Introduction
Pathogenic strains of Escherichia coli can cause many different types of diseases in people and animals. In people, they cause diarrhea, hemorrhagic colitis, hemolytic uremic syndrome, urinary tract infections, and neonatal meningitis, among other types of illnesses. In cattle, they produce calf scours and mastitis; in pigs they are the etiologic agents for post-weaning diarrhea and edema disease; and in chickens they cause peritonitis and airsaccullitis. In horses, extraintestinal pathogenic E. coli (ExPEC) cause bronchopneumonia, hemolytic uremic syndrome, and encephalopathy (DebRoy et al., Reference DebRoy, Roberts, Jayarao and Brooks2008; Dickinson et al., Reference Dickinson, Gould, Davidson, Avery, Legare, Hyatt and DebRoy2008). In dogs, cats, and tigers, ExPEC strains have been reported to cause bronchopneumonia (Handt et al., Reference Handt, Stoffregen, Prescott, Pouch, Ngai, Anderson, Gatto, DebRoy, Fairbrother, Motzel and Klein2003; Sura et al., Reference Sura, Van Kruiningen, DebRoy, Hinckley, Greenberg, Gordon and French2007; Carvallo et al., Reference Carvallo, DebRoy, Baeza, Hinckley and Gilbert2010).
The E. coli pathotypes have been classified based on the type of diseases they may cause. The intestinal E. coli, that cause diarrhea are characterized by the virulence attributes they carry, are divided into a number of major groups, including the Shiga toxin-producing E. coli (STEC) of which enterohemorrhagic E. coli (EHEC) are a subset, as well as enterotoxigenic E. coli (ETEC), enteropathogenic E. coli (EPEC), enteroinvasive E. coli (EIEC), and enteroaggregative E. coli (EAggEC) (Kaper et al., Reference Kaper, Nataro and Mobley2004). ExPEC, strains that cause diseases outside the intestinal tract, are associated with neonatal meningitis, urinary tract infection, and septicemia in human beings, as well as pneumonia and urinary tract infection in animals (Russo and Johnson, Reference Russo and Johnson2003; Smith et al., Reference Smith, Fratamico and Gunther2007).
E. coli strains can be distinguished by their O- and H-antigens that are located in the outer membrane of E. coli. Lipopolysaccharides (LPS) that are part of the outer membrane of Gram-negative bacteria are composed of three parts: lipid A, the core region, and the O-antigen polysaccharide that is specific for each O-serogroup and is exposed on the cell surface (Stenutz et al., Reference Stenutz, Weintraub and Widmalm2006; Bos et al., Reference Bos, Robert and Tommassen2007). LPS, as a pathogenic entity, is responsible for stimulating the innate immune system in the host that recognizes a bioactive component known as the Toll-like receptor 4(TLR4), which is present on the surface of cells of the innate immune system (Poltorak et al., Reference Poltorak, He, Smirnova, Liu, Van Huffel, Du, Birdwell, Alejos, Silva, Galanos, Freudenberg, Ricciardi-Castagnoli, Layton and Beutler1998; Akira et al., Reference Akira, Uematsu and Takeuchi2006). O-antigens are known to contribute to virulence as specific clones of some serotypes are associated with certain diseases (Achtman and Pluschke, Reference Achtman and Pluschke1986). Outbreaks of gastrointestinal illness caused by EHEC O157:H7 were first described in 1983 (Riley et al., Reference Riley, Remis, Helgerson, McGee, Wells, Davis, Hebert, Olcott, Johnson, Hargrett, Blake and Cohen1983; Remis et al., Reference Remis, MacDonald, Riley, Puhr, Wells, Davis, Blake and Cohen1984). Among ExPEC strains, O4 and O6 serogroups have been implicated in causing fetal pneumonia in dogs, cats, and tigers (Handt et al., Reference Handt, Stoffregen, Prescott, Pouch, Ngai, Anderson, Gatto, DebRoy, Fairbrother, Motzel and Klein2003; Sura et al., Reference Sura, Van Kruiningen, DebRoy, Hinckley, Greenberg, Gordon and French2007; Carvallo et al., Reference Carvallo, DebRoy, Baeza, Hinckley and Gilbert2010). O-groups provide important pathotype information, and therefore, are considered essential for outbreak investigation, risk management, epidemiological studies, and treatment options.
