson. Scale bar = 1 cm. c. Matonidium sp. cf. M. goeppertii. GIX-CN14-041a, northern ridge of Mount Carson. Scale bar = 5 mm. d. Matonidium sp. cf. M. goeppertii, isolated pinnule. GIX-CN-019, northern ridge of Mount Carson. Scale bar = 1 mm. e. Matonidium sp. cf. M. goeppertii. GIX-CN14-045a, northern ridge of Mount Carson. Scale bar = 5 mm. f. Coniopteris hymenophylloides, pinna fragment. GIX-CE15-018b, eastern ridge of Mount Carson. Scale bar = 5 mm. g. Coniopteris hymenophylloides, isolated sterile pinnule. GIX-CE15-030, eastern ridge of Mount Carson. Scale bar = 5 mm. h. Coniopteris hymenophylloides, isolated sterile pinnule. GIX-CN14-039, northern ridge of Mount Carson. Scale bar = 5 mm. i. Coniopteris hymenophylloides, isolated sterile pinnule. GIX-CN14-026a, northern ridge of Mount Carson. Scale bar = 5 mm. j. Coniopteris hymenophylloides, isolated fertile pinnule. GIX-CE15-010, eastern ridge of Mount Carson. Scale bar = 2 mm. k. Coniopteris murrayana, fertile pinna fragment (some sori marked with arrows). GIX-CE15-033, eastern ridge of Mount Carson. Scale bar = 5 mm. i. Spiropteris sp. GIX-CN14-030, eastern ridge of Mount Carson. Scale bar = 1 mm.
Selected synonymy:
Reference Harris1931 Clathropteris meniscoides Brongniart; Harris p. 88, pl. 15, figs 1 & 9; pl. 16 figs 9 & 10; pl. 18 figs 3, 5 & 12; text-figs 32–34.
Reference Oishi1940 C. elegans Oishi, p. 213.
2009 C. meniscoides Brongniart; Schweitzer, Schweitzer, Kirchner, Van Konijnenburg-Van Cittert, Van der Burgh & Ashraf pl. 21 & 2; pl. 22, figs 1–3; pl. 23, fig. 1; text-figs 16 & 17.
Material: GIX-SHC32-012–014.
Description: Narrow-triangular leaf segments, up to 11 cm long with a basal width of up to 3.5 cm. Blunt, asymmetrical teeth arising from the margin at angles of less than 45°. Rachis straight, up to 2 mm wide, slightly tapering, persisting into leaf apex. Secondary veins subopposite or alternate, arising at intervals of about 7 mm and at wide angles between 70 and 90°, slightly curving towards the apex. Tertiary and higher order venation forming a dense and very regular orthogonal meshwork.
Remarks: These specimens have recently been described and compared in detail (Bomfleur & Kerp Reference Bomfleur and Kerp2010). The Antarctic Clathropteris meniscoides presumably represents the impression/compression state of the permineralized dipterid Polyphacelus stormensis from Storm Peak and Mount Carson (Yao et al. Reference Yao, Taylor and Taylor1991, Bomfleur & Kerp Reference Bomfleur and Kerp2010).
Family MATONIACEAE
Genus Matonidium Schenk
Type species. Matonidium goeppertii (Ettingshausen) Schenk emend. Harris, Reference Harris1961
Matonidium sp. cf. M. goeppertii
Fig. 5b–e
Material: GIX-CN14-007, 008, 016, 017, 019–21, 028, 029, 037, 041, 045, 046.
Description: Preserved pinna portions oblong to oblanceolate in outline with a basal width of up to 15 mm, tapering towards the apex. Pinnae with a strong, straight midrib projecting prominently from the lower leaf surface. Pinnules opposite to subopposite, rarely alternate, inserted at angles between 60 and 90° to the pinna midrib, either straight or bent towards the apex. Apical pinnules generally inserted at lower angles than pinnules in proximal pinna portions. Pinnules close, sometimes touching, oblong, oblanceolate or sometimes falcate, up to 7 mm long and up to 2 mm wide, generally about three to four times as long as wide; pinnule apices obtuse. Sterile pinnule surface flattened (Fig. 5b), margins of fertile pinnules abaxially recurved (Fig. 5d & e). Pinnule margins entire, connected at their bases by a c. 0.5 mm wide laminar wing. Primary vein prominent, running straight up to near the apex. Secondary veins arising at angles between 60 and 90°, running straight to the margin, simple or once forking. Fertile pinnules with a single row of sori at each side of the mid-vein, sori of circular outline with a diameter of up to 0.5 mm, in some cases with a dark central spot (Fig. 5i).
Remarks: The distribution and organization of sori in fertile pinnules suggests affinities with the Matoniaceae. Common Mesozoic fossil representatives of the family include Phlebopteris Brongniart, Matonidium, and Matonia Brown, which are very similar in macromorphology and are distinguished from each other mainly by the development of an indusium covering the sori (Van Konijnenburg-Van Cittert Reference Van Konijnenburg-van Cittert1993). These features are not preserved in the present material, although the dark central spot in some of the sori (Fig. 5i) may represent remains of a receptacle. Harris (Reference Harris1961) provided a key to distinguish between different matoniaceaen taxa from the Yorkshire Jurassic on the basis of their venation pattern. Following this key, the present fossils can be assigned to the genus Matonidium as the vein branches are all free (unlike in most Phlebopteris species) and the secondary veins arise at angles of more than 45° from the midrib (unlike in Selenocarpus Schenk). Van Konijnenburg-Van Cittert (Reference Van Konijnenburg-van Cittert1993) mentioned that pinnules of Matonidium are generally smaller, more slender and more falcate compared to those of Phlebopteris and Matonia, which lends further support to ascribe the present material to Matonidium. The fossils are superficially similar to Matonidium goeppertii figured, e.g. by Harris (Reference Harris1961, p. 114, fig. 37 C, G & H) and Appert (Reference Appert1973, e.g. pl. 30, fig. 5; pl. 33, fig. 3; pl. 36, fig. 4).
Order CYATHEALES
Family DICKSONIACEAE
Morphogenus Coniopteris Brongniart emend. Harris
Type species. Coniopteris murrayana (Brongniart) Brongniart emend. Harris, Reference Harris1961
Coniopteris murrayana
Selected synonymy:
1913 Coniopteris hymenophylloides (Brongniart) Seward; Halle pl. 3, figs 27, 28 & 28a.
1961 C. murrayana (Brongniart) Brongniart emend. Harris, text-figs 55–57.
1989 C. murrayana (Brongniart) Brongniart emend. Harris; Gee pl. 1, fig. 9.
Material: GIX-CE15-033.
Description: Fertile pinna fragment, 1.5 cm long and 1 cm wide, bearing opposite to subopposite pinnules inserted at wide angles. Pinnules widely spaced, free, linear-lanceolate in outline, deeply lobed. A single sorus borne distally on each lobe except for the two basal lobes. Sori up to 1 mm in diameter and preserved as relatively thick carbonaceous compressions with a semicircular shape.
Remarks: We distinguish this single fertile specimen from the co-occurring Coniopteris specimens because it clearly differs in its widely spaced pinnules and the well-developed pinnule lamina. Both features are typical for C. murrayana (e.g. Harris Reference Harris1961, Gee Reference Gee1989).
