Staphylococci and foodborne illness
Staphylococcus aureus is a food-borne pathogen that produces heat-stable enterotoxins during growth in a variety of foods including meat and poultry products, eggs, cream-filled pastries, potatoes, and some salads. Staphylococcal enterotoxins are estimated to cause food-borne illness in about 241,000 persons in the U.S. annually. Staphylococcal food poisoning is believed to be greatly underreported and underdiagnosed because of the short duration of illness and infrequent complications (Scallan et al., Reference Scallan, Hoekstra, Angulo, Tauxe, Widdowson, Roy, Jones and Griffin2011). Data from Centers for Disease Control and Prevention (CDC) for 1998 to 2010 indicate that the annual number of reported outbreaks peaked at 82 in 2002 and declined to 11 in 2010. Nearly half of the 573 reported outbreaks occurring in 1998–2010 were associated with some type of meat (Table 1). Seafood, potatoes/rice/noodles, vegetables/salads, combination foods, and dairy products were also cited as food vehicles. Approximately 51% of reported outbreaks involved only 2–4 cases while only 7.3% of outbreaks involved more than 50 cases. Table 2 lists some large outbreaks occurring during this period in the U.S. and other countries.
Table 1. Reported food vehicles for 573 outbreaks of staphylococcal food poisoning reported by CDC for 1998–2010 (http://wwwn.cdc.gov/foodborneoutbreaks/)

* Total of outbreak numbers is >573 (and total of percentages is >100) because more than one food was implicated in some outbreaks.
Table 2. Large outbreaks of staphylococcal food intoxication (1998–2008)
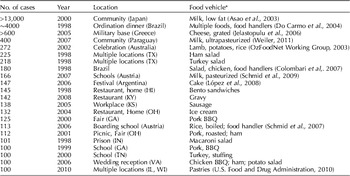
U.S. outbreak information from CDC: http://wwwn.cdc.gov/foodborneoutbreaks/
Data from Alberta, Canada indicate that 10.5% of food samples collected from food-borne disease outbreaks in 2007–2010 contained S. aureus. Of the positive samples, about 65% were meat or prepared foods containing meat, 15% were prepared foods not containing meat, about 14% were dairy products and 4% were produce items (Crago et al., Reference Crago, Ferrato, Drews, Svenson, Tyrrell and Louie2012).
S. aureus has been a food safety concern for meat producers and food processors for decades because it is widespread in the environment and often detected in air, dust, water, raw milk, other foods, and on environmental surfaces. Three recent surveys of retail meat in the U.S detected S. aureus in a significant number of samples of pork, chicken, turkey, and beef, ranging from 12% to 65% of packages. Cell numbers per gram of meat were low, but can increase rapidly if meats are not kept refrigerated (Bhargava et al., Reference Bhargava, Wang, Donabedian, Zervos, da Rocha and Zhang2011; Kelman et al., Reference Kelman, Soong, Dupuy, Shafer, Richbourg, Johnson, Brown, Kestle, Li, Zheng, McDermott and Meng2011; O'Brien et al., Reference O'Brien, Hanson, Farina, Wu, Simmering, Wardyn, Forshey, Kulick, Wallinga and Smith2012). S. aureus cells survive desiccation and tolerate high levels of salt. Although these bacteria are destroyed by heat, enterotoxins produced in a food will survive many preservation processes, including pasteurization and approved doses of irradiation (Rose et al., Reference Rose, Modi, Tranter, Bailey, Stringer and Hambleton1988; Genigeorgis, Reference Genigeorgis1989).
Numerous staphylococcal enterotoxins have been described and it is ingestion of these enterotoxins and not of S. aureus cells that causes onset of nausea and vomiting within 1–6 h. Less than 200 ng of toxin is sufficient to cause symptoms (Evenson et al., Reference Evenson, Hinds, Bernstein and Bergdoll1988). Although symptoms may be severe, they usually resolve within a day and serious complications, hospitalization, and death are rare, afflicting primarily the very young, the elderly, the chronically ill, and those who have consumed a large amount of contaminated food.
S. aureus has also been a problem for caterers and others involved in food preparation. According to several studies, S. aureus is present in nasal passages or skin of about 50% of people and in intestines of about 20% of people in the general population (Acton et al., Reference Acton, Plat-Sinnige, Van Wamel, De Groot and Van Belkum2009; Frank et al., Reference Frank, Feazel, Bessesen, Price, Janoff and Pace2010). Thus, asymptomatic food handlers may harbor S. aureus and can contaminate food during preparation (Todd et al., Reference Todd, Greig, Bartleson and Michaels2008). If contaminated foods, for example salads or some desserts at a picnic, are left at ambient temperature for extended periods, S. aureus may multiply and produce enterotoxins.
In some circumstances, ingestion of staphylococcal cells causes enteritis. Occasionally infants, immunocompromised adults and others treated with large doses of antibiotics develop staphylococcal enterocolitis. When the normal human intestinal microbiota is depleted or absent, ingested S. aureus cells may grow in the intestines and produce enterotoxins that cause profuse diarrhea (Lin et al., Reference Lin, Kotler, Schlievert and Sordillo2010).
Although S. aureus is the most common cause of food-borne staphylococcal intoxication, other species of staphylococci carry genes for enterotoxin production and can potentially cause food-borne illness (Becker et al., Reference Becker, Keller, Von Eiff, Brück, Lubritz, Etienne and Peters2001). One food-borne outbreak in southwestern U.S. in 1991 affecting over 265 people was traced to Staphylococcus intermedius producing type A enterotoxin in a butter blend (Khambaty et al., Reference Khambaty, Bennett and Shah1994).
Non-food-borne illness
Staphylococcus aureus
S. aureus causes a variety of mild to severe skin and soft tissue infections and numerous serious infections including endocarditis, endophthalmitis, osteomyelitis, meningitis, bacteremia, pneumonia, and toxic shock syndrome in humans. These bacteria produce many virulence factors (besides enterotoxins) such as exfoliative toxins, toxic shock syndrome toxin, and immunomodulators. The Panton–Valentine leukocidin (PVL) is a toxin often associated with abscess formation and severe necrotizing pneumonia (Watkins et al., Reference Watkins, David and Salata2012). Asymptomatic carriage of S. aureus by healthy individuals is a risk factor for developing serious staphylococcal infections (Safdar and Bradley, Reference Safdar and Bradley2008).