Serological classification
Kaufmann (Reference Kaufmann1943, Reference Kaufmann1944, Reference Kaufmann1947) classified E. coli into 20 O-groups on the basis of an antigenic scheme that he developed by using boiled cultures of E. coli for antisera production. In this method, antisera are generated against E. coli strains belonging to each O-group by injecting the boiled cultures into rabbits. An agglutination reaction that occurs with one of these antisera with an unknown strain (that is also boiled) represents the O-group of the unknown strain. This serotyping scheme has been used as the ‘gold standard’ for classification of E. coli. Identification of a combination of three surface antigens, O-antigen, H-flagellar antigen, and K-capsular antigen, was initially conceived for subtyping E. coli. However, most laboratories lack capabilities to type K-antigens, and therefore, serotyping based on O- and H-typing has been considered as the standard. In the past 70 years, E. coli strains have been classified into serogroups O1–O187, except for six O-groups that have not been designated (O31, O47, O67, O72, O94, and O122) (Ørskov and Ørskov, Reference Ørskov and Ørskov1984; Scheutz et al., Reference Scheutz, Cheasty, Woodward and Smith2004). Although four O-groups have been sub-grouped previously as O18ab/O18ac, O28ab/O28ac, O112ab/O112ac, and O125ab/O125ac, we recently reported O18ab/O18ac, O112ab/O112ac, and O125ab/O125ac to have identical O-AGCs (DebRoy et al., Reference DebRoy, Fratamico, Yan, Baranzoni, Liu, Needleman, Tebbs, O'Connell, Allred, Swimley, Mwangi, Kapur, Raygoza Garay, Roberts and Katani2016). Eight O groups were previously designated as OX1–OX8 by Ewing and Tatum (Reference Ewing and Tatum1956); however, OX1 is now designated as O170, OX2 as O169, OX3 as O174, OX4 as O146, OX5 as O168, OX6 as O171, and OX7 as O175 (Scheutz et al., Reference Scheutz, Cheasty, Woodward and Smith2004). Eleven other OX groups have been informally used by many laboratories including ours. Some of the OX groups have been found to be 98–99% related to the designated O-groups. These are OX6/O168 (OX6 strains may vary, and we found OX6 to be similar to O168), OX9/O184, OX10/O159, OX19/O11, OX21/O163, OX38/O128, and OX43/O19. OX groups OX13, OX18, OX25, OX28, and OX38 have been found to be unique (DebRoy et al., Reference DebRoy, Fratamico, Yan, Baranzoni, Liu, Needleman, Tebbs, O'Connell, Allred, Swimley, Mwangi, Kapur, Raygoza Garay, Roberts and Katani2016). Currently, the Staten Serum Institut in Denmark (http://www.ssi.dk/English.aspx) has listed serogroups up to O188. Additional O-groups have been since discovered (Iguchi et al., Reference Iguchi, Iyoda, Seto, Nishii, Ohnishi, Mekata, Ogura and Hayashi2016) that have not yet been designated.
Traditional serotyping still remains one of the most comprehensive and simple methods (DebRoy et al., Reference DebRoy, Roberts and Fratamico2011a, Reference DebRoy, Roberts, Valadez, Dudley and Cutterb); however, the method is not reliable as many strains cross-react with other O-group antisera, and results are equivocal if the organism is encapsulated, rough, does not carry LPS, or is autoagglutinating. In our experience of serotyping thousands of E. coli strains, about 25% of the strains cannot be assigned to any O-group and are designated either negative, rough, autoagglutinating, or as belonging to multiple serogroups. Similar observations have also been reported by others (Lacher et al., Reference Lacher, Gangiredla, Jackson, Elkins and Feng2014). The O-antigens are highly immunogenic, and variability among the groups provides the basis for serotyping and classification of E. coli. Carbohydrate analysis methods have been valuable in the identification of the structures of numerous O-antigens (MacLean et al., Reference MacLean, Webb and Perry2006; Chafchaouni-Moussaoui et al., Reference Chafchaouni-Moussaoui, Novikov, Bhrada, Perry, Filali-Maltouf and Caroff2011).