Coniopteris hymenophylloides (Brongniart) Seward emend. Harris
Fig. 5f–j
Selected synonymy:
1961 Coniopteris hymenophylloides (Brongniart) Seward emend. Harris, text-figs 53 & 54.
1989 C. hymenophylloides (Brongniart) Seward emend. Harris; Gee pl. 1, figs 7 & 8.
2009 C. hymenophylloides (Brongniart) Seward emend. Harris; Schweitzer, Kirchner, Van Konijnenburg-Van Cittert, Van der Burgh & Ashraf pl. 28, fig. 3; pl. 29, figs 1–3; pl. 30, figs 1 & 2; pl. 31, figs 1–4; pl. 32, figs 1–3; text-figs 28–31a, b.
Material: GIX-CE15-001, 002, 004, 005, 007, 008, 010, 012–018, 020, 024, 025, 027–031, 034–040; GIX-CN14-010, 018, 026, 039.
Description: Pinnae straight, narrow-elongate to triangular in outline, with an up to 1 mm wide prominent central vein. Pinnules opposite or subopposite in basal pinna portions and increasingly alternate towards the apex. Insertion angles decreasing from up to 90° in basal pinna portions to less than 30° near the apex. Largest pinnules in basal pinna portions up to 7 mm long, linear-lanceolate in outline and deeply divided into up to seven lobes (Fig. 5f). Size and degree of lobing decreasing towards the apex; terminal pinnules lanceolate, entire-margined (Fig. 5i). Venation pattern obscure. Fertile pinnule laminae strongly reduced to short stalk-like lobes each bearing a single cup shaped sorus (Fig. 5j). Compressed sori up to 1 mm wide, semicircular to reniform in outline.
Remarks: The narrow triangular pinna outlines and the strong reduction of fertile pinnule laminae to stalk-like lobes allows an assignment to Coniopteris hymenophylloides (e.g. Harris Reference Harris1961, Gee Reference Gee1989). Although sterile pinnae are strongly fragmented and show little diagnostic criteria they fully agree with those figured by previous authors (e.g. Harris Reference Harris1961, fig. 53b–d & f; Schweitzer et al. Reference Schweitzer, Schweitzer, Kirchner, van Konijnenburg-van Cittert and Ashraf2009, text-figs 28a–d & 29a–c, pl. 29, fig. 3).
Ordo incertae sedis
Familia incertae sedis
Morphogenus Spiropteris Schimper
Type species. Spiropteris miltonii (Brongniart) Schimper, 1869
Spiropteris sp.
Material: GIX-CN14-030.
Description: Fragment of an up to 2 mm long coiled pteridophyll crozier. Axis involute, less than 1 mm wide, smooth, without foliar appendages.
Remarks: The morphogenus Spiropteris serves as a repository for immature fronds with circinate vernation (e.g. Taylor et al. Reference Taylor, Taylor and Krings2009).
CYCADOPHYTA
Order BENNETTITALES
Familia incertae sedis
Genus Otozamites Braun emend. Watson et Sincock
Type species. Otozamites (Zamites) brevifolius Braun in Muenster, 1843
Remarks: The differentiation of superficially similar morphogenera for pinnately compound leaves of supposed bennettitalean affinity has been intensively discussed (e.g. Harris Reference Harris1969, Barnard & Miller Reference Barnard and Miller1976, Gee Reference Gee1989, Watson & Sincock Reference Watson and Sincock1991, Rees & Cleal Reference Rees and Cleal2004, McLoughlin & Pott Reference McLoughlin and Pott2009). There is general agreement that Otozamites can be reasonably well delimited from Zamites and Ptilophyllum based on the presence of an auricle at the acroscopic margin of the leaflet bases, and by asymmetrically radiating veins. Further, Zamites has symmetrical leaflet bases, whereas the basiscopic margins of the leaflet bases in Ptilophyllum are decurrent (e.g. Harris Reference Harris1969, Watson & Sincock Reference Watson and Sincock1991).
Otozamites linearis Halle
Figs 6a–i, 7a–j, 8a–e & 9

Fig. 6 Otozamites linearis from the Lower Jurassic of north Victoria Land, East Antarctica. a. Central to apical portion of a large leaf. GIX-SHC32-024, Shafer Peak. Scale bar = 2 cm. b. Central to apical portion of a medium-sized leaf. GIX-SHC32-001a, Shafer Peak. Scale bar = 1 cm. c. Central portion of a medium-sized leaf. GIX-SHC32-023, Shafer Peak. Scale bar = 1 cm. d. Central portion of a large leaf. GIX-SHC32-022b, Shafer Peak. Scale bar = 1 cm. e. Isolated leaflet. GIX-CE15-019, eastern ridge of Mount Carson. Scale bar = 5 mm. f. Basal leaf portion. GIX-SHC32-007, Shafer Peak. Scale bar = 1 cm. g. Basal leaf portion. GIX-SHC32-018, Shafer Peak. Scale bar = 1 cm. h. Basal to central leaf portion. GIX-SHC32-025, Shafer Peak. Scale bar = 1 cm. i. Central portion of a small leaf. GIX-SHC32-020b, Shafer Peak. Scale bar = 5 mm.
Selected synonymy:
1913 Otozamites linearis Halle, pl. 7, figs 1–4, 8, 9, 9a & 11, text-fig. 15.
1989 O. linearis Halle; Gee pl. 6, figs 50–52.
1990 O. linearis Halle; Baldoni pl. 4, figs 1–3.
2004 O.? linearis Halle; Rees & Cleal pl. 14, figs 1–3.
2009 O. linearis Halle; McLoughlin & Pott figs 9a & 10a–f.
Material: GIX-CN14-038, 048; GIX-SHC32-001, 003, 004, 006–011, 015, 017, 018, 020–025, 028.
Description: Leaves pinnately compound, lanceolate in outline, straight (Fig. 6a, c, d, & f) to curved (Fig. 6b). Leaflets inserted on the upper side of the rachis at oblique angles to the leaf plane and at angles of 40 to 70° to the rachis (Fig. 6e), touching to strongly imbricate (Fig. 6c, d & h), with a more or less pronounced acroscopic basal auricle (Fig. 6e) generally overlapping the rachis and basal portions of superpositioned leaflets. Leaflets ovate, measuring c. 6 x 4 mm in basal leaf portions (Fig. 6g) to oblong, straight to falcate, and up to 25 x 7 mm large in central portions (Fig. 6a & d); apices obtuse. Leaf apex formed by a rounded–subrhombic terminal segment.
Veins radiating asymmetrically; first basiscopic veins ending farther from the rachis than first acroscopic veins (Fig. 6e); venation dense, with about two to four veins crossing a given line of 1 mm length oriented perpendicular to the margin in central leaflet portions.
