Introduction
Antarctica has a number of active volcanoes including Mount Erebus, Mount Melbourne and Mount Rittman all within the Ross Dependency, Victoria Land. The continent has been separated from all other volcanic habitats for over 30 million years (Barker & Burrell Reference Barker and Burrell1977), and the volcanic soils are exposed to a combination of extreme environmental conditions, including low nutrient availability, limited oxygen, prolonged periods of darkness, and extreme chemical and temperature gradients (Parish & Bromwich Reference Parish and Bromwich1991, Hansom & Gordon Reference Hansom and Gordon1998, Soo et al. Reference Soo, Wood, Grzymski, McDonald and Cary2009). Mount Erebus hosts the only phonolitic lava lake on the planet, and experiences up to ten strombolian eruptions per day (Calkins et al. Reference Calkins, Oppenheimer and Kyle2008). Tramway Ridge is a gently sloping, extensively mineralized, ice-free area of geothermally altered heated soil located 1.5 km north-west of the main crater of Mount Erebus, at an elevation of 3350 m a.s.l. The ridge has some of the hottest surface soil found on the mountain, maintaining an annual average temperature of 65°C in localized fumaroles (Soo et al. Reference Soo, Wood, Grzymski, McDonald and Cary2009). The scientific value of this habitat lies in the unique biological systems identified in early research (Broady Reference Broady1984, Skotnicki et al. Reference Skotnicki, Selkirk, Broady, Adam and Ninham2001, Lesser et al. Reference Lesser, Barry and Banaszak2002). As such the area was designated an Antarctic Specially Protected Area (ASPA) in 1985. The unique location and nature of this ecosystem provides a great opportunity to study novel biodiversity, unique adaptations and evolutionary processes in an isolated context (Hudson et al. Reference Hudson, Daniel and Morgan1989).
Previous cultivation dependent approaches (Hudson & Daniel Reference Hudson and Daniel1988, Melick et al. Reference Melick, Broady and Rowan1991) conducted on the Tramway Ridge geothermal soils had success in isolating unique organisms. The application of 16 S rRNA gene cloning to investigate the community diversity (Soo et al. Reference Soo, Wood, Grzymski, McDonald and Cary2009) revealed that these isolates were not dominant members of the hottest (65°C) soils. The cloning efforts did however reveal a unique microbial community with all bacterial sequences showing less than 92% similarity with their closest known relatives in the NCBI database. The largest bacterial clade within the community branched within an unknown group loosely related to Armatimonadetes and Chloroflexi phyla. Recently, pyrosequencing has supported these findings and described the community in more detail (Herbold et al. Reference Herbold, Lee, McDonald and Cary2014).
A community-based cultivation strategy was devised in an attempt to enrich for the dominant representatives in the 65°C soils. Responses and metabolic requirements of the Tramway Ridge microbial community to different nutrient treatments were analysed using a combination of molecular genetic approaches (DNA fingerprinting and 454 pyrosequencing) and phenotypic microarrays (Biolog). Physicochemical analysis of the 65°C soil was also conducted to understand the environmental factors influencing the microbial community.
Materials and methods
Sample collection
Samples were collected from 60–65°C geothermal fumaroles at Tramway Ridge (ASPA 130). Samples for preliminary genetic analysis and geochemical characterization were collected from three sites (1–3) in February 2009, and were immediately frozen and maintained at -80°C until analysed in the laboratory. Samples for Biolog experiments were collected at site 3 in November 2009, and samples for the enrichment experiment were taken from site 3 in November 2010. Soil was collected aseptically from three depths (0–2 cm, 2–4 cm and 4–8 cm) at the same fumarole using a sterile metal spatula in 50 ml sterile CELLSTAR centrifuge tubes (Greiner Bio One, Germany). Samples for phenotypic arrays and enrichment studies were maintained at 65°C in a thermally regulated transport container until experiments were initiated, within an hour of collection.
Characterization of edaphic qualities
Soil oxygen concentration was determined at each site using a Fibox 3 LCD trace minisensor oxygen meter with data-logger (PreSens Precision Sensing, Germany), calibrated for soil temperature and altitude. Subsurface gasses from the soil were drawn into a low volume chamber containing the sterilized oxygen probe. On site temperature measurements were taken using a hand-held Checktemp1C thermometer (Hanna Instruments). Analyses of pH/conductivity, carbon, nitrogen, moisture and elements were conducted as described in Lee et al. (Reference Lee, Barbier, Bottos, McDonald and Cary2012) and Tiao et al. (Reference Tiao, Lee, McDonald, Cowan and Cary2012).