S. aureus has been isolated from many domestic and wild mammals and from wild and domestic birds and some reptiles (Weese and Van Duijkeren, Reference Weese and Van Duijkeren2010; Ho et al., Reference Ho, Chow, Lai, Law, Chan, Ho, Ng and Yam2012; Wardyn et al., Reference Wardyn, Kauffman and Smith2012). Animals may be asymptomatic carriers or may suffer respiratory, gastrointestinal, or skin and soft tissue infections. S. aureus is a significant cause of mastitis in cows and small ruminants (Vanderhaeghen et al., Reference Vanderhaeghen, Hermans, Haesebrouck and Butaye2010b; Foster, Reference Foster2012). Whether animals can be persistent carriers of S. aureus has yet to be determined. However, healthy animals can intermittently carry S. aureus. A recent study found that 10% of dogs visiting a clinic for regular vaccinations harbored S. aureus (Rubin and Chirino-Trejo, Reference Rubin and Chirino-Trejo2010). Molecular analyses of isolates from different animals have revealed that there are some strains that appear to be host-adapted to a particular animal species and other strains can colonize many species of animals (Cuny et al., Reference Cuny, Friedrich, Kozytska, Layer, Nübel, Ohlsen, Strommenger, Walther, Wieler and Witte2010). S. aureus can be transferred in both directions between humans and animals and frequently infections in companion animals can be traced back to their human caretakers (Rutland et al., Reference Rutland, Weese, Bolin, Au and Malani2009). Farmers also often harbor some S. aureus strains that are similar to those detected in their livestock (Khanna et al., Reference Khanna, Friendship, Dewey and Weese2008).
Other pathogenic staphylococci
Coagulase-positive staphylococci, other than S. aureus, also cause infections in humans and animals. Some animal isolates of coagulase-positive staphylococci are classified in the S. intermedius group (SIG). S. intermedius was originally described in 1976 and is part of the normal microflora of the skin and mucosal membranes of dogs and cats. It has also been detected in a variety of other animals, including horses, mink, goats, foxes, raccoons, and pigeons, but is not commonly present in humans. Recent molecular analyses demonstrated that isolates of S. intermedius detected in a large number of various animals living in several geographic locations have some significant differences and the species can best be reclassified into three clusters: S. intermedius, Staphylococcus pseudintermedius, and Staphylococcus delphini A and B. These three species constitute the SIG (Sasaki et al., Reference Sasaki, Kikuchi, Tanaka, Takahashi, Kamata and Hiramatsu2007; Sledge et al., Reference Sledge, Danieu, Bolin, Bolin, Lim, Anderson and Kiupel2010). Extensive differences were observed in transposons and other accessory genetic elements among different SIG species that may reflect adaptations to different hosts (Ben Zakour et al., Reference Ben Zakour, Beatson, Van Den Broek, Thoday and Fitzgerald2012).
S. pseudintermedius is the most frequently encountered pathogen in the SIG and its taxonomy, epidemiology and pathogenicity were recently reviewed (Bannoehr and Guardabassi, Reference Bannoehr and Guardabassi2012). This species was first identified as distinct from other SIG in 2005 by examination of rRNA gene sequences in clinical staphylococcal isolates from several animals (Devriese et al., Reference Devriese, Vancanneyt, Baele, Vaneechoutte, De Graef, Snauwaert, Cleenwerck, Dawyndt, Swings, Decostere and Haesebrouck2005). The majority of isolates from dogs are now classified as S. pseudintermedius and some surveys report that S. pseudintermedius is present in the nares, pharynx and/or rectum of more than 80% of healthy dogs (Rubin and Chirino-Trejo, Reference Rubin and Chirino-Trejo2011). S. delphini was originally isolated from a dolphin and has been detected in horses. All SIG isolates from 118 mink, 6 badgers, and 1 ferret were recently identified as S. delphini, whereas all except one SIG isolate from 33 foxes were S. pseudintermedius (Guardabassi et al., Reference Guardabassi, Schmidt, Petersen, Espinosa-Gongora, Moodley, Agersø and Olsen2012).
SIG pathogens produce a number of virulence factors (coagulase, hemolysins and exfoliative toxin) similar to those associated with S. aureus (Fitzgerald, Reference Fitzgerald2009). When animals are injured, sick, or otherwise weakened, these bacteria may cause skin, ear, and wound infections (Weese and Van Duijkeren, Reference Weese and Van Duijkeren2010). S. pseudintermedius has been isolated from pet owners and veterinarians (Morris et al., Reference Morris, Boston, O'Shea and Rankin2010) and occasionally causes soft tissue or respiratory infections in humans exposed to dogs carrying these bacteria (Chuang et al., Reference Chuang, Yang, Hsueh and Lee2010). Invasive infections have occurred in a few persons, including some who were bitten by dogs (Hatch et al., Reference Hatch, Sree, Tirrell, Torres and Rothman2012).