Biosynthesis of O-antigens
The genes within the O-antigen gene clusters (O-AGC) that are responsible for the biosynthesis of O-antigens are located on the chromosome between the two housekeeping genes, galF and gnd, and can be amplified by polymerase chain reaction (PCR) targeting of a 39-bp JUMPstart region upstream of the O-AGC and gnd (Batchelor et al., Reference Batchelor, Alifano, Biffali, Hull and Hull1992; Bastin et al., Reference Bastin, Stevenson, Brown and Reeves1993; Hobbs and Reeves, Reference Hobbs and Reeves1994). O-antigens are composed of repeat units of oligosaccharides (O-unit) that are sugar residues varying considerably in number of units, arrangement, and linkage of the O-units, thus making the O-antigen the most variable region of the bacterial cell. There are three major gene classes that compose the O-AGC: the nucleotide sugar synthesis genes, sugar transferase genes, and O-unit processing genes (Bronner et al., Reference Bronner, Clarke and Whitfield1994; Keenleyside and Whitfield, Reference Keenleyside and Whitfield1996; Daniels et al., Reference Daniels, Vindurampulle and Morona1998; Linton and Higgins, Reference Linton and Higgins1998; Samuel and Reeves, Reference Samuel and Reeves2003; Liu et al., Reference Liu, Knirel, Feng, Perepelov, Senchenkova, Wang, Reeves and Wang2008a; Wang et al., Reference Wang, Ruan, Wei, Hu, Wu, Yu, Feng and Wang2010). Nucleotide sugar synthesis genes encode for proteins that are responsible for sugar synthesis, specific to certain O-groups, and some of these genes are conserved among various O-AGCs. There are four genes, rmlB (dTDP-glucose 4, 6-dehydratase), rmlD (dTDP-4-dehydrorhamnose reductase), rmlA (glucose-1-phosphate thymidylyltransferase), and rmlC (dTDP-4-dehydrorhamnose 3,5-epimerase) that are involved in the biosynthesis of dTDP-L-rhamnose and that exhibit relatedness in different O-groups and often group together in the cluster (DebRoy et al., Reference DebRoy, Fratamico, Yan, Baranzoni, Liu, Needleman, Tebbs, O'Connell, Allred, Swimley, Mwangi, Kapur, Raygoza Garay, Roberts and Katani2016). In 56 of the O-AGCs, the manB gene encoding for phosphomannomutase and manC encoding for mannose-1-phosphate guanyltransferase responsible for the biosynthesis of GDP-D-mannose (Samuel and Reeves, Reference Samuel and Reeves2003) are present. Two other genes involved in biosynthesis of UDP-L-FucNAc derived from UDP-GlcNAc, fnlA (UDP-glucose epimerase) and fnlC (UDP-N-acetylglucosamine 2-epimerase), were identified in 15 O-AGCs. VioA and VioB that carry out transamination of dTDP-6-deoxy-D-xylo-4-hexulose to dTDP-4-amino-4,6-dideoxy-D-glucose (VioN) and VioB that N-acetylates VioN to dTDP-VioNAc were found to be associated with the O-AGC for O39, O49, and O116 (DebRoy et al., Reference DebRoy, Fratamico, Yan, Baranzoni, Liu, Needleman, Tebbs, O'Connell, Allred, Swimley, Mwangi, Kapur, Raygoza Garay, Roberts and Katani2016). Sugar transferase genes encoding for glycosyl transferase proteins, transfer various precursor sugars to form oligosaccharides on the carrier lipid undecaprenyl phosphate (UndP), situated in the inner membrane facing the cytoplasm (Reeves, Reference Reeves, Ghuysen and Hakenbeck1994). Highly specific and varied glycosyl transferases are associated with each of the O-AGCs. The O-antigen processing proteins, Wzx (O-antigen flippase), and Wzy (O-antigen polymerase) are involved in translocation of the O-units across the membrane and in polymerization of the sugar units, respectively. The O-unit is synthesized by sequential transfer of sugars and other constituents to the first sugar, which is then translocated and flipped across the membrane by the protein Wzx to the periplasmic face. These are further polymerized by Wzy (Mulford and Osborn, Reference Mulford and Osborn1983; McGrath and Osborn, Reference McGrath and Osborn1991; Reeves and Wang, Reference Reeves and Wang2002; Liu et al., Reference Liu, Knirel, Feng, Perepelov, Senchenkova, Wang, Reeves and Wang2008a). The number of O-units in the final O-antigen is regulated by Wzz. The Wzx and Wzy proteins are both hydrophobic and have about 12 predicted transmembrane regions, and a long cytoplasmic region is predicted between the transmembrane domains in Wzy. O-groups O8, O9, O52, O60, O89, O92, O95, O97, O99, O101, and O162 are ABC transporter dependent for O-antigen processing and carry wzt and wzm genes (DebRoy et al., Reference DebRoy, Fratamico, Yan, Baranzoni, Liu, Needleman, Tebbs, O'Connell, Allred, Swimley, Mwangi, Kapur, Raygoza Garay, Roberts and Katani2016). The transportation processing is brought about by the ABC transporter protein, Wzm, which is responsible for export, and Wzt is the ATP-binding component.