Biolog community-based phenotypic microarray experiment
Biolog experiments were initiated at Lower Erebus Hut (LEH), located 1 km north-east of Tramway Ridge, within one hour of sample collection. Soil from all three depths of site 3 were combined and homogenized to allow sufficient biomass for inoculation by mixing with a sterile spatula, followed by vortexing for 10 seconds. The soil inoculum was prepared by adding 15 g of 65°C Tramway Ridge soil to a 50 ml sterile CELLSTAR centrifuge tube. Soil was thoroughly homogenized by adding 40 ml of sterile H2O and vortexing for 30 min. The soil inoculum was left to settle for 5 minutes before transferring 30 ml of the supernatant (cellular inoculum) to a new centrifuge tube. The final stock inoculum for each plate consisted of 20 ml IF-Oa, 0.24 ml dye mix, 2 ml cellular inoculum and 2 ml of the relevant phenotypic microarray (PM) additive solution (as per manufacturer’s instructions). The inoculum stock fluid (100 µl) was dispensed into individual wells of the PM plate. Five different PM plates (Biolog, Hayward, CA, USA) were used: two different carbon plates (PM1 and 2), a potassium and sulfur plate (PM3), a nitrogen plate (PM4) and a pH plate (PM10). All PM plates were inoculated in triplicate to ensure reproducibility. Plates were incubated at 65°C under both oxic and sub-oxic conditions and examined each day for evidence of respiration (visually conspicuous colour development in wells from clear to purple). Sub-oxic incubation was achieved by placing the plates in an airtight box, purging the box with Argon gas and placing non-catalyst, oxygen-consuming, CO2-generating Campy Packs (Hardy Diagnostics, California, USA) in the box before it was sealed.
Community enrichment
Enrichment experiments were also initiated at LEH within one hour of sample collection. Soil from all three depths were combined to allow sufficient initial biomass for enrichment cultures and homogenized by mixing with a sterile spatula, followed by vortexing for 10 seconds. From the homogenized sample, 5 g aliquots were dispensed into 15 ml sterile CELLSTAR centrifuge tubes for nutrient treatment and incubation. The two time zero (T0) tubes were immediately frozen at -20°C. The remaining duplicate tubes were supplemented with 1 ml of the corresponding nutrient solution (Table I). All nutrient solutions were prepared in sterile Milli-Q H2O. Tubes were then sealed and incubated at 65°C. After nine days, 1 g of soil was removed from each tube using a sterile spatula, transferred to a sterile 2 ml screw cap tube and frozen at -20°C. Each treatment tube was then supplemented with an additional 1 ml of nutrient solution, sealed and incubated for a further 13 days (day 22). After incubation, another 1 g of soil was removed from each tube and frozen as described above. Samples were transported back to New Zealand for DNA extraction and molecular analysis. DNA extraction was conducted as described by Barrett et al. (Reference Barrett, Virginia, Wall, Cary, Adams, Hacker and Aislabie2006) and DNA was quantified using a Qubit 2.0 fluorometer (Invitrogen, New Zealand). Soil pH controls were also implemented under enrichment conditions: 5 g aliquots of soil were dispensed into three centrifuge tubes with 1 ml of Milli-Q water. No additional nutrients were added and each sample was measured in duplicate. Prior to incubation, samples were pH tested as described in Tiao et al. (Reference Tiao, Lee, McDonald, Cowan and Cary2012) and Lee et al. (Reference Lee, Barbier, Bottos, McDonald and Cary2012) to give a T0 pH reading of soil. Tubes were then incubated at 65°C for 9 days, pH tested and incubated for a further 13 days with a final pH testing.
Table I Nutrient treatments for enrichment experiments.

Automated ribosomal intergenic spacer analysis
Automated ribosomal intergenic spacer analysis (ARISA) was used as a preliminary fingerprinting tool to identify differences in composition of bacterial communities after enrichment. The internal transcribed spacer region of the bacterial rRNA operon was amplified using PCR from total community DNA of each sample. The PCR components and conditions for ARISA, and quality control procedures for PCR amplicons, were carried out as described in Lee et al. (Reference Lee, Barbier, Bottos, McDonald and Cary2012). The PCR products were diluted 1:20 in Milli-Q H2O and analysed at the University of Waikato DNA Sequencing Facility.