Methicillin resistance in staphylococci
Emergence and spread of methicillin resistance
Staphylococci are notorious for rapidly becoming resistant to many antibiotics. Penicillins and other β-lactam antibiotics kill bacterial cells by interfering with cell wall synthesis. Not long after penicillin was first used to treat human infections, S. aureus strains producing penicillinase were detected and it is estimated that now >80% of S. aureus cells produce penicillinase. Methicillin (meticillin), a β-lactam antibiotic that is not inactivated by penicillinase, was introduced in the late 1950s. But by 1961, there were reports of methicillin-resistant staphylococci in a hospital in the United Kingdom (Jevons, Reference Jevons1961). Methicillin-resistant S. aureus (MRSA) are now resistant to all currently available β-lactam antibiotics, including penicillins, cephalosporins, carbapenems, and their derivatives. MRSA have spread worldwide and are currently the most commonly identified antibiotic resistant bacteria in hospitals in Europe, the Americas, North Africa, and the Middle- and Far-East (European Centre for Disease Prevention and Control, 2011). Approximately 478,000 hospitalizations in the U.S. in 2005 were associated with S. aureus infections and 58% of those were caused by MRSA (Klein et al., Reference Klein, Smith and Laxminarayan2007). In 2010, it was estimated that MRSA caused illness in more than 150,000 persons annually in health care facilities in the European Union (Köck et al., Reference Köck, Becker, Cookson, van Gemert-Pijnen, Harbarth, Kluytmans, Mielke, Peters, Skov, Struelens, Tacconelli, Navarro Torné, Witte and Friedrich2010). Recent statistics indicate that severe, invasive, health-care associated MRSA infections have declined, but MRSA infections acquired in the community are still increasing (Kallen et al., Reference Kallen, Mu, Bulens, Reingold, Petit, Gershman, Ray, Harrison, Lynfield, Dumyati, Townes, Schaffner, Patel and Fridkin2010; Hadler et al., Reference Hadler, Petit, Mandour and Cartter2012).
Methicillin resistance in canine S. intermedius isolates was first reported in the mid-late 1990s (Gortel et al., Reference Gortel, Campbell, Kakoma, Whittem, Schaeffer and Weisiger1999). Early isolates of methicillin-resistant S. intermedius from companion animals were probably strains of methicillin-resistant S. pseudintermedius (MRSP) based on the recent changes to SIG taxonomy (Sasaki et al., Reference Sasaki, Kikuchi, Tanaka, Takahashi, Kamata and Hiramatsu2007). For several years, these strains appeared to constitute a small proportion of S. intermedius isolates from animals and, although they exhibited some resistance to other drugs, there were other antibiotics effective against them (Van Duijkeren et al., Reference Van Duijkeren, Catry, Greko, Moreno, Pomba, Pyörälä, Ružauskas, Sanders, Threlfall, Torren-Edo and Törneke2011a). Starting in 2006, there were more frequent reports of MRSP and methicillin-resistant SIG (MRSIG) strains that were resistant to multiple classes of antibiotics, in addition to the β-lactam group (Loeffler et al., Reference Loeffler, Linek, Moodley, Guardabassi, Sung, Winkler, Weiss and Lloyd2007; Van Duijkeren et al., Reference Van Duijkeren, Catry, Greko, Moreno, Pomba, Pyörälä, Ružauskas, Sanders, Threlfall, Torren-Edo and Törneke2011a; Proietti et al., Reference Proietti, Bietta, Coletti, Marenzoni, Scorza and Passamonti2012). Colonization of dogs with MRSP may persist after symptoms of pyoderma have resolved (Beck et al., Reference Beck, Waisglass, Dick and Weese2012).
Methicillin resistance has also been detected in other staphylococcal species, including several species isolated from pigs in Belgium (Vanderhaeghen et al., Reference Vanderhaeghen, Vandendriessche, Crombé, Dispas, Denis, Hermans, Haesebrouck and Butaye2012), Staphylococcus lentus in chickens (Koller et al., Reference Koller, Huber, Cernela, Stephen and Zweifel2011), and several coagulase negative species isolated from livestock, chickens, raw milk and meat in Switzerland (Huber et al., Reference Huber, Ziegler, Pfluger, Vogel, Zweifel and Stephan2011).
Genetic basis of resistance
Resistance to methicillin is mediated by the mecA gene that encodes an altered penicillin-binding protein that is located in the cell wall and that has a low affinity for β-lactam antibiotics. Since β-lactam antibiotics interfere with bacterial cell wall synthesis, this decreased antibiotic binding causes cells to be resistant to the effects of methicillin. The mecA gene resides on a large heterogeneous mobile genetic element called the staphylococcal cassette chromosome (SCCmec) (Ito et al., Reference Ito, Katayama and Hiramatsu1999; Katayama et al., Reference Katayama, Ito and Hiramatsu2000).
To date, 11 major SCCmec variations have been described but types I–V are the most common. SCCmec types I–III are relatively large and are typically found in strains associated with healthcare facilities. SCCmec types IV and V are smaller and are usually found in MRSA associated with community-acquired infections. Molecular analyses of numerous MRSA strains indicate that resistance genes have been transferred to various methicillin-susceptible S. aureus (MSSA) strains on multiple occasions (Robinson and Enright, Reference Robinson and Enright2004). These resistance genes have also been transferred to other staphylococcal species. Many MRSA strains, particularly hospital-associated (HA) strains, are also resistant to other classes of antibiotics, which makes it a challenge to treat serious infections. MRSA continues to evolve and present new challenges for treatment and identification (Sabat et al., Reference Sabat, Koksal, Akkerboom, Monecke, Kriegeskorte, Hendrix, Ehricht, Koeck, Becker and Friedrich2012).
Genes coding for resistance to antibiotics may be present on mobile genetic elements (such as transposons), located on bacterial chromosomes, or on extrachromosomal plasmid DNA. In some species, plasmid gene transfer can occur directly from cell to cell by conjugation. In other cases, genes on mobile elements can be transferred by transduction to other bacterial cells of the same or related species with the aid of viruses. Efficient transduction of resistance genes has been demonstrated in MRSA (Varga et al., Reference Varga, Kuntová, Pantucek, Mašlanová, Ružicková and Doškar2012).