Relatedness among O-AGCs
The nucleotide sequences of all designated 187 O-AGCs have been recently reported (Iguchi et al., Reference Iguchi, Iyoda, Kikuchi, Ogura, Katsura, Ohnishi, Hayashi and Thomson2015a; DebRoy et al., Reference DebRoy, Fratamico, Yan, Baranzoni, Liu, Needleman, Tebbs, O'Connell, Allred, Swimley, Mwangi, Kapur, Raygoza Garay, Roberts and Katani2016). Out of these O-groups, 13 sets exhibited 98–99.9% identical nucleotide sequences. These are O2/O50, O13/O129/O135, O17/O44/O73/O77/O106, O18ab/O18ac, O42/O28ac, O46/O134, O62/O68, O90/O127, O101/O162, O107/O117, O118/O151, O123/O186, O124/O164, and O125ab/O125ac (Table 1) (DebRoy et al., Reference DebRoy, Fratamico, Yan, Baranzoni, Liu, Needleman, Tebbs, O'Connell, Allred, Swimley, Mwangi, Kapur, Raygoza Garay, Roberts and Katani2016). O-groups that carry identical nucleotide sequences may or may not cross-react in serological reactions. There were three nucleotide differences between the O-AGCs of O118 and O151, and there was an insertion sequence in O62 located between the rmlA and rmlC genes, but otherwise, the O-AGCs of O62 and O68 were similar. However, these differences may be responsible for the absence of serological cross-reactions with these serogroups (Liu et al., Reference Liu, Fratamico, DebRoy, Bumbaugh and Allen2008b, Reference Liu, Yan, DebRoy, Fratamico, Needleman, Li, Wang, Losada, Brinka, Radune, Toro, Hegde and Meng2015). There are other O-groups that have identical nucleotide sequences that do not exhibit any serological cross-reactions. Further studies on the expression of genes of the O-antigens may shed light on production of the O-antigen and the immune response to these antigens.
Table 1. Escherichia coli O-AGCs that are 98–99% identical in nucleotide sequence
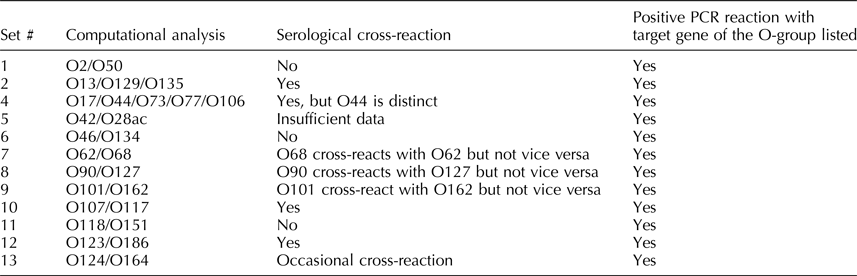
Molecular genotyping of O-groups
Molecular serogrouping of O-antigens entails determining the O-group by molecular methods. Several molecular methods have been used to distinguish different O-groups, including restriction fragment length polymorphism (RFLP) (Coimbra, et al., Reference Coimbra, Grimont, Lenormand, Burguière, Beutin and Grimont2000; Beutin et al., Reference Beutin, Strauch, Zimmermann, Kaulfuss, Schaudinn, Männel and Gelderblom2005a, Reference Beutin, Kong, Feng, Wang, Krause, Leomil, Jin and Wangb; Moreno et al., Reference Moreno, Guth and Martinez2006; Abbadi and Strockbine, Reference Abbadi and Strockbine2007), high throughput real-time PCR (Bugarel et al., Reference Bugarel, Beutin, Martin, Gill and Fach2010; Delannoy et al., Reference Delannoy, Beutin and Fach2013; Tseng et al., Reference Tseng, Fratamico, Bagi, Delannoy, Fach, Manning and Funk2014), multiplex-PCR (Fratamico and DebRoy, Reference Fratamico and DebRoy2010; Botkin et al., Reference Botkin, Galli, Sankarapani, Soler, Rivas and Torres2012; Doumith et al., Reference Doumith, Day, Hope, Wain and Woodford2012; Fratamico et al., Reference Fratamico, Wasilenko, Garman, DeMarco, Varkey, Jensen, Rhoden and Tice2014; Iguchi et al., Reference Iguchi, Iyoda, Seto, Morita-Ishihara, Sheutz, Ohnishi and Pathogenic2015b), microarray (Hegde et al., Reference Hegde, Praul, Gehring, Fratamico and DebRoy2013; Lacher et al., Reference Lacher, Gangiredla, Jackson, Elkins and Feng2014; Patel et al., Reference