For analysis of ARISA profiles, all peaks present between 100 and 1200 bp that were larger than 50 relative fluorescent units were accepted as peaks, and peaks were binned (width=1) (Abdo et al. Reference Abdo, Schüette, Bent, Williams, Forney and Joyce2006). In each community profile, the abundance of each accepted peak was expressed as a proportion of the total of all peak heights accepted in the profile. These profiles were analysed using Primer 6 software (PRIMER-E, UK). Bray-Curtis distances between each sample were used to compare and cluster samples. The total number of peaks from each sample was used as a proxy for biodiversity.
454 pyrosequencing
Six samples that best represented the trends apparent in the fingerprint data were selected for sequencing using the Roche GS Junior Ti-454 pyrosequencing platform. Each sample was PCR amplified in triplicate and 20 ng genomic DNA was used as a template. The PCR reactions, analysis, purification and quantification were modified from Lee et al. (Reference Lee, Barbier, Bottos, McDonald and Cary2012). Modifications include 0.02 U Primestar Taq (Takara Holdings, Japan). A second round of triplicate PCR was run as above but with only ten cycles and using 25 ng of the purified DNA from the previous step per reaction (Milli-Q H2O volume adjusted accordingly). Primers contained adapters for one-way reads according to the Roche GS Junior System Guidelines for Amplicon Experimental Design Manual (August 2010), including unique MID identifiers for each sample (BacX-Tx9F, 5’-CCATCTCATCCCTGCGTGTCTCCGACTCAG-MID-GGATTAGAWACCCBGGTAGTC-3’ and BacB-1391R, 5’-CCTATCCCCTGTGTGCCTTGGCAGTCTCAG-GACGGGCRGTGWGTRCA-3’). A second gel extraction and AMpure clean up were performed as above. Sample DNA content was quantified using a Qubit Fluorometer, and then diluted to 1×109 molecules μl-1 as per the Roche Amplicon Library Preparation Method Manual (GS Junior Titanium Series, May 2010 (Rev. June 2010)). QPCR using a KAPA Library Quantification Kit for Roche 454 Titanium/Universal (Kapa Biosystems, Woburn, MA, USA) was used to check the 1×109 dilution and was adjusted accordingly for making the amplicon library. The diluted amplicons were mixed together in the desired proportions to create the 1×109 amplicon pool. Sequencing was performed using the GS Junior Titanium emPCR Kit (Lib-L), the GS Junior Titanium Sequencing Kit, PicoTiterPlate Kit and GS Junior System (Roche 454 Life Sciences, Branford, CT, USA) at The University of Waikato DNA Sequencing Facility.
454 pyrosequencing data processing
454 PCR amplicon pyrosequencing data was denoised using AmpliconNoise v1.0 (Quince et al. Reference Quince, Lanzen, Davenport and Turnbaugh2011) and unique reads observed with a frequency lower than three were discarded. Distances between the remaining unique reads were calculated using pairwise Needleman alignments in ESPRIT (Sun et al. Reference Sun, Cai, Liu, Yu, Farrell, McKendree and Farmerie2009). Mothur 1.17.0 (Schloss et al. Reference Schloss, Westcott, Ryabin, Hall, Hartmann, Hollister, Lesniewski, Oakley, Parks, Robinson, Sahl, Stres, Thallinger, van Horn and Weber2009) was used to cluster reads into operational taxonomic units (OTUs) defined at a distance of 0.03 using furthest neighbour clustering (OTU0.03). Sequences were classified in Mothur with the November 2012 release of the Greengenes database and taxonomy (McDonald et al. Reference McDonald, Price, Goodrich, Nawrocki, DeSantis, Probst, Andersen, Knight and Hugenholtz2012). Classification of amplicons to Candidate Division OctSpA1-106 was made for amplicons that matched sequence AF015925 (Blank et al. Reference Blank, Cady and Pace2002) with>97% identity, >99% of amplicon length. For diversity calculations and comparative analysis, the dataset was resampled (bootstrapped) 1000 times with a sample size of 1000 from each sample. Evenness was calculated as described by Schloss et al. (Reference Schloss, Westcott, Ryabin, Hall, Hartmann, Hollister, Lesniewski, Oakley, Parks, Robinson, Sahl, Stres, Thallinger, van Horn and Weber2009).