Characterization of MRSA and MRSIG strains
Terms used to designate different MRSA strains are sometimes inconsistent or confusing. Many isolates and clones were originally named according to the geographical areas of origin. In 2002, a proposal was made to identify isolates according to sequence type (ST), antibiotic resistance, and SCCmec type. ST is determined by multilocus sequence typing (MLST) of seven housekeeping genes in an isolate and comparing these with known sequences published on the MLST website (http://saureus.mlst.net). As of October 2012, this site contained data on 4588 isolates, representing 2315 STs. Antibiotic resistance is designated as MRSA or MSSA and the SCCmec type as I to VII. For example the New York/Japan clone is ST5-MRSA-II and USA300 is ST8-MRSA-IV. However, many publications continue to refer to well-known strains by their old names. STs that differ in only a few of the genetic loci tested are grouped into clonal complexes (CCs) using BURST (based on related STs) analysis. The ST number of the isolate considered to be closest to the ancestral sequence is assigned as the CC number for that group of highly related STs. Five major CCs originated in hospitals (Robinson and Enright, Reference Robinson and Enright2004). Other CCs developed from S. aureus strains circulating in the community, outside of healthcare facilities (David and Daum, Reference David and Daum2010). CC398 is a CC that appeared to originate in swine (Leonard and Markey, Reference Leonard and Markey2008; Cuny et al., Reference Cuny, Friedrich, Kozytska, Layer, Nübel, Ohlsen, Strommenger, Walther, Wieler and Witte2010).
S. pseudintermedius ST71 has become established as the most common multidrug resistant strain in Europe, whereas ST68 is most commonly detected in North America. Another clonal group, ST106, was identified as the most important MRSP in Norway (Osland et al., Reference Osland, Vestby, Fanuelsen, Slettemeas and Sunde2012). Nearly all strains are resistant to nine classes of important veterinary antimicrobials. Over 70% of MRSP isolates contain the SCCmec elements II or III, and the remaining strains have SCCmec elements III, IV, V, or VII (Perreten et al., Reference Perreten, Kadlec, Schwarz, Andersson, Finn, Greko, Moodley, Kania, Frank, Bemis, Franco, Iurescia, Battisti, Duim, Wagenaar, Van Duijkeren, Weese, Fitzgerald, Rossano and Guardabassi2010; Ruscher et al., Reference Ruscher, Lübke-Becker, Semmler, Wleklinski, Paasch, Šoba, Stamm, Kopp, Wieler and Walther2010; Black et al., Reference Black, Eberlein, Solyman, Wilkes, Hartmann, Rohrbach, Bemis and Kania2011). The genetic basis of antimicrobial resistance in S. pseudintermedius and other SIG was recently reviewed (Kadlec and Schwarz, Reference Kadlec and Schwarz2012).
Epidemiology of MRSA and MRSIG
MRSA carriage and infection in humans
Approximately 50% of people in the general population are carriers of S. aureus (Acton et al., Reference Acton, Plat-Sinnige, Van Wamel, De Groot and Van Belkum2009; Frank et al., Reference Frank, Feazel, Bessesen, Price, Janoff and Pace2010), but only about 1.5% of the population are carriers of MRSA according to CDC. However, certain population subgroups have a significantly higher prevalence of MRSA than others, including those older than 60 years (Enoch et al., Reference Enoch, Carter and Karas2010; Reilly et al., Reference Reilly, Stewart, Christie, Allardice, Smith, Masterton, Gould and Williams2010). Persons with occupational exposure to animals carrying MRSA are also more likely to carry related animal MRSA strains than the general population (Van Loo et al., Reference Van Loo, Huijsdens, Tiemersma, De Neeling, Van De Sande-Bruinsma, Beaujean, Voss and Kluytmans2007a, Reference Van Loo, Diederen, Savelkoul, Woudenberg, Roosendaal, Van Belkum, Toom, Verhulst, Van Keulen and Kluytmansb; Van Cleef et al., Reference Van Cleef, Broens, Voss, Huijsdens, Züchner, Van Benthem, Kluytmans, Mulders and Van De Giessen2010). Several studies have demonstrated that carriers of MRSA are at greater risk for developing serious infections compared to people who are not carriers.
MRSA, like MSSA, can cause a range of illness from relatively mild skin disorders to life threatening, invasive infections. MRSA has been a chronic problem in hospitals and long-term care facilities for over 40 years, particularly in surgical wards and intensive care units. Community-acquired infections typically affect skin and soft tissues, causing mild to moderate symptoms, often in healthy, younger people without the usual risk factors for healthcare-acquired MRSA. Severe, invasive community acquired MRSA infections also occur and there is evidence that these more severe infections are increasing as a more virulent strain, USA300, spreads (David and Daum, Reference David and Daum2010). At the same time, there is evidence that MRSA infections in hospitals have leveled off or decreased in the past 5 years (Hadler et al., Reference Hadler, Petit, Mandour and Cartter2012).
One troubling aspect of MRSA infections and colonizations is that they often appear to be chronic and bacteria can be detected in human tissues for extended periods. Persistence of MRSA in 403 German patients had a half-life of 549 days with the duration dependent on the site(s) colonized or infected (Mattner et al., Reference Mattner, Biertz, Ziesing, Gastmeier and Chaberny2010).
HA-MRSA
MRSA was first detected in a UK hospital in 1961 and a few years later was detected in U.S. hospitals and other health care facilities where the widespread use of antibiotics selected for drug resistant bacteria. Until the 1990s, MRSA continued to be almost exclusively an issue in hospitals and long-term care facilities. Some MRSA infections occurred in non-hospitalized persons, but these were usually close contacts of persons who had been hospitalized. Due to the high rate of antibiotic usage in health care facilities, HA-MRSA are often resistant to many classes of antibiotics in addition to the β-lactams. Five major lineages or CCs (CC5, CC8, CC22, CC30 and CC45) originated in hospitals and have spread globally. Most of them possess one of the larger SCCmec types I–III, which also carry genes for resistance to other antibiotics. Type II is most common among U.S. HA-MRSA isolates, whereas type III is found more often in other countries (David and Daum, Reference David and Daum2010; McCarthy et al., Reference McCarthy, Sullivan, Gaynes and Rimland2010). Two major genotypes of HA-MRSA are circulating in Asia: CC5 which is dominant in Korea and Japan and CC239 which is dominant in China, India, and several countries in southeast Asia (Ko et al., Reference Ko, Lee, Suh, Oh, Peck, Lee and Song2005; Li et al., Reference Li, Du, Villaruz, Diep, Wang, Song, Tian, Hu, Yu, Lu and Otto2012).