Patel, Gangiredla, Lacher, Mammel, Jackson, Lampel and Elkins2016), flow cytometry (Liu and Fratamico, Reference Liu and Fratamico2006; Hegde et al., Reference Hegde, Jayarao and DebRoy2012; NeoSEEK™ (PCR-Mass spectroscopy) (Stromberg et al., Reference Stromberg, Baumann, Lewis, Sevart, Cernicchiaro, Renter, Marx, Phebus and Moxley2015), and targeted amplicon sequencing for single-nucleotide-polymorphism genotyping (Ison et al., Reference Ison, Delannoy, Bugarel, Nagaraja, Renter, den Bakker, Nightingale, Fach and Loneragan2016). Most of these methods have been applied to distinguish pathotypes belonging to certain O-groups. A multiplex PCR method using 162 PCR primer pairs for 141 O-groups was developed by Iguchi et al. (Reference Iguchi, Iyoda, Kikuchi, Ogura, Katsura, Ohnishi, Hayashi and Thomson2015a, Reference Iguchi, Iyoda, Seto, Morita-Ishihara, Sheutz, Ohnishi and Pathogenicb). Although currently a whole genome sequencing (WGS) approach to determine O-groups is trending (Norman et al., Reference Norman, Clawson, Strockbine, Mandrell, Johnson, Ziebell, Zhao, Fratamico, Stones, Allard and Bono2015), we have developed PCR for each of the O-groups for laboratories that are still not ready for WGS because of cost or lack of analysis tools.
Target genes for detection of O-groups
The O-antigen gene clusters are generally highly heterogeneous with considerable nucleotide differences among the clusters. However, the predominant genes in the clusters are those involved in nucleotide sugar synthesis, and encoding for synthetases, transferases, epimerases, and other enzymes. Although the genes encoding specific proteins may have similarities in functions among the O-groups, the DNA sequences for some may be quite variable. In most of the clusters, two genes, encoding the O-antigen flippase (wzx) and the O-antigen polymerase (wzy) responsible for O-antigen translocation across the membrane and polymerization, are relatively unique, and therefore have been used as targets in genetic-based methods for serogroup identification. For developing PCR tests for detecting specific O-groups, primers targeting unique genes (usually wzx and wzy) in the O-AGCs were designed. In 11 O-AGCs, O8, O9, O52, O60, O89, O92, O95, O97, O99, O101, and O162, are ATP-binding Cassette (ABC) transporter integral membrane proteins that are dependent on wzt and wzm genes that assist in the O-antigen processing and transportation process. The O-AGCs for O14 and O57 could not be mapped (Iguchi et al., Reference Iguchi, Iyoda, Kikuchi, Ogura, Katsura, Ohnishi, Hayashi and Thomson2015a, Reference Iguchi, Iyoda, Seto, Morita-Ishihara, Sheutz, Ohnishi and Pathogenicb; DebRoy et al., Reference DebRoy, Fratamico, Yan, Baranzoni, Liu, Needleman, Tebbs, O'Connell, Allred, Swimley, Mwangi, Kapur, Raygoza Garay, Roberts and Katani2016). O14 strains are rough and cannot be serotyped (Ørskov et al., Reference Ørskov, Ørskov, Jann and Jann1977), and an O-AGC could not be identified in O57. Therefore, primers for these two O-groups were not designed. We have put together all the primers that have been developed by our group and others, and provided a practical method for detecting the O-serogroup of E. coli with PCR (Table 2).
Table 2. Primer sequences for the detection of O-groups by PCR

pos, positive by PCR; bp, base pairs.