Results
Tramway Ridge edaphic characteristics
The edaphic characteristics of three fumarolic sites were determined (Table II) and considered in a principal co-ordinate analysis (Fig. 1). All depths from site 3 and the non-surface samples from site 2 form a cluster indicating a relatively common physicochemistry; however, the geochemistry at site 1 showed much greater variation between depths. The first co-ordinate axis accounted for 46% of the physicochemical variance between samples, and sample placement in the primary axis is correlated to sample depth (Spearman correlation r=-0.738, P=0.023). The secondary axis explains 28.1% of the variance in physicochemistry, and approximately divides samples according to site (ANOVA test P=0.05934). This apparent difference is driven primarily by differences between sites 1 and 2 (Tukeys Honest Significant difference P=0.0518). Site 3 is less unique, exhibiting no significant difference in placement along the secondary axis from either site 1 (Tukeys Honest Significant difference P=0.1886) or site 2 (Tukeys Honest Significant difference P=0.3106). Subsurface soils were found to be significantly oxygen depleted, but not strictly anaerobic i.e.≤1.2 ppm oxygen within soil, compared to≥9.7 ppm oxygen in the atmosphere. The pH of soil samples ranged from pH 7.5 to 8.1.

Fig. 1 Principle co-ordinate plot showing physicochemical similarity between sites sampled in this study. Of the total observed physicochemical variance, 74% is summarized in the first two co-ordinate axes.
Table II Soil characteristics.
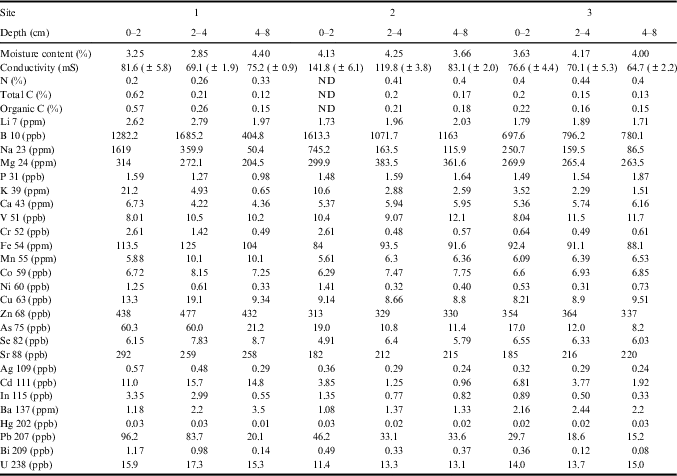
*S 34 and Tl 205 were below the detection limit.
Biolog community-based phenotypic array experiment
The PM plates indicated that most respiration of the Tramway Ridge microbial community occurred under strongly alkaline conditions in sub-oxic environments (Table III). In this experiment, microaerophilic growth revealed a broader range of metabolic capability compared to aerobic growth, and identified microbial activity on compounds such as inulin, 2,3-butanone, urea and several amino acids including alanine, arginine, histidine, leucine, lysine, methionine, ornithine, phenylalanine, proline, serine, threonine, tryptophan, tyrosine and valine.
Table III Biolog phenotypic microarray (PM) positive respiration response to substrates.

Initial Tramway Ridge microbial community
The fumarole-associated microbial community in the combined soil sample from site 3 used for enrichment experiments (the T0 community) was characterized using ARISA and next-generation sequencing (NGS) of amplicons. A total of 91 unique OTUs were identified using NGS, and 67 peaks were identified using ARISA (Table IV). Bacterial evenness of the T0 community was 0.52, and the most abundant OTU (12% relative abundance) in the T0 community was classified as a member of the GAL35 class of candidate division OP1. Other prominent taxa included Chloroflexi, Meiothermus, Cyanobacteria, Armatimonadetes and a diverse assemblage of unclassified OTUs.
Table IV Diversity measurement of treatment samples using sequencing analysis.

* Dataset was resampled (bootstrapped) 1000 times with a sample size of 1000 from each sample.