Leaves hypostomatic. Upper cuticle thin, granulate, with a homogeneous pattern of randomly oriented, polygonal epidermal cells with sinuous anticlinal walls; epidermal cells about 40–80 μm long and 25–50 μm wide (Fig. 7a). Lower cuticle thin, well differentiated into costal fields and intercostal fields (Figs 7b & c and 8a). Costal fields with three to eight more or less regular longitudinal rows of rectangular epidermal cells with moderately to strongly sinuous longitudinal walls and generally less sinuous transverse walls (Fig. 7b). Intercostal fields extending between vein courses, ending abruptly c. 100 μm before the leaf margin (Fig. 7c). Stomata brachyparacytic (syndetocheilic), restricted to intercostal fields; most stomatal apertures oriented transversely to vein courses (Figs 7d & 8a). Stomatal complexes composed of a sunken pair of reniform guard cells with thickened distal dorsal ledges (Figs 7j & 8c–e) and an overlying pair of strongly cutinized lateral subsidiary cells, each bearing a more or less pronounced solid papilla (Fig. 7e–g & i). Stomatal complexes almost superficial (Fig. 7h & i) to deeply sunken below the level of the epidermis in an epistomatal chamber (Fig. 7e–g); in few cases two stomata share a single epistomatal chamber (Fig. 7f & g). Adjacent (superficial) epidermal cells usually with a single, hollow, bilobate to dendroid papilla (Fig. 7d, f, h & i). Other cells in intercostal fields smooth or bearing a single, hollow, simple to dendroid papilla (Fig. 7d). Leaf margins with parallel rows of ordinary cells similar to those in costal fields.

Fig. 7 Cuticles of Otozamites linearis from the Lower Jurassic of Shafer Peak, north Victoria Land, East Antarctica. a. Upper leaf surface. GIX-SHC32-021-$10. Scale bar = 50 μm. b. Lower leaf surface with costal field (centre) and two adjacent intercostal fields. GIX-SHC32-028-$01. Scale bar = 50 μm. c. Lower leaf surface showing differentiation into costal and intercostal fields and leaflet margin. GIX-SHC32-021-$14. Scale bar = 100 μm. d. Intercostal field of lower leaf surface with transversely oriented, little sunken stomatal complexes; note hollow dendroid papillae. GIX-SHC32-000(029)-$1. Scale bar = 25 μm. e. Intercostal field of lower leaf surface with stomata sunken in epistomatal chambers; note solid papillae on subsidiary cells. GIX-SHC32-001-$06. Scale bar = 25 μm. f. Distal view of an epistomatal chamber with two stomata overarched by large, hollow, and complexly dendroid papillae. GIX-SHC32-001-$07. Scale bar = 25 μm. g. Same as Fig. 7f in proximal focus, showing two stomata; note solid papillae on subsidiary cells. Scale bar = 25 μm. h. Moderately sunken stomatal complex surrounded by partly overarching, lobed to dendroid hollow papillae. GIX-SHC32-000(029)-$1. Scale bar = 10 μm. i. Almost superficial stomatal complex with hollow dendroid papillae on neighbouring cells, solid papillae on subsidiary cells and guard cell cutinizations. GIX-SHC32-000(029)-$1. Scale bar = 10 μm. j. Guard cell cutinizations. GIX-SHC32-001-$05. Scale bar = 10 μm.

Fig. 8 SEM views of the interior surface of the lower leaf cuticle of Otozamites linearis from the Lower Jurassic of Shafer Peak, north Victoria Land, East Antarctica. GIX-SHC32-000(029)-$1b. a. Cuticle with costal field (centre) and two adjacent intercostal fields. Scale bar = 50 μm. b. Detail showing a group of stomata in an intercostal field, note the hollow bases of protruding papillae between stomatal complexes. Scale bar = 50 μm. c–e. Details showing individual stomatal complexes, note that unlike the hollow papillae on adjacent epidermal cells, subsidiary cell papillae are not recognizable under SEM because of the solid bases. Scale bars = 20 μm.
Remarks: The specimens are assigned to Otozamites linearis based on: 1) the comparatively wide-lanceolate shape, 2) the oblong leaflet shape (length/width ratio between three and four) in central and apical leaf portions, 3) radiating venation, and 4) the common presence of solid, overarching papillae on subsidiary cells (Gee Reference Gee1989, Baldoni Reference Baldoni1990, Rees & Cleal Reference Rees and Cleal2004, McLoughlin & Pott Reference McLoughlin and Pott2009). Otozamites antarcticus from the Lower Jurassic of Carapace Nunatak (Plumstead Reference Plumstead1962) may be conspecific with O. linearis. The former is known from a single, small leaf fragment that falls within the morphological variability seen in the present material.
The present material includes several large leaf fragments that for the first time provide information on the variability of Otozamites linearis. The morphological variability of leaflets relates to the position within the leaf, but probably also reflects leaf maturity (Fig. 9). Cuticles with consistent epidermal and cuticular features were obtained from five different leaves, supporting their assignment to a single, morphologically variable species. Cuticles of O. linearis were previously described from the Middle Jurassic of Patagonia (e.g. Baldoni Reference Baldoni1990). The material agrees with the present specimens in bearing solid, overarching papillae on the subsidiary cells. The poor preservation and illustration of the material hampers more detailed comparisons of the cuticular features.

Fig. 9 Suggested reconstruction of a large, mature leaf of Otozamites linearis showing variability of leaflet dimension, shape, and attachment. Scale bar = 5 cm.
Based only on gross morphology, individual specimens or fragments of Otozamites linearis may be indistinguishable from other, coeval Otozamites species, i.e. O. ptilophylloides Barnard et Miller from the upper Lower to lower Middle Jurassic of Iran (Barnard & Miller Reference Barnard and Miller1976, Schweitzer & Kirchner Reference Schweitzer and Kirchner2003) and O. hsiangchiensis Sze from the Lower Jurassic of China (e.g. Sun et al. Reference Sun, Yang and Shen1989, Wang et al. Reference Wang, Ni, Jiang and Tian2008). Both O. ptilophylloides and O. hsiangchiensis have considerable variability in leaf and leaflet shapes (Schweitzer & Kirchner Reference Schweitzer and Kirchner2003, Wang et al. Reference Wang, Ni, Jiang and Tian2008). These, together with O. linearis, overlap in gross morphology and cuticular features (see Barnard & Miller Reference Barnard and Miller1976, Sun et al. Reference Sun, Yang and Shen1989), and differ only in minor details. The main distinguishing feature of O. hsiangchiensis is that stomatal pits are never overarched by papillae or encircling cell protrusions (Sun et al. Reference Sun, Yang and Shen1989). Cuticles of O. ptilophylloides are very similar to those of O. linearis, and characterized by densely spaced, hollow, bilobed to dendroid papillae in the intercostal fields and the presence of epistomatal chambers. The main differences between O. ptilophylloides and O. linearis are that stomata of O. ptilophylloides are always laterally displaced in the epistomatal chambers, and that subsidiary cells usually lack solid papillae (Barnard & Miller Reference Barnard and Miller1976, Schweitzer & Kirchner Reference Schweitzer and Kirchner2003). However, the latter feature may be artificial, because solid subsidiary cell papillae are recognizable in light microscope preparations only (Fig. 7e, g & i) but not in interior view under SEM (Fig. 8b–e). The present material demonstrates that the development of papillae and epistomatal chambers in O. linearis may vary even within individual leaflets. If future studies should prove that the same variability also occurs in O. ptilophylloides, the latter should be considered as a synonym of O. linearis. Compared to the Otozamites species from the Yorkshire Jurassic, leaves of O. linearis show the greatest morphological similarities to O. graphicus Leckenby, O. simpsonii Harris, and O. penna Harris (Harris Reference Harris1969). Otozamites graphicus can easily be distinguished by its much longer leaflets (exceeding 3 cm) and the consistent lateral displacement of stomatal complexes within each epistomatal chamber (Harris Reference Harris1969). Otozamites simpsonii and O. penna both differ from O. linearis in having abaxial trichomes, and lacking papillae on the subsidiary cells.