Community-associated MRSA (CA-MRSA)
Cases of MRSA that genuinely originated in the community were first reported from Australia in the early 1990s. MRSA isolates from these cases were not resistant to multiple antibiotics and genetic analyses revealed that they were different from other MRSA in Australia (Udo et al., Reference Udo, Pearman and Grubb1993; Coombs et al., Reference Coombs, Monecke, Ehricht, Slickers, Pearson, Tan, Christiansen and O'Brien2010). More frequent reports of CA-MRSA emerged in other countries in the late 1990s, primarily among healthy persons with skin and soft tissue infections. These CA-MRSA isolates were susceptible to more classes of antibiotics than HA-MRSA and they generally carried smaller, more mobile SCCmec elements, usually types IV or V (David and Daum, Reference David and Daum2010). Many CA-MRSA strains produce a toxin, PVL, that attacks white blood cells and is not commonly present in HA-MRSA (Li et al., Reference Li, Cheung, Hu, Wang, Joo, Deleo and Otto2010). More extensive information on the evolution, virulence, and epidemiology of CA-MRSA can be found in a recent comprehensive review article (David and Daum, Reference David and Daum2010).
Several CA-MRSA clones originated in Europe (ST80), North America (ST1 and ST8), and Australia (ST30), and subsequently spread worldwide with reported cases in countries as diverse as South Africa, Nepal, Argentina, Saudi Arabia, Japan, Malaysia, and most countries in Europe (Tristan et al., Reference Tristan, Bes, Meugnier, Lina, Bozdogan, Courvalin, Reverdy, Enright, Vandenesch and Etienne2007). A great deal of genetic diversity exists in currently circulating CA-MRSA in Europe (Rolo et al., Reference Rolo, Miragaia, Turlej-Rogacka, Empel, Bouchami, Faria, Tavares, Hryniewicz, Fluit and De Lencastre2012). A highly virulent CA-MRSA clone, ST93 (Queensland CA-MRSA), has emerged in Australia and has now spread to become the dominant CA-MRSA there (Otto, Reference Otto2010; Coombs et al., Reference Coombs, Goering, Chua, Monecke, Howden, Stinear, Ehricht, O'Brien and Christiansen2012).
A particularly virulent clone, USA300 (ST8), first reported in a prison outbreak in 2000 (Culpepper et al., Reference Culpepper, Nolan, Chapman, Kennedy and Currier2001), now causes nearly all CA-MRSA cases in the U.S. USA300 infections have also been increasing rapidly in Canada (Kim et al., Reference Kim, Ferrato, Simmonds, Chui, Mulvey, Golding, Svenson and Louie2010) and this clone is now a major international epidemic strain having spread to numerous countries on five continents (Nimmo, Reference Nimmo2012). USA300 contains SCCmec type IV and a gene encoding PVL appears to be more capable of colonizing human epithelial surfaces and causing skin and soft tissue infections than other CA-MRSA clones (Thurlow et al., Reference Thurlow, Joshi and Richardson2012). In the past 5 years, USA300 has acquired a number of additional antibiotic resistance genes, apparently from USA100, a common HA-MRSA strain (Huang et al., Reference Huang, Tseng, Hu, Tsai, Hsueh and Teng2007; Kennedy et al., Reference Kennedy, Otto, Braughton, Whitney, Chen, Mathema, Mediavilla, Byrne, Parkins, Tenover, Kreiswirth, Musser and Deleo2008; McDougal et al., Reference McDougal, Fosheim, Nicholson, Bulens, Limbago, Shearer, Summers and Patel2010; David and Daum, Reference David and Daum2010).
Human HA-MRSA and CA-MRSA infections
In the past, categorizing MRSA as HA or CA, depending on where patients acquired infections, was useful. HA strains were more likely than CA strains to be resistant to multiple classes of antibiotics and therefore treatment for the two would differ. However, the emergence of CA-MRSA in healthcare settings and the appearance of HA-MRSA in the community, along with changes in virulence and the scope of antibiotic resistance have blurred distinctions between HA-MRSA and CA-MRSA.
CA-MRSA are now causing an increasing proportion of MRSA infections, including invasive infections, in hospitalized patients (Van De Griend et al., Reference Van De Griend, Herwaldt, Alvis, Demartino, Heilmann, Doern, Winokur, Vonstein and Diekema2009; Valsesia et al., Reference Valsesia, Rossi, Bertschy and Pfyffer2010; Zhanel et al., Reference Zhanel, DeCorby, Adam, Mulvey, McCracken, Lagacé-Wiens, Nichol, Wierzbowski, Baudry, Tailor, Karlowsky, Walkty, Schweizer, Johnson and Hoban2010; Tenover et al., Reference Tenover, Tickler, Goering, Kreiswirth, Mediavilla and Persing2012; Farr et al., Reference Farr, Aden, Weiss, Nash and Marx2012). An analysis of discharge data on 616,375 pediatric cases of skin and soft tissue infections occurring in the U.S. during a 10-year period revealed that hospitalizations for infections caused by CA-MRSA increased dramatically from <1 case/100,000 in 1996 to 25.5 cases/100,000 in 2006 (Frei et al., Reference Frei, Makos, Daniels and Oramasionwu2010). It has been predicted that CA-MRSA strains may eventually displace conventional strains of HA-MRSA. Therefore, it may now be more appropriate to define MRSA strains based on genetic differences revealed by SCCmec typing and MLST analysis in addition to noting whether MRSA was acquired in the hospital or the community and whether disease symptoms first appeared in the hospital or community (Otter and French, Reference Otter and French2011).