Enrichment experiment
Thirteen nutrient treatments were selected for the community enrichment studies (Table I). Treatments were selected for their potential to i) enrich, for taxa involved in nitrogen cycling identified during the preliminary analysis of the original (T0) community including Planctomycetes (chitin and N-acetylglucosamine), Nitrospira (nitrite/nitrate) and Thaumarchaeota (bicarbonate and ammonium), ii) be utilized by the community via the Biolog plate experiment (sulfate, sulfide, ammonium oxalate, inulin, casamino acids and trypticase peptone), or iii) exert osmotic stress (salts and inositol).
After enrichment, ARISA analysis showed that community profiles from each treatment clustered into three primary groups, and a singleton outlier: a NO2/NO3 day 22 treatment (Fig. 2). The two largest groups corresponded approximately to day 9 and day 22 communities. Four samples clustered into the third group: the T0 community, and both day 9 and one day 22 bicarbonate treatments. Analysis showed that multiple enrichment conditions induced a similar community response, with communities clustering based on length of enrichment (9 or 22 days), rather than nutrient treatment. The exceptions were the bicarbonate and NO2/NO3 treatments (Fig. 2). For bicarbonate: three treatments grouped with the T0 community profile, while one of the day 22 treatments grouped with the day 9 profiles. For NO2/NO3 treatment: one day 9 treatment grouped with the day 22 communities, and one day 22 treatment was an outlier. Samples representing the three primary clusters identified by ARISA were selected for further analysis by pyrosequencing.

Fig. 2 Clustering of ARISA peak distributions for each sample. All treatment samples were collected from site 3.
Pyrosequencing revealed that, with the exception of the bicarbonate treatment, diversity and evenness in treatment communities decreased in respect to the original diversity at T0 (Table IV). Bicarbonate treatments were the only samples that maintained a community structure that did not significantly differ from that at T0 (Fig. 3 and Table IV). The drop in diversity of non-bicarbonate treatments resulted from a reduction of many dominant members of the original community (Fig. 3). The most apparent reductions were in the Armatimonadetes/OP10, Cyanobacteria, and a diverse assemblage of OTUs that are classified as Chloroflexi with low confidence (40±15% confidence in assignment) (Fig. 3). Each of the non-bicarbonate treatment communities were dominated (68–88%) by a single OTU with 95% sequence identity to Thermus filiformis Hudson et al., which represented only 1.1% of the T0 community. Soil pH controls showed an average change in pH of 0.2±0.2 for each soil sample, suggesting that pH did not significantly fluctuate during enrichment.
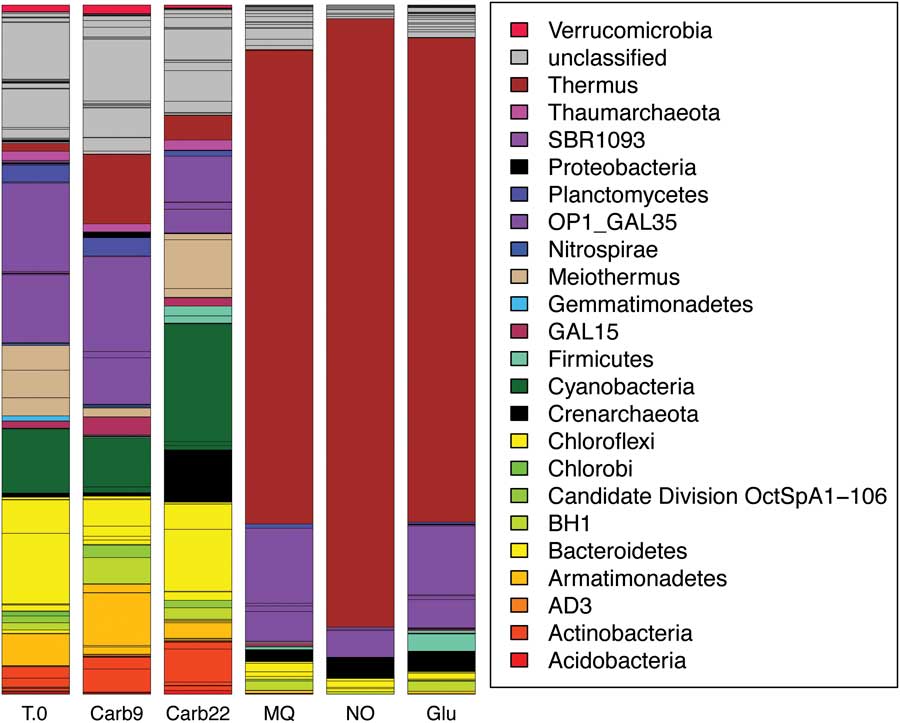
Fig. 3 Community OTU distribution for T0 and enriched communities. The area of each coloured block corresponds to the proportion of the community the OTU comprises.