PCR primers, methodology, and specificity
E. coli strains belonging to the targeted serogroup were grown overnight on tryptic soy agar (TSA) plates at 37°C. A single colony was picked and resuspended in 150 µl of distilled water and heated at 100°C for 10 min. The suspension was centrifuged at 12,000 ×g, and the supernatant containing genomic DNA was used for the PCR reaction. Primers were designed for the genes listed in Table 2 for each serogroup. They were synthesized from Integrated DNA Technologies, (Coralville, IA) and reconstituted to 100 µM concentration using nuclease-free water from the manufacturer. PCR was conducted in 96-well microtiter plates. Each well contained 300 nM of primer pairs and Denville ChoiceTaq Blue working mix (22 µl) (Denville Scientific, MA, USA). Template DNA (3 µl) was added to the mix, and the PCR was carried out using an Eppendorf Mastercycler Pro (Eppendorf, Germany) consisting of initial denaturation at 94°C for 2 min, followed by 25 cycles of 94°C for 10 s and using annealing temperatures listed in Table 2 for each O-group, followed by extension at 72°C for 15 s and the final extension at 72°C for 1 min. Reaction mixtures without template DNA and without primers served as negative controls. The amplification products were subjected to electrophoresis in 1% agarose gels at 200 V for 1 h. The gels were stained with ethidium bromide, and PCR products were visualized under UV light. Positive samples were identified based on the presence of bands of the expected sizes and compared with results using DNA from control reference strains belonging to the targeted serogroup.
PCR assays were developed using the reference O-standard strains, routinely used for serotyping, that were obtained from the World Health Organization Collaborating Center for Reference and Research on Escherichia and Klebsiella based at the Statens Serum Institut. The reference strains belong to serogroups O1 to O187 except for O31, O47, O67, O72, O94, and O122 serogroups that are not designated (Ørskov et al., Reference Ørskov, Ørskov, Jann and Jann1977). The specificity of the PCR assays for each serogroup was tested by using field strains or clinical isolates (see Table 2) belonging to the targeted serogroup and obtained from the E. coli collection at the E. coli Reference Center. The percentage of the strains that exhibited positive results by PCR was noted, and the ones that exhibited negative results were re-serotyped to make sure that the serogroup was correctly listed in the database. In addition, randomly selected field strains (n = 50) belonging to serogroups other than the targeted serogroup and 21 other bacterial species, including Citrobacter freundii, Enterobacter cloacae, Enterococcus aerogenes, E. faecalis, Hafnia alvei, Klebsiella pneumoniae, Lactococcus lactis, Listeria monocytogenes, Proteus vulgaris, Pseudomonas aeruginosa, Salmonella enterica subsp. Anatum, Arizona, Choleraesuis, and Typhimurium, Serratia marcescens, Shigella boydii, Shigella flexneri, Staphylococus aureus, Streptococcus pyrogenes, and Yersinia enterocolitica were tested for specificity.
Detection of the O-antigen for all serogroups
PCR assays for most of the O-groups were between 80 and 100% specific for the targeted O-type. Although primers designed for O19, O20, and O97 exhibited positive results in PCR for the reference strains, reflecting that the PCR is designed correctly, the specificities among the clinical isolates belonging to these O-groups were very low. All the clinical isolates or field strains that exhibited negative results by PCR were re-serotyped and found to belong to the targeted O-group sometimes, and sometimes they exhibited a different O-group. The serotyping results, therefore, may be flawed. For example, we tested 44 isolates that were all serotyped as O20; however, none of them were positive by PCR targeting wzx or wzy (GenBank no. 778793.1). Sequencing O-AGCs of 44 O20 strains showed that they belonged to other serogroups such as O8, O48, O86, O111, O118, O131, O160, O178, and O-non-typeable (Dr D. W. Lacher, personal communication). Therefore, O20 antigens are not always specific for the O20 genotype. Although Iguchi et al. (Reference Iguchi, Iyoda, Kikuchi, Ogura, Katsura, Ohnishi, Hayashi and Thomson2015a, Reference Iguchi, Iyoda, Seto, Morita-Ishihara, Sheutz, Ohnishi and Pathogenicb) reported primer sequences for detecting O20, these primers also did not show any positive results for O20 clinical isolates. Other O-groups that exhibited relatively low specificity among the field strains were O32 (62%), O34 (53%), O64 (21%), O93 (30%), and O152 (58%). The percentage of strains that exhibit a positive response by PCR may improve in these O-groups if more clinical/field isolates are tested, because the serotyping results for these O-groups are not always accurate. Thus, when we compare classical serotyping to molecular serotyping results, disparities are observed. Clinical isolates of certain O-groups, may not exhibit positive results with PCR of the targeted O-group. Further investigation as to why they are not specific for the O-type designated by serotyping needs to be determined for each individual isolate. This can be due to mutations, insertions, or deletions in the targeted gene, which can be further analyzed to determine the actual O-group. On the other hand, serotyping may not be always accurate, and strains that showed negative results by PCR may actually belong to other serogroups. It has been reported (Plainvert et al., Reference Plainvert, Bidet, Peigne, Barbe, Médigue, Denamur, Bingen and Bonacorsi2007) that a pathogenic clone of E. coli that causes meningitis belonging to serogroup O45 has two O45 polysaccharides, representing two different antigens. The O-AGC for this E. coli O45 clone is genetically related to the O45 reference strain, although they show low DNA sequence homology of the orthologous genes. Both the meningitis strain and the reference strain react to O45 antisera serologically.