MRSA carriage and infection in animals
MRSA colonizes and infects a variety of animals, including livestock, companion animals and some wild animals. Epidemiology of MRSA and predominant strains differ in different animals; human-associated MRSA strains are often isolated from pets while some horse- and livestock-adapted strains are more commonly present in other animals (McCarthy et al., Reference McCarthy, Lindsay and Loeffler2012; Petinaki and Spiliopoulou, Reference Petinaki and Spiliopoulou2012). The earliest published report of MRSA in farm animals described the detection of MRSA, in 1972, in dairy cows with mastitis in Belgium (Devriese and Hommez, Reference Devriese and Hommez1975). Although current methods for typing MRSA strains were not available then, it is believed that these cases resulted from human to animal transmission of HA-MRSA. Later reports documented HA-MRSA in horses, dogs, and other animals at veterinary clinics and hospitals (Hartmann et al., Reference Hartmann, Trostle and Klohnen1997; Seguin et al., Reference Seguin, Walker, Caron, Kloos, George, Hollis, Jones and Pfaller1999). Some later reports described dogs, horses and cats at veterinary hospitals with CA-MRSA infections (Middleton et al., Reference Middleton, Fales, Luby, Oaks, Sanchez, Mnyon, Wu, Maddox, Welsh and Hartmann2005).
A new MRSA strain, ST398, was first detected in 2003 in swine and swine farmers in the Netherlands and was designated LA (livestock associated) MRSA (Van Loo et al., Reference Van Loo, Huijsdens, Tiemersma, De Neeling, Van De Sande-Bruinsma, Beaujean, Voss and Kluytmans2007a, Reference Van Loo, Diederen, Savelkoul, Woudenberg, Roosendaal, Van Belkum, Toom, Verhulst, Van Keulen and Kluytmansb; Fluit, Reference Fluit2012). ST398 has also been detected in pigs and pig farmers in other European countries (Denis et al., Reference Denis, Suetens, Hallin, Catry, Ramboer, Dispas, Willems, Gordts, Butaye and Struelens2009; Köck et al., Reference Köck, Harlizius, Bressan, Laerberg, Wieler, Witte, Deurenberg, Voss, Becker and Friedrich2009; Pomba et al., Reference Pomba, Baptista, Couto, Loução and Hasman2010), the U.S. (Smith et al., Reference Smith, Male, Harper, Kroeger, Tinkler, Moritz, Capuano, Herwaldt and Diekema2009), and Canada (Khanna et al., Reference Khanna, Friendship, Dewey and Weese2008; Golding et al., Reference Golding, Bryden, Levett, McDonald, Wong, Wylie, Graham, Tyler, Van Domselaar, Simor, Gravel and Mulvey2010). ST398 has also been isolated from humans and other animals living on pig farms (Van De Giessen et al., Reference Van De Giessen, Van Santen-Verheuvel, Hengeveld, Bosch, Broens and Reusken2009; Cuny et al., Reference Cuny, Friedrich, Kozytska, Layer, Nübel, Ohlsen, Strommenger, Walther, Wieler and Witte2010; Hasman et al., Reference Hasman, Moodley, Guardabassi, Stegger, Skov and Aarestrup2010). Surveys indicate that pigs, veal calves, and broilers are the main reservoirs for ST398 (ECDC Reference ECDC2009), but this strain has also been detected in dairy cattle and milk (Kreausukon et al., Reference Kreausukon, Fetsch, Kraushaar, Alt, Mueller, Kroemker, Zessin, Kaesbohrer and Tenhagen2012; Tavakol et al., Reference Tavakol, Riekerink, Sampimon, Van Wamel, Van Belkum and Lam2012). A different swine-associated MRSA strain, CC9, is circulating among pigs and pig farmers in China (Cui et al., Reference Cui, Li, Hu, Jin, Li, Guo, Ran and Ma2009; Ho et al., Reference Ho, Chow, Lai, Law, Chan, Ho, Ng and Yam2012).
It appears, from genome analysis of numerous human and animal strains belonging to the CC 398, that the LA-MRSA ST398 was derived from a human MSSA ST398 strain that was transferred to pigs. When this occurred, some genes coding for immune modulators specific to humans were lost and the ST398 in pigs acquired resistance to tetracycline and methicillin (Price et al., Reference Price, Stegger, Hasman, Aziz, Larsen, Andersen, Pearson, Waters, Foster, Schupp, Gillece, Driebe, Liu, Springer, Zdovc, Battisti, Franco, Zmudzki, Schwarz, Butaye, Jouy, Pomba, Conception-Porrero, Ruimy, Smith, Robinson, Weese, Arriola, Yu, Laurent, Keim, Skov and Aarestrup2012; Jamrozy et al., Reference Jamrozy, Fielder, Butaye and Coldham2012). The loss of genes specific for human infections appears to limit transmission of ST398 among people (Bootsma et al., Reference Bootsma, Wassenberg, Trapman and Bonten2011). Over 70% of 54 LA-MRSA strains tested were resistant to three or more classes of antibiotics. All of the strains tested were PVL negative and only four strains had genes coding for enterotoxins (Kadlec et al., Reference Kadlec, Ehricht, Monecke, Steinacker, Kaspar, Mankertz and Schwarz2009, Reference Kadlec, Fessler, Hauschild and Schwarz2012).
Certain horse-adapted MRSA strains have been described and have apparently been transmitted to humans (Catry et al., Reference Catry, Van Duijkeren, Pomba, Greko, Moreno, Pyörälä, Ružauskas, Sanders, Threlfall, Ungemach, Törneke, Muñoz-Madero and Torren-Edo2010). A majority of horse isolates in Canada belong to a subtype of the Canadian epidemic strain, MRSA-5, which has a type IV SCCmec. This strain is also present in horses in other countries and has been reported in numerous people working with horses (Weese et al., Reference Weese, Archambault, Willey, Dick, Hearn, Kreiswirth, Said-Salim, McGeer, Likhoshvay, Prescott and Low2005; Catry et al., Reference Catry, Van Duijkeren, Pomba, Greko, Moreno, Pyörälä, Ružauskas, Sanders, Threlfall, Ungemach, Törneke, Muñoz-Madero and Torren-Edo2010).