Discussion
The aim of this study was to identify substrates utilized by the microbial community at Tramway Ridge, and use them to enrich for the dominant members of the microbial community that to date have evaded cultivation. Initially samples were collected for physicochemical and geochemical characterization, and these results were used to guide Biolog and enrichment experiments. Site 3 was selected for Biolog and enrichment experiments because of the common physciochemistry observed at all depths analysed. Subsequently, these depths were pooled for the culture-based analyses to provide sufficient biomass in initial inoculations. Biolog experiments showed microbial respiration was highest under conditions that most closely mimicked the natural environment, namely alkaline and sub-oxic, and many novel carbon and nitrogen compounds were identified as putative substrates for organisms in Tramway Ridge soils. Significant respiration was observed at pH 4.5 and pH 9.5; however, it is probable that the soil based inoculum had a buffering effect on the conditions making it difficult to assess the actual experimental pH of the system. The response of the microbial community to a subset of these substrates in enrichment studies was examined using ARISA, which indicated that most treatments resulted in a similar shift in the community over time. Pyrosequencing confirmed that this shift was a result of decreased richness, decreased evenness and the dominance of a single OTU, similar to Thermus filiformis. Bacterial evenness of the T0 community was 0.52, comparable to that observed in other geothermal environments (Costa et al. Reference Costa, Navarro, Shock, Zhang, Soukup and Hedlund2009), but lower than in mesothermal soils and water columns (Dunbar et al. Reference Dunbar, Takala, Barns, Davis and Kuske1999, Liao et al. Reference Liao, Huang and Huang2007). The response of the community to nitrite/nitrate and bicarbonate enrichments defied this trend. Nitrite/nitrate enrichment accelerated the community shift, suggesting this enrichment has a destabilizing effect on the community. Bicarbonate severely limited the community shift, suggesting this enrichment has a stabilizing effect on the community.
Bicarbonate has well known buffering capacity in acid-base homeostasis (Vanbreem & Wielemak Reference Vanbreem and Wielemak1974, Georgacakis et al. Reference Georgacakis, Sievers and Iannotti1982). Buffer systems are typically composed of a weak acid and its conjugate base, which function to resist pH change by absorbing input of either pH altering hydrogen or hydroxide ions. Because pH appears to play a role in structuring the microbial community at Tramway Ridge (Soo et al. Reference Soo, Wood, Grzymski, McDonald and Cary2009), the presence of bicarbonate in the enrichment experiment may maintain homeostasis by buffering pH. In the absence of this buffering, the pH of the enriched systems may have shifted, thus creating a new niche for organisms with the capacity to thrive in a changed pH environment. This may partly explain why Thermus sp. came to dominate the community in all non-bicarbonate treatments, as Thermus sp. can grow over a pH range of 6 to 8.6 (Hudson et al. Reference Hudson, Morgan and Daniel1987). However, no appreciable change in pH was detected in samples incubated with added water at 65°C, a condition that promoted the shift to a community dominated by Thermus.