Otozamites linearis is a comparatively rare species in Jurassic floras of Gondwana. It has been reported from the Middle Jurassic Hope Bay and Botany Bay floras (Halle Reference Halle1913, Gee Reference Gee1989, Rees & Cleal Reference Rees and Cleal2004), the Middle Jurassic of Neuquén Province, Argentina (e.g. Baldoni Reference Baldoni1990), and from the Upper Jurassic of Western Australia (McLoughlin & Pott Reference McLoughlin and Pott2009).
Otozamites sanctae-crucis Feruglio, Reference Feruglio1951
Fig. 10a & b
Selected synonymy:
1951 Otozamites sanctae-crucis Feruglio, pl. II, figs 4 & 5; pl. III, figs 1 & 3 (right)
1987 O. sp. Tidwell, Kim & Kimura, fig. 2d; pl. II, fig. 3
2005 O. santaecrucis Feruglio; Cantrill & Hunter fig. 3c, d & i
Material: GIX-CN14-047; GIX-SHC32-016, 027.
Description: Leaves pinnately compound, straight, narrow-lanceolate to almost linear, up to 25 cm long and up to c. 2 cm wide (Fig. 10a). Leaflets densely spaced, alternate, and inserted on the upper side of the rachis at an oblique angle to the leaf plane, forming dense imbricate foliation (Fig. 10b). Leaflets inserted with the basiscopic portion of the leaflet base at angles between 40 and 70°, oblong to subrhombic, some slightly falcate, with a length/width ratio between one and two; acroscopic margins straight to slightly concave, with a distinct basal auricle overlapping the rachis and the basal portion of the superpositioned leaflet; basiscopic margins straight for most of their length, then gradually tapering to form a rounded to obtuse apex.

Fig. 10 Further cycadophyte and putative conifer macrofossils from the Lower Jurassic of north Victoria Land, East Antarctica. a. Otozamites sanctae-crucis, central portion of a large leaf. GIX-SHC32-027, Shafer Peak. b. Otozamites sanctae-crucis, small leaf fragment. GIX-SHC32-016, Shafer Peak. c. Zamites sp. (centre) and O. linearis (lower left), field image of leaf fragments on a large block of tuffitic siltstone at Shafer Peak. d. Cycadolepis sp. GIX-CE15-021, eastern ridge of Mount Carson. e. Schizolepis sp. GIX-CN14-022a, northern ridge of Mount Carson.
Remarks: The specimens clearly differ from the co-occurring Otozamites linearis in having much shorter leaflets resulting in very narrow leaves, with a greatest width of c. 2 cm and a length of 25 cm. Otozamites sanctae-crucis is a variable species that encompasses a wide range of leaf and leaflet shapes. One of the morphological varieties is characterized by small, imbricate, ovate to subrhombic leaflets (Feruglio Reference Feruglio1951). The present material corresponds very well to this form. Moreover, the gross morphology and dimensions of the present specimens agree well with those of O. sanctae-crucis from the Latady basin (Cantrill & Hunter Reference Cantrill and Hunter2005). Initially considered Early to Middle Jurassic, these latter floras have subsequently been dated as latest Jurassic to earliest Cretaceous based on radiometric ages of detrital zircons (Hunter et al. Reference Hunter, Cantrill and Flowerdew2006). We regard the specimens described as Otozamites sp. Tidwell, Kim & Kimura from the mid-Mesozoic of southern Tasmania (Tidwell et al. Reference Tidwell, Kim and Kimura1987) as being conspecific with O. sanctae-crucis.
A very common and closely comparable Gondwanan species is Otozamites bengalensis Oldham et Morris. This species differs from the material ascribed here to O. sanctae-crucis in having less dense and imbricate foliation, and in having rhombic leaflets with parallel margins for most of the leaflet length (McLoughlin & Pott Reference McLoughlin and Pott2009).
Genus Zamites Brongniart
Type species. Zamites gigas Lindley et Hutton, 1837
Zamites sp.
Material: One specimen, documented in the field.
Description: Fragment of a large pinnately compound leaf with an estimated total length of more than 50 cm and a width of c. 12 cm (Fig. 10c). Leaflets attached to upper surface of rachis, crowded and partly overlapping, inserted at angles between 80 and 70° in the central leaf portion; insertion angles decreasing to c. 50° towards the apex. Leaflets over 5 cm long and up to 1 cm wide, straight, lanceolate with the widest portion in the lower third, tapering symmetrically to form an acute apex; leaflet bases more or less symmetrical, acroscopic basal margin in some cases slightly overlapping the rachis; basiscopic basal margin constricted.
Remarks: The single specimen assigned to Zamites occurred on the weathered surface of a large block of tuffitic siltstone (SHC32) at Shafer Peak that could not be collected. The leaf differs from the other bennettitalean leaves in its large size, symmetrically lanceolate leaflets, and in the symmetrical leaflet bases lacking distinct auricles. We therefore assign the specimen to the genus Zamites (see e.g. Harris Reference Harris1969, Watson & Sincock Reference Watson and Sincock1991). From Antarctica, four species of Zamites have initially been described from the Hope Bay flora, i.e. Z. anderssonii Halle, Z. antarcticus Halle, Z. pachyphyllus Halle, and Z. pusillus Halle (Halle Reference Halle1913, Gee Reference Gee1989). Rees and Cleal (Reference Rees and Cleal2004) included the former three species in Z. antarcticus and placed Z. pusillus in synonymy of Otozamites latior Saporta. Our specimen differs in several aspects from Z. antarcticus: 1) in being two (see Cantrill & Hunter Reference Cantrill and Hunter2005) to even four times (see Gee Reference Gee1989, Rees & Cleal Reference Rees and Cleal2004) wider than previously described specimens, 2) in the lower length/width-ratio of the leaflets (about 5–6:1 compared to 5–12:1 in Z. antarcticus), and 3) in its leaflet margins, which run (sub)parallel for most of the length in Z. antarcticus.
The large size of the present specimen is overall unusual for Jurassic cycadophyte foliage from Gondwana; we are unaware of any previous reports of similarly large leaves. With respect to leaflet size and shape, the most closely comparable species is Zamites quiniae Harris from the Yorkshire Jurassic (Harris Reference Harris1969), but the obscure venation and lack of cuticles hamper more detailed comparisons.
Genus Cycadolepis Saporta emend. Harris
Type species. Cycadolepis villosa Saporta, 1873
Cycadolepis sp.
Material: GIX-CE15-021.
Description: 12 mm long, trapeziform fragment of a scale leaf, 10 mm wide at the base and c. 5 mm in the central portion (Fig. 10d). Outer surface of the leaf convex with fine longitudinal striations. Up to 3 mm long, very dense and fine hairs arising at angles of c. 45° from the lateral margins.