When bacteria other than E. coli were used for testing specificity, most of the PCR assays for O-groups were highly specific for E. coli except for O13 strains, in which PCR assays targeting the O13 wzx gene reacted positively with S. flexneri. O147 strains with PCR assays targeting the O147 wzx gene exhibited positive results with S. boydii. It is known that the O-antigens of E. coli and Shigella species are structurally closely related (Liu et al., Reference Liu, Wu, Li, Beutin, Chen, Cao and Wang2010; Knirel et al., Reference Knirel, Qian, Shashkov, Sizova, Zdorovenko, Naumenko, Senchenkova, Perepelov and Liu2016). The O-antigens of O13, O129, and O135 are almost identical and the structure is shared by the S. flexneri O-antigen group. While S. flexneri O-antigen F2a reacts with E. coli O13 serologically, F4b and F5 react with O135 and O129, respectively (Ewing, Reference Ewing, Edwards and Ewing1986; Morona et al., Reference Morona, Van Den Bosch and Manning1995). Shigella O-antigens, F1–5 have close structural similarity and have the same basic O-unit structure, and the distinguishing features are due to phage-encoded post-polymerization modifications in O acetylation and/or glucosylation (Liu et al., Reference Liu, Knirel, Feng, Perepelov, Senchenkova, Wang, Reeves and Wang2008a).
Future directions
While serotyping has been a reliable and low-cost method for determining the E. coli O-groups, it has many disadvantages. It is now imperative that a platform be developed for detecting O-types based on molecular methods that are fast, reliable, and cost-effective. Although the PCR is a comparatively easy and low-cost method, developing other formats such as microarray, for high throughput processing could substantially reduce the labor and time required. WGS is now being considered by many public health laboratories for routine diagnostics as the cost of DNA sequencing is declining (Joensen et al., Reference Joensen, Tetzschner, Iguchi, Aarestrup and Scheutz2015; Kwong et al., Reference Kwong, McCallum, Sintchenko and Howden2015). Joensen et al. (Reference Joensen, Scheutz, Lund, Hasman, Kaas, Nielsen and Aarestrup2014) developed the web tool known as SerotypeFinder for identifying E. coli serotypes by analysis of the WGS data. Another method developed by Ingle et al. (Reference Ingle, Valcanis, Kuzevski, Tauschek, Inouye, Stinear, Levine, Robins-Browne and Holt2016) was used to rapidly and accurately detect serotypes of E. coli directly from WGS reads using short read data. Their approach bypasses the need for de novo assembly of the genome by directly screening short read data against the curated database of alleles linked to known E. coli O- and H-groups (the EcOH database), using the software package SRST2. Applying this approach, they explored the genetic diversity of >1500 E. coli genomes from multiple sources and found serotype diversity, revealing 38 novel O-AGCs. The O-antigen region is subjected to diversity due to selection pressure from the host immune system, as well as due to insertion of phages. Therefore, recombination events may occur in O-AGCs, resulting in O-antigens from related strains having different O-types and from different lineages having similar O-types. As more WGS data are becoming available, diversity in the O-AGC region will be realized. As different approaches for typing E. coli serogroups are explored, it is hoped that in the near future, an easy, rapid and reliable molecular-based method based on the sequences of the O-AGCs will become available for routine testing of E. coli serogroups.