Swine
Of all livestock, swine appear to most commonly harbor MRSA. In most cases, MRSA does not appear to seriously affect the health of pigs, but there have been reports of MRSA in pathological lesions in pigs (Atyah et al., Reference Atyah, Zamri-Saad and Siti-Zahrah2010; Meemken et al., Reference Meemken, Blaha, Tegeler, Tenhagen, Guerra, Hammerl, Hertwig, Käsbohrer, Appel and Fetsch2010; Vanderhaeghen et al., Reference Vanderhaeghen, Hermans, Haesebrouck and Butaye2010b). Data from the EU on MRSA in 4597 swine holdings (breeding and production) in 26 countries revealed that overall 14% of breeding and 27% of production herds tested positive for MRSA. However, in some countries, no herds tested positive, whereas in others, up to 51% of holdings contained MRSA. Highest prevalence of MRSA was recorded in Spain, Germany, Belgium, and Italy. LA-MRSA (ST398) accounted for 92.5% of isolates tested (European Food Safety Authority, 2009).
Other recent surveys report the presence of MRSA in:
• 70% of pigs sampled at one U.S. production facility and none of the pigs at another facility. Isolates were ST398 (Smith et al., Reference Smith, Male, Harper, Kroeger, Tinkler, Moritz, Capuano, Herwaldt and Diekema2009).
• 2.9% of pigs tested on 10 Ohio farms (Molla et al., Reference Molla, Byrne, Abley, Mathews, Jackson, Fedorka-Cray, Sreevatsan, Wang and Gebreyes2012).
• 58–79% in pigs in Spain and Hungary sampled in 2010 (European Food Safety Authority and European Centre for Disease Prevention and Control, 2012).
• 4.6% of pigs on 45 Canadian farms. The predominant strain was ST398 (Weese et al., Reference Weese, Rousseau, Deckert, Gow and Reid-Smith2011).
• 70% of German pig farms. All were ST398 (Köck et al., Reference Köck, Harlizius, Bressan, Laerberg, Wieler, Witte, Deurenberg, Voss, Becker and Friedrich2009).
• 71% of Dutch finishing pig herds and 67% of breeding herds (Broens et al., Reference Broens, Graat, Van Der Wolf, Van De Giessen and De Jong2011).
• 44% of pigs 50 Belgian farms (Crombée et al., Reference Crombé, Willems, Dispas, Hallin, Denis, Suetens, Gordts, Struelens and Butaye2012).
• 0.9% of swine nasal samples in Japan. No ST398 was detected (Baba et al., Reference Baba, Ishihara, Ozawa, Tamura and Asai2010).
Factors positively associated with prevalence of MRSA in swine include larger herd sizes and greater numbers of imported pigs (European Food Safety Authority, 2010; Broens et al., Reference Broens, Graat, Van Der Wolf, Van De Giessen and De Jong2011), ‘open’ versus ‘closed’ farms (Crombé et al., Reference Crombé, Willems, Dispas, Hallin, Denis, Suetens, Gordts, Struelens and Butaye2012), conventional rearing of pigs as compared to organic rearing (Meemken and Blaha, Reference Meemken and Blaha2009) and poorer hygiene practices (Nathaus et al., Reference Nathaus, Blaha, Tegeler and Meemken2010). Trading of MRSA colonized pigs between farms was documented as a mode of transmission to new farms as molecular typing confirmed that MRSA isolates at the receiving farm were indistinguishable from those at the supplying farm (Espinosa-Gongora et al., Reference Espinosa-Gongora, Broens, Moodley, Nielsen and Guardabassi2012). LA-MRSA, ST398, readily spread from pigs nasally inoculated with a low dose to other pigs in the same pen. MRSA was not detected in feces, but was present in lymph nodes (Jouy et al., Reference Jouy, Le Roux, Kéranflec'h, Granier, Laurent, Kempf, Brisabois, Cariolet and Chauvin2012).
Cattle
S. aureus is a significant cause of mastitis in cows and small ruminants (Vanderhaeghen et al., Reference Vanderhaeghen, Hermans, Haesebrouck and Butaye2010b). However, the prevalence of methicillin-resistant strains in European cows appears to be low, although there is some intercountry variation (Hendriksen et al., Reference Hendriksen, Mevius, Schroeter, Teale, Meunier, Butaye, Franco, Utinane, Amado, Moreno, Greko, Stärk, Berghold, Myllyniemi, Wasyl, Sunde and Aarestrup2008; Alves et al., Reference Alves, McCulloch, Even, Le Maréchal, Thierry, Grosset, Azevedo, Rosa, Vautor and Le Loir2009). CA-, HA-, and LA-MRSA were all recently detected in bulk tank milk from cows in Minnesota (Haran et al., Reference Haran, Godden, Bender and Sreevatsan2010). Human-associated MRSA strains have also been detected in mastitic cows in Hungary (Juhász-Kaszanyitzky et al., Reference Juhász-Kaszanyitzky, Jánosi, Somogyi, Dan, Van Der Graaf-Van, Van Dulijkeren and Wagenaar2007), Korea (Nam et al., Reference Nam, Lee, Jung, Kim, Jang, Wee and Lim2011), and Turkey (Turutoglu et al., Reference Turutoglu, Hasoksuz, Ozturk, Yildirim and Sagnak2009), and recent studies have demonstrated that ST398 is present in German (Fessler et al., Reference Fessler, Scott, Kadlec, Ehricht, Monecke and Schwarz2010; Spohr et al., Reference Spohr, Rau, Friedrich, Klittich, Fetsch, Guerra, Hammerl and Tenhagen2011), Dutch (Tavakol et al., Reference Tavakol, Riekerink, Sampimon, Van Wamel, Van Belkum and Lam2012), and Belgian (Vanderhaeghen et al., Reference Vanderhaeghen, Cerpentier, Adriaensen, Vicca, Hermans and Butaye2010a) cows. Some healthy dairy cows in Denmark carry a CC130 strain that harbors a new mecC gene and these MRSA strains can be transferred between cattle and humans (Petersen et al., Reference Petersen, Stegger, Heltberg, Christensen, Zeuthen, Knudson, Urth, Sorum, Schouls, Larsen, Skov and Larsen2012).