Remarks: This scale leaf with its dense hair cover resembles Cycadolepis thysanota Harris figured by Harris (Reference Harris1969, pl. 1, figs 2 & 3), but differs in being about two times larger. It is interesting to note that the specimen is associated with Otozamites spp., as is C. thysanota in the Yorkshire Jurassic (Harris Reference Harris1969). Two other cycadophyte scale leaves have been described from the Lower Jurassic of Antarctica, i.e. cycadophyte scale leaf types A (= Cycadolepis sp. in Halle Reference Halle1913, Gee Reference Gee1989) and B (Rees & Cleal Reference Rees and Cleal2004). In cycadophyte scale leaf type A hairs become longer towards the apex, and type B differs in its broadly ovate shape and obtuse apex (Rees & Cleal Reference Rees and Cleal2004).
Phylum CONIFEROPHYTA
Order VOLTZIALES
Familia incertae sedis
Genus Schizolepis Braun
Type species. Schizolepis liaso-keuperianus Braun, 1847
Schizolepis sp.
Material: GIX-CN14-022, 023.
Description: Detached 7 mm long and 3 mm wide cone scales. Lower half of scale narrowed into a straight, c. 0.8 mm wide stalk; upper half formed by a convex, cup shaped blade with an obovate outline that is more or less deeply divided into two lobes (Fig. 10e).
Remarks: The principal organization of these specimens, including a basal stalk, an entire median region and an apical portion that is more or less deeply divided into two lobes, is in accordance with that of the voltzialean bract scale complex Schizolepis. Our specimens strongly resemble Schizolepis liaso-keuperianus figured by Harris (Reference Harris1979, pl. 4, figs 1–4, 7 & 8), Kräusel (Reference Kräusel1959, pl. 5, figs 24 & 25), and Corsin (Reference Corsin1973, pl. 1, f). However, the much smaller size of our specimens precludes a definite assignment.
Order CONIFERALES
Familia incertae sedis
Conifer cuticle type 1 (cf. Allocladus sp.)

Fig. 11 Dispersed conifer cuticles from the Lower Jurassic of Shafer Peak, north Victoria Land, East Antarctica. Scale bars = 500 μm. a. Conifer cuticle type 1 (cf. Allocladus sp.). GIX-SHC32-000(022)-$1. b. Conifer cuticle type 1 (cf. Allocladus sp.). GIX-SHC32-000(011)-$1. c. Conifer cuticle type 1 (cf. Allocladus sp.). GIX-SHC32-000(012)-$1. d. Conifer cuticle type 2 (cf. Elatocladus sp.), strongly elongate needle. GIX-SHC32-000(010)-$1. e. Conifer cuticle type 2 (cf. Elatocladus sp.), shoot fragment with helically arranged, adpressed leaves. GIX-SHC32-000(015)-$1. f. Conifer cuticle type 2 (cf. Elatocladus sp.). GIX-SHC32-000(016)-$1. g. Conifer cuticle type 3 (cf. Pagiophyllum sp.), shoot fragment indicating opposite-decussate leaf arrangement. GIX-SHC32-000(018)-$1. h. Conifer cuticle type 3 (cf. Pagiophyllum sp.), isolated needle. GIX-SHC32-000(001)-$1.

Fig. 12 Dispersed conifer cuticles from the Lower Jurassic of Shafer Peak, north Victoria Land, East Antarctica. a. Conifer cuticle type 1 (cf. Allocladus sp.), detail of scarious leaf margin. GIX-SHC32-000(013)-$1. Scale bar = 50 μm. b. Conifer cuticle type 1 (cf. Allocladus sp.), lower cuticle. GIX-SHC32-000(014)-$1. Scale bar = 50 μm. c. Conifer cuticle type 1 (cf. Allocladus sp.), upper cuticle with papillate epidermal cells and densely spaced, randomly oriented stomata. GIX-SHC32-000(014)-$1. Scale bar = 50 μm. d. Conifer cuticle type 1 (cf. Allocladus sp.), densely spaced, randomly oriented stomata on upper leaf surface. GIX-SHC32-000(021)-$1. Scale bar = 25 μm. e. Conifer cuticle type 2 (cf. Elatocladus sp.), cuticle with irregular longitudinal stomatal files. GIX-SHC32-000(016)-$1. Scale bar = 100 μm. f. Conifer cuticle type 2 (cf. Elatocladus sp.), detail showing two transversely oriented stomata. GIX-SHC32-000(019)-$1. Scale bar = 50 μm. g. Conifer cuticle type 3 (cf. Pagiophyllum sp.), lower cuticle with longitudinal files of obliquely oriented stomata. GIX-SHC32-000(001)-$1. Scale bar = 50 μm. h. Conifer cuticle type 3 (cf. Pagiophyllum sp.), detail of Fig. 12g showing two stomata with strongly cutinized, papillate subsidiary cells. GIX-SHC32-000(001)-$1. Scale bar = 25 μm.
Material: Dispersed cuticle fragments: GIX-SHC32-000-(011–014, 021, 022, 030, 031, 034, 037).
Description: Bifacially flattened, epistomatic leaves, rhombic in proximal/distal view (Fig. 11c), lanceolate-falcate in lateral view (Fig. 11a), strongly decurrent, attached with more than half the length; abaxial surface strongly convex (Fig. 11a & b), adaxial surface concave; lateral margins with a scarious wing (Fig. 12a); apex curved, forward pointing. Cuticle showing pronounced dorsiventral differentiation; lower leaf epidermis composed of rows of longitudinal cells (Fig. 12b); cell outlines generally rectangular, commonly curved; anticlinal walls strongly cutinized, straight, smooth; periclinal walls with variously developed thickenings, including: 1) a centrally positioned, longitudinal single ridge or pair of ridges (Fig. 12b), 2) more or less centrally positioned, diffuse thickenings, and 3) continuous longitudinal striae oriented parallel to the leaf margins. Upper leaf cuticle strongly papillate (Fig. 12c); anticlinal walls straight or curved, evenly cutinized; periclinal walls each bearing a single, centrally positioned, solid papilla; stomata densely crowded in longitudinal files, randomly oriented, composed of a complete ring of generally four (in some cases up to six) subsidiary cells and a pair of slightly sunken, weakly cutinized guard cells (Fig. 12c & d); each subsidiary cell with a single solid papillae close to and generally overarching the stomatal pit; some stomatal complexes with a weakly developed Florin ring; ordinary epidermal cells between stomatal files narrow, elongate.