An alternate explanation for the observed community shift is that addition of organic substrates favours the growth of heterotrophic Thermus sp. In the natural environment, the subsurface of Tramway Ridge has very low organic carbon levels (Hansom & Gordon Reference Hansom and Gordon1998, Soo et al. Reference Soo, Wood, Grzymski, McDonald and Cary2009). Organic substrates that enhanced respiration in Biolog experiments (N-acetyl glucosamine, chitin, inositol and inulin) failed to support maintenance of the original community composition in enrichment experiments, instead enhancing growth of Thermus sp. which were carbon limited in the environment and took advantage of the carbon-rich conditions of the enrichments. This explanation fits the experimental observations, with the exception of inorganic treatments that showed no retardation in community shift e.g. Milli-Q, sodium sulfate and sulfide, salt, potassium sulfate, ammonium oxalate and nitrite/nitrate, with the latter showing an acceleration in community shift. This suggests that the community shift is not substrate specific, but an effect of the general experimental conditions. It is interesting that nitrite/nitrate treatment accelerated the shift, as some Thermus species have been shown to utilize nitrate as an electron acceptor under anaerobic conditions (Ramírez-Arcos et al. Reference Ramírez-Arcos, Fernández-Herrero, Marín and Berenguer1998). Although this experiment was not strictly anaerobic, the soil was compacted into sealed tubes which may have restricted oxygen supply, and created anoxic zones within the soil. Furthermore, it would explain how a facultative organism could come to dominate the community in the presence of appropriate electron acceptors. Another explanation for accelerated nitrite/nitrate induced community destabilization is the potential toxicity of nitrite to the system.
A tempting hypothesis to consider is that bicarbonate may provide a supply of inorganic substrates for chemoautotrophic members of the community. At Tramway Ridge, the CO2 rich fumeroles, and slightly alkaline pH of the soils (Soo et al. Reference Soo, Wood, Grzymski, McDonald and Cary2009), would act to provide a constant mix of CO2 and bicarbonate to organisms living within fumarolic sediments. The GAL35-like sequence dominating the T0 and bicarbonate communities matched (> 99% nucleotide identity) sequences from Yellowstone National Park (Meyer-Dombard et al. Reference Meyer-Dombard, Swingley, Raymond, Havig, Shock and Summons2011), United States Great Basin (Costa et al. Reference Costa, Navarro, Shock, Zhang, Soukup and Hedlund2009), El Tatio geyser field, Chile (Engel et al. Reference Engel, Johnson and Porter2013), and Rupi Basin, Bulgaria (unpublished data). Although very little is known about the bacterial GAL35 class, the only genomic data available for this phylum points to the presence of an acetogenic Wood-Ljungdahl (acetyl-CoA) pathway for CO2 fixation (Takami et al. Reference Takami, Noguchi, Takaki, Uchiyama, Toyoda, Nishi, Chee, Arai, Nunoura, Itoh, Hattori and Takai2012). Other chemoautotrophs were also present as minor components of the T0 community, and were maintained through bicarbonate treatment, including the nitrifying organisms, Thaumarchaeota group 1.1b and Nitrospirae (Hatzenpichler et al. Reference Hatzenpichler, Lebedeva, Spieck, Stoecker, Richter, Daims and Wagner2008). However, in the fumarole environment at Tramway Ridge the measured organic carbon concentrations in the soils were higher than the total carbon concentrations (although both were very low), suggesting there is no inorganic carbon available. Therefore, it may be possible that in this environment any available inorganic carbon is rapidly turned over by the chemoautotrophic members of the community, thus limiting their growth.
This study has provided new insights into the metabolism of the Tramway Ridge microbial community and putative roles of specific organisms, and how these drive the structure and functioning of the community. Enrichment studies showed that bicarbonate slows down the otherwise dramatic shift in community structure. The buffering capacity of bicarbonate would reasonably explain this observation; however, soil pH did not change during incubation. The dramatic shift observed in all other treatments was not substrate specific and could not be explained by organic substrate loading as inorganic treatments also caused the same shift. However, the original community was dominated by a putative chemoautotroph (OP1-GAL35), and contained other known chemoautotrophs (Thaumarchaeota, Nitrospirae), which were only maintained by the bicarbonate treatment. These results suggest that bicarbonate prevented the community shift by supplying essential inorganic compounds which are utilized for slow, autotrophic growth. Hopefully these new insights will aid in the isolation of the dominant organisms from the soils of Tramway Ridge fumaroles, rather than the continued isolation of minor members of the community.
Acknowledgements
Financial support was provided by grant UOW0802 from the New Zealand Marsden Fund to SCC and IRM, and a CRE award from the National Geographic Society to SCC. Antarctic logistic support for Event K-023 was provided by Antarctica New Zealand. The authors would also like to thank the reviewers in helping to improve the manuscript.
Author contributions
Planning and field sampling by CJV, CWH, SCC and IRM. Data collection by CJV and CWH. Manuscript preparation by CJV, CWH, SCC and IRM.