MRSA has been detected in retail beef, but nasal and fecal sampling of nearly 500 Canadian feedlot cattle, shortly before slaughter, detected no MRSA (Weese et al., Reference Weese, Reid-Smith, Rousseau and Avery2012b). MRSA was detected in 18–31% of veal calves on Dutch farms. Prevalence was strongly correlated with the use of antimicrobials and was also related to management and hygiene practices on different farms (Bos et al., Reference Bos, Graveland, Portengen, Wagenaar and Heederik2012). In 2010, the European Union reported that 20% of veal calves in Germany carried MRSA (European Food Safety Authority and European Centre for Disease Prevention and Control, 2012).
Poultry
Methicillin resistance was observed in S. aureus isolates from chickens in Korea in 2001–2003 (Lee, Reference Lee2003). MRSA was later detected in broiler chickens in Belgium (Nemati et al., Reference Nemati, Hermans, Lipinska, Denis, Deplano, Struelens, Devriese, Pasmans and Haesebrouck2008; Persoons et al., Reference Persoons, Van Hoorebeke, Hermans, Butaye, De Kruif, Haesebrouck and Dewulf2009) and in broilers, but not in breeder chickens in the Netherlands (Mulders et al., Reference Mulders, Haenen, Geenen, Vesseur, Poldervaart, Bosch, Huijsdens, Hengeveld, Dam-Deisz, Graat, Mevius, Voss and Van De Giessen2010). These isolates were identified primarily as the livestock associated strain, ST398. A survey of the prevalence of MRSA in broilers on three Belgian mixed poultry-pig farms revealed that from 0 to 28% of chickens carried MRSA strain 398 as compared to 82–92% of pigs on the same farms. No MRSA was isolated from barns containing chickens and it may be that the shorter production period for broilers and more frequent disinfection of their quarters explains the lower prevalence of MRSA. Chickens may also be naturally less susceptible to MRSA infection (Pletinckx et al., Reference Pletinckx, Verhegghe, Dewulf, Crombé, De Bleecker, Rasschaert, Goddeeris and De Man2011). A report on MRSA in animals, sampled in 2010, in countries in the European Union reported that 20–46% of turkeys in Germany and Hungary carried MRSA. MRSA was also detected in 71% of chickens tested in Hungary, but was not found in 398 flocks of broilers in Switzerland (European Food Safety Authority and European Centre for Disease Prevention and Control, 2012).
Horses
MRSA was first reported in a horse with a surgical wound in 1996 (Hartmann et al., Reference Hartmann, Trostle and Klohnen1997). Transmission of MRSA from humans appeared to cause the early infections in horses in veterinary hospitals and is still a factor (Seguin et al., Reference Seguin, Walker, Caron, Kloos, George, Hollis, Jones and Pfaller1999; Couto et al., Reference Couto, Tilley, Simoes, Sales Luis and Pomba2012). Surveys of horses on farms usually report a low prevalence of MRSA of 0–4.7%. A higher prevalence (up to 12%) has been observed in horses admitted to veterinary hospitals (Tokateloff et al., Reference Tokateloff, Manning, Weese, Campbell, Rothenburger, Stephen, Bastura, Gow and Reid-Smith2009; Weese and Van Duijkeren, Reference Weese and Van Duijkeren2010). The most common MRSA strain now identified in horses in Europe, North America, and Australia, Canadian CMRSA-5 or USA500, is a member of the CC8 clone (Abbott et al., Reference Abbott, Leonard and Markey2010b). Although this clone appears to be of human origin, it seldom causes illness in humans and now appears to be horse-adapted (Weese et al., Reference Weese, Rousseau, Willey, Archambault, Mcgeer and Low2006; Axon et al., Reference Axon, Carrick, Barton, Collins, Russell, Kiehne and Coombs2011). Unlike other farm animals that are primarily transported only for slaughter, horses are transported internationally for breeding, racing, and show-jumping, and these movements have contributed to the spread of this clone. Recently, there have been reports of the livestock-associated (LA) strain ST398 in horses (Loeffler and Lloyd, Reference Loeffler and Lloyd2010; Van Duijkeren et al., Reference Van Duijkeren, Moleman, Sloet Van Oldruitenborgh-Oosterbaan, Multem, Troelstra, Fluit, Van Wamel, Houwers, De Neeling and Wagenaar2010), including veterinary hospital outbreaks in Finland (Salmenlinna et al., Reference Salmenlinna, Lyytikäinen, Vainio, Myllyniemi, Raulo, Kanerva, Rantala, Thomson, Seppänen and Vuopio2010) and Sweden (Bergstrom et al., Reference Bergstrom, Aspan, Landen, Johnston and Gronlund-Andersson2012a). Tracking of antibiotic resistant S. aureus strains in a Swiss veterinary hospital over a 6-year period revealed that strain ST398 was first detected in an employee in 2006 and then appeared in 10–15% of horses as ST398 rapidly replaced the antibiotic resistant strains of human origin within 6 months (Sieber et al., Reference Sieber, Gerber, Jandova, Rossano, Evison and Perreten2011).
Dogs and cats
A human-associated MRSA was first detected in companion animals in Nigeria in 1972 (Loeffler and Lloyd, Reference Loeffler and Lloyd2010). Later, MRSA was detected in dogs with surgical wounds or skin infections (Gortel et al., Reference Gortel, Campbell, Kakoma, Whittem, Schaeffer and Weisiger1999; Tomlin et al., Reference Tomlin, Pead, Lloyd, Howell, Hartmann, Jackson and Muir1999) and the use of veterinary drugs and IV catheters were identified as risk factors for MRSA infections in dogs (Faires et al., Reference Faires, Traverse, Tater, Pearl and Weese2010; Magalhães et al., Reference Magalhães, Loeffler, Lindsay, Rich, Roberts, Smith, Lloyd and Pfeiffer2010). Generally, cases of MRSA infection in dogs and cats involve lesions in the skin or ears but invasive infections sometimes occur. Healthy dogs and cats can also transiently carry MRSA asymptomatically (Weese, Reference Weese2005; Morris et al., Reference Morris, Lautenbach, Zaoutis, Leckerman, Edelstein and Rankin2012). Surveys indicate that prevalence of