Remarks: These specimens can be easily differentiated from co-occurring conifer remains by: 1) the strongly convex, subrhombic abaxial surface and the broadly triangular, concave adaxial surface, 2) the scarious lateral margins, 3) the adaxial distribution of stomata, and 4) the presence of pronounced solid papillae on subsidiary cells and ordinary epidermal cells on the adaxial surface. The wide triangular outline of the free leaf portion is characteristic for Brachyphyllum. Furthermore, epistomatic Brachyphyllum-type shoots with scarious leaf margins and adaxial, densely crowded stomata have been referred to Allocladus Townrow (Townrow Reference Townrow1967b). The use of this genus has been controversially discussed (e.g. Manum et al. Reference Manum, Bose and Vigran1991). We follow Jansson et al. (Reference Jansson, McLoughlin, Vajda and Pole2008), who regard the genus as being sufficiently well delimited from other Mesozoic conifer genera on the basis of both impressions and compressions. Among the different species assigned to Allocladus, only A. papillosus Banerji et Pal has monocyclic stomata and papillate subsidiary cells (Banerji & Pal Reference Banerji and Pal1986), and is therefore closely comparable to the present material. The majority of Allocladus-type shoots have tentatively been assigned to the Araucariaceae. However, the random orientation of stomata, a complete ring of four to six subsidiary cells, and the pronounced papillate stomatal protection in the present material may alternatively indicate affinities with the Cheirolepidiaceae (e.g. Watson Reference Watson1988).
Conifer cuticle type 2 (cf. Elatocladus sp.)
Figs 11d–f and 12e & f
Material: Dispersed cuticle fragments: GIX-SHC32-000-(003, 010, 015, 016, 019, 027, 032, 033).
Description: Shoot fragments with helically arranged leaves (Fig. 11e); leaf morphology variable, ranging from scale-like and closely adpressed to divergent, falcate to needle-like elongate (Fig. 11d & f); scale-like leaves bifacial, rhombic in proximal/distal view, up to 2 mm long by c. 1.5 mm wide, widest below the middle; slightly falcate in lateral view, with a decurrent base and a recurved, pointed tip; free leaf portion triangular in proximal/distal view, lateral margins without wings (Fig. 11e). Divergent leaves exceeding 3.5 mm in length, ranging from elongate, rhomboidal–falcate (c. 1 mm wide; Fig. 11f) to straight and linear (0.5 mm wide; Fig. 11d); apices acute.
Cuticle apparently uniformly developed over the entire leaf, epidermal cells aligned in longitudinal rows, generally rectangular in outline, in fewer cases polygonal. Anticlinal walls straight to slightly curved, cutinization smooth and regular, or with tuberculate thickenings and irregular interruptions; periclinal walls generally smooth, in few cases with faint longitudinal striations or bearing a single, indistinct, solid papilla. Up to twelve irregular and discontinuous longitudinal rows of stomata per leaf (Fig. 11f). Stomatal complexes generally widely separated from each other, randomly oriented (Fig. 12e), composed of a pair of sunken guard cells, a surrounding ring of four to six, slightly sunken and weakly cutinized subsidiary cells with pronounced distal thickenings, and a second, complete or incomplete ring of neighbouring cells with usually thickened anticlinal walls (Fig. 12f); subsidiary cells lacking papillae. Stomatal pit elongate-rectangular, 10–35 μm long and generally much narrower.
Remarks: The above described different leaf morphologies probably belong to a single, variable taxon because the cuticles are essentially similar. It is interesting to note that two other morphotaxa of heterophyllous conifers from the Jurassic of Antarctica encompass a very similar range of leaf shapes: the impression taxon Elatocladus confertus Halle (e.g. Halle Reference Halle1913, Gee Reference Gee1989, Rees & Cleal Reference Rees and Cleal2004), and the cuticle bearing compression taxon Nothodacrium warrenii (Plumstead Reference Plumstead1962, Townrow Reference Townrow1967a). In fact, the main difference between these two taxa is apparently the preservation of cuticles and attached seed cones in the latter. Affinities with the Podocarpaceae have been assumed for both N. warrenii and E. confertus. The present material, however, is unlikely to represent a podocarpaceaen cuticle because of the random orientation of stomata. In extant podocarp conifers stomata are predominantly oriented parallel to the leaf length (Pole Reference Pole2000).
Conifer cuticle type 3 (cf. Pagiophyllum sp.)
Figs 11g & h and 12g & h
Material: Dispersed cuticle fragments: GIX-SHC32-000-(001, 004, 008, 023, 035).
Description: Leaves decussate (Fig. 11g), short, thick, up to 2 mm long and 1 mm wide, amphistomatic. Abaxial leaf surface convex with a decurrent base (Fig. 11g), keeled in lower half, subrhombic in proximal/distal view, attached over more than half the leaf length; leaf cushion subrhombic in proximal view; adaxial surface concave, narrow-triangular in proximal view, c. 0.6 mm wide at base, tapering to a slightly curved, obtuse apex; lateral margins with a scarious wing composed of a short row of fused cells.
Cuticle uniformly developed over the entire leaf surface; epidermal cells aligned in longitudinal rows (Fig. 12g & h), rectangular to rarely polygonal, c. 30–80 μm long; anticlinal walls straight or curved, evenly cutinized; most ordinary epidermal cells with a single, solid papilla positioned generally closer to the acroscopic side (Fig. 12g). Stomatal complexes arranged in up to twelve discontinuous longitudinal rows (Figs 11h & 12g), generally obliquely oriented (Fig. 12g), closely spaced; stomata absent on the abaxial keel. Stomatal complexes almost circular to star shaped in outline, composed of a pair of sunken guard cells partly overlain by a complete ring of four to six subsidiary cells (Fig. 12g & h); subsidiary cells stronger cutinized than ordinary epidermal cells, each bearing a single solid papilla close to and generally overarching the stomatal pit (Fig. 12h). In few cases two adjacent stomata share one subsidiary cell. Stomatal pit oval in outline, 20–30 μm wide.
Remarks: The most complete shoot fragment bears three leaves in decussate phyllotaxy, indicating affinities with Cupressaceae, Podocarpaceae, or Cheirolepidiaceae. A cheirolepidiaceaen affinity for these cuticles appears probable based on the random orientation of stomata and the strongly papillate subsidiary cells (e.g. Okubo & Kimura Reference Okubo and Kimura1991). However, the presence of subsidiary cells shared by two adjacent stomatal complexes is a feature typically occurring in representatives of the Cupressaceae sensu stricto (see e.g. Okubo & Kimura Reference Okubo and Kimura1991).
Discussion
Even though the new Antarctic localities could only be sampled by short visits, the macrofloras of the Shafer Peak Formation constitute the richest and most diverse Jurassic plant fossil assemblages so far recovered from East Antarctica. The preservation of cuticles is most remarkable, because cuticles are very rare in the Jurassic of southern Gondwana. Cuticles may be helpful for detailing biological affinities and interpreting palaeoenvironmental adaptations of fossil plants (e.g. Kerp Reference Kerp1990).
Comparisons with other Gondwanan Jurassic floras
The majority of plant fossil taxa from the Shafer Peak Formation are widespread and stratigraphically long ranging in the mid-Mesozoic of southern Gondwana. The dominant genera in the present collection are Equisetites (Equisetum), Coniopteris, and Otozamites, all of which are very common also in other Jurassic floras of Antarctica (e.g. Halle Reference Halle1913, Gee Reference Gee1989, Cantrill Reference Cantrill2000, Rees & Cleal Reference Rees and Cleal2004, Cantrill & Hunter Reference Cantrill and Hunter2005), Australia (e.g. Gould Reference Gould1974, Tidwell et al. Reference Tidwell, Kim and Kimura1987, McLoughlin & Pott Reference McLoughlin and Pott2009), and New Zealand (e.g. Edwards Reference Edwards1934). The few dispersed conifer cuticles obtained are difficult to compare with other conifer assemblages from the Gondwana Jurassic because of the fragmentary preservation. However, shoot and leaf morphologies agree well with those of the conifer leaf morphotaxa Brachyphyllum, Pagiophyllum, and Elatocladus, which are all ubiquitous components of Jurassic Gondwanan floras (e.g. Halle Reference Halle1913, Cantrill Reference Cantrill2000, Cantrill & Hunter Reference Cantrill and Hunter2005, McLoughlin & Pott Reference McLoughlin and Pott2009). Matoniaceous ferns are less widespread, and have previously only been reported from the Botany Bay Group (Rees & Cleal Reference Rees and Cleal2004) and from eastern Australia (Gould Reference Gould1974) with a further tentative record from Western Australia (McLoughlin & Pott Reference McLoughlin and Pott2009). The occurrence of exceptionally large Zamites leaves at Shafer Peak is also unusual. To our knowledge such large cycadophyte foliage is so far unknown from the Gondwanan Jurassic. Furthermore, Clathropteris is exceptionally rare in the Southern Hemisphere, and has been reported from only four localities in Argentina and East Antarctica (e.g. Yao et al. Reference Yao, Taylor and Taylor1991, Escapa et al. Reference Escapa, Cúneo and Cladera2008, Bomfleur & Kerp Reference Bomfleur and Kerp2010). In addition, the here described bract scales Schizolepis sp. represent the first record of this genus from Gondwana.
The macrofloral data available from the Shafer Peak Formation are based on a relatively small suite of specimens that was collected in only a few days. Nevertheless, the plant fossil assemblages have a similar overall composition to other Jurassic floras of Gondwana, which further demonstrates the remarkable homogeneity and longevity of Southern Hemisphere vegetation during the mid-Mesozoic. It remains difficult to interpret the observed differences in composition. These may rather reflect preservation and collection bias in the Jurassic fossil record of Gondwana in general, and from Antarctica in particular, rather than a distinct phytogeographic pattern.
Palaeoclimatological and palaeoenvironmental interpretations
Jurassic palaeoclimate reconstructions still suffer from the notorious lack of data from southern Gondwana, especially from East Antarctica (e.g. Vakhrameev Reference Vakhrameev1991, Rees et al. Reference Rees, Ziegler and Valdes2000). The macrofloras from the Shafer Peak Formation fill an important gap in the Gondwanan macrofossil record, and thus may help to better refine Jurassic palaeoclimate reconstructions and palaeophytogeographic patterns.
During the Early Jurassic, the Transantarctic basin was situated between c. 60 and 70°S, fringing the East Antarctic craton behind the active Panthalassan margin of southern Gondwana (e.g. Golonka Reference Golonka2007, Torsvik et al. Reference Torsvik, Gaina and Redfield2008). There is general consensus that Jurassic climates were characterized by ‘hothouse’ conditions, with ice-free poles and decreased latitudinal temperature gradients (e.g. Scotese et al. Reference Scotese, Boucot and McKerrow1999, Rees et al. Reference Rees, Ziegler and Valdes2000, McLoughlin Reference McLoughlin2001). Previous palaeoclimatic reconstructions have overall agreed in that the Transantarctic basin was located in an extended warm temperate climatic belt, experiencing humid and mesothermal to megathermal conditions during the Early Jurassic (e.g. Vakhrameev Reference Vakhrameev1991, Parrish Reference Parrish1993, Hallam Reference Hallam1994, Scotese et al. Reference Scotese, Boucot and McKerrow1999). However, more recent models predict a cool temperate biome for the continental interior of southern Gondwana, which is based mainly on the assumption that Jurassic climate zones were distributed symmetrically along the equator (Rees et al. Reference Rees, Ziegler and Valdes2000). Whereas previous palaeoclimatic reconstructions have relied heavily on the presence/absence of a few individual indicator plant taxa (e.g. Vakhrameev Reference Vakhrameev1991), Rees et al. (Reference Rees, Ziegler and Valdes2000) established a more rigorous ‘whole-flora’ approach. Rees et al. (Reference Rees, Ziegler and Valdes2000) assigned 32 Jurassic leaf genera to five broadly defined morphological categories in order to assess their significance for palaeoclimatological interpretations. The two resulting end members are floras dominated by small-leaved cycadophyte and conifer taxa (i.e. Zamites, Otozamites, Brachyphyllum) indicating warm temperatures and commonly dry conditions, and floras of ginkgophytes and broad-leaved conifers (e.g. Phoenicopsis Heer, Czekanowskia Heer, Podozamites Braun) prevalent in cooler, presumably higher latitude biomes. Applied to the present material, the Shafer Peak Formation floras would be characteristic for a warm climatic regime, refuting most recent model predictions. This may be further supported by the dominance of cheirolepidiaceous pollen (Classopollis Pflug) in Jurassic microfloras from north Victoria Land (Bomfleur et al. unpublished data). Cheirolepidiaceous conifers are traditionally regarded as thermophilic elements (e.g. Watson Reference Watson1988). Dry conditions in this part of Gondwana, however, are neither indicated by palaeoclimate models nor by sedimentological data (e.g. Hallam Reference Hallam1994, Parrish Reference Parrish1993, McLoughlin Reference McLoughlin2001). In the Shafer Peak Formation sedimentary features indicating (seasonally) dry conditions, such as mudcracks, evaporites, caliche/calcrete, and aeolian sand are absent (Schöner et al. unpublished data). In contrast, common Planolites Nicholson burrows on bedding planes document high water tables (Schneider et al. unpublished data), and Otozamites, one of the dominant plants, presumably favoured high humidity as well (Wang et al. Reference Wang, Ni, Jiang and Tian2008). Altogether, the new palaeobotanical and sedimentological data provide further evidence for warm temperatures and overall humid atmospheric conditions in south-eastern Gondwana during the Early Jurassic.
It is interesting to note that, regardless of this favourable setting, plant growth did not result in peat accumulation and coal formation. We suggest this may have been due to overall landscape instability caused by the intense Early Jurassic volcanism in the Transantarctic basin. Sediment supply was governed by a massive input of wind-blown rhyolitic ashes (Schöner et al. Reference Schöner, Viereck-Götte, Schneider and Bomfleur2007), resulting in sediment overload and poorly drained, cohesive substrates. Simultaneously, shallow-level sill intrusions during the Early Jurassic transformed the Transantarctic basin into an extensive phreatomagmatic province (Ross et al. Reference Ross, Ukstins-Peate, McClintock, Xu, Skiling, White and Houhgton2005, Elliot & Fleming Reference Elliot and Fleming2008). This is evidenced by the widespread occurrences of mafic volcaniclastic deposits, which were produced in local hydrovolcanic eruption centres by violent water-magma interactions when ascending sills reached groundwater (e.g. Ross et al. Reference Ross, Ukstins-Peate, McClintock, Xu, Skiling, White and Houhgton2005, Viereck-Götte et al. Reference Viereck-Götte, Schöner, Bomfleur and Schneider2007, Elliot & Fleming Reference Elliot and Fleming2008). As a consequence, landscape and vegetation patterns must have experienced repeated perturbation through a wide array of catastrophic events related to phreatomagmatism (e.g. Bateman & Scott Reference Bateman and Scott1990, Orton Reference Orton1996). Apart from immediate devastating events such as hydrovolcanic blasts and base surges, secondary processes must have had strong effects on landscape evolution. For example, volcanic activity was commonly associated with wildfires. This is evidenced by common charred plant debris and wood (e.g. Scott Reference Scott2010), which at least at Mount Carson co-occur with juvenile mafic clasts indicating contemporaneous volcanism. Volcanically induced wildfires may result in clearing of local vegetation and destabilization of substrates, thus greatly increasing erosion rates and promoting gravity driven mass movements, i.e. debris flows and sheet floods (Bateman & Scott Reference Bateman and Scott1990, Orton Reference Orton1996, Lovelace Reference Lovelace2006). This may have been further enhanced by heavy rainfall, which is also commonly associated with volcanic eruptions due to atmospheric seeding with ash particles (see Bateman & Scott Reference Bateman and Scott1990). Mass transport is indicated by the random orientation of plant fossils floating in the matrix at Shafer Peak and Mount Carson north. At Mount Carson east sphenophyte rhizomes and aerial stems, isoetalean leaves, and abundant clay-gall rip-up clasts are concentrated at the base of the CEL15 bed. We interpret this assemblage as reflecting rapid erosion and redeposition of non-carbonaceous overbank deposits that formed in ephemeral pools colonized by (semi)aquatic plants. Altogether, Early Jurassic vegetation in this part of East Antarctica was presumably in a constant flux, and composed of a complex mosaic of local plant communities at different successional stages. The lack of any evidence for soil development and peat accumulation in the Shafer Peak Formation may indicate that the re-occurring disturbances hampered the establishment of persistent climax communities.
Palaeoecological significance of the plant cuticles
Fossil plant cuticles may reflect certain palaeoenvironmental conditions that their parent plants experienced, and can therefore contribute towards more accurate reconstructions of palaeoenvironments (e.g. Busche et al. Reference Busche, Hass and Remy1978, Kerp Reference Kerp1990). The cuticles of Otozamites linearis and the three conifers show pronounced xeromorphic features. For instance, O. linearis is hypostomatic, and stomata are commonly sunken in deep epistomatal chambers that are additionally protected by overarching dendroid papillae. Stomata in all conifers are sunken as well. Pronounced papillate stomatal protection occurs in two of the three conifers. In addition, conifer cuticle type 1 is epistomatic, with stomata restricted to the strongly protected upper leaf surface of the adpressed, scale-like leaves. Such xeromorphic features, however, do not necessarily reflect dry atmospheric conditions (e.g. Busche et al. Reference Busche, Hass and Remy1978, Kerp Reference Kerp1990, Haworth & McElwain Reference Haworth and McElwain2008).
With respect to the conifer cuticles, xeromorphic features may simply be due to an evergreen habit of the parent plants. The vast majority of conifers in polar latitudes of the Mesozoic greenhouse world were probably evergreen (Osborne et al. Reference Osborne, Royer and Beerling2004), including those bearing Brachyphyllum-, Pagiophyllum-, and Elatocladus-type foliage comparable to the present material (Falcon-Lang & Cantrill Reference Falcon-Lang and Cantrill2001). In high latitude evergreen trees, xeromorphic adaptations serve to prevent excessive loss of water and carbon during the long lasting dark, yet warm conditions of polar night (e.g. Falcon-Lang & Cantrill Reference Falcon-Lang and Cantrill2001, Nagalingum et al. Reference Nagalingum, Drinnan and McLoughlin2005). It should also be considered that xeromorphic adaptations may be essential for plants growing under overall humid conditions that are only punctuated by erratic, short dry phases, which may not always be reflected in sedimentary features.
However, the xeromorphic features of the plant cuticles may also be pre-adaptations that proved beneficial for growth in volcanically active environments. For instance, xeromorphic traits reducing water loss through stomatal evapotranspiration, such as sunken stomata and papillate stomatal protection, may also serve to minimize uptake of volcanogenic atmospheric pollutants (e.g. Haworth & McElwain Reference Haworth and McElwain2008). Furthermore, an important function of papillae and other cuticle microrelief is to alter leaf surface wettability, e.g. in order to hamper formation and retention of water droplets, or to increase water runoff rates and the self-cleaning effect of leaves. All such processes ultimately have a profound effect on stomatal conductance, assimilation rate, and leaf lifespan (e.g. Neinhuis & Barthlott Reference Neinhuis and Barthlott1997, Pott et al. Reference Pott, Krings and Kerp2007). In this context it is interesting to note that the Otozamites leaves have densely spaced to imbricate foliation. Some xeric plants benefit from imbricate leaves by collecting and directing water flow towards the leaf base (e.g. Krings et al. Reference Krings, Klavins, DiMichele, Kerp and Taylor2005). However, this is apparently not the case in Otozamites because leaflet overlap is inversely arranged (i.e. acroscopic auricles and leaflet portions overlap the bases of the superpositioned leaflets), directing a potential water flow towards the apex. We suggest this feature has rather enhanced runoff rate and the self-cleaning effect of the leaves, which is particularly important for plants growing in volcanic environments, where accumulation of deleterious volcanogenic particles on the leaf surface can have very detrimental effects (e.g. Haworth & McElwain Reference Haworth and McElwain2008).
Conclusions
In several ways the new Jurassic macrofloras from north Victoria Land fill a crucial gap in the Southern Hemisphere plant fossil record. Our results underscore the large discrepancies between modelled high latitude palaeoclimates and palaeobotanical evidence, demonstrating the need for further refinement of model simulations. Of particular significance is the exceptional preservation of plant fossils. The combination of cuticle preservation and permineralization in the Lower Jurassic of East Antarctica may constitute a key source of information on the biological affinities and the palaeoecology of Gondwanan Jurassic plants that are still largely known from impression/compression material only. For instance, the cuticles of Otozamites linearis enabled us to recognize a very broad intraspecific variability. Such observations may ultimately lead to a much more accurate, biological characterization of fossil plant taxa. Analysis of cuticles can also greatly contribute to detailing palaeoenvironment reconstructions. Altogether, we suggest that future systematic collecting in the still very poorly sampled Lower Jurassic of the Transantarctic Mountains may play a pivotal role in our understanding of mid-Mesozoic palaeoclimates, palaeobiology and palaeoecology of past high latitude biotas.
Acknowledgements
BB wishes to thank the Bundesanstalt für Geowissenschaften und Rohstoffe (BGR), Hannover, for the invitation to join the GANOVEX IX expedition, and J. Schneider (Freiberg), R. Schöner (Jena), and L. Viereck-Götte (Jena) for a fruitful and most enjoyable collaboration in the field. We thank J.E. Francis, D.J. Cantrill, M. Krings, and A.P.M. Vaughan for valuable comments and discussions on the manuscript. Technical support by the Alfred-Wegener-Institut für Meeres- und Polarforschung (AWI), Bremerhaven, is gratefully acknowledged. We thank T. Grund (Münster) and P. Moisan (Münster) for assistance in SEM imaging. This study was funded by the Deutsche Forschungsgemeinschaft (DFG), grants KE584/12-1, KE584/16-1 and 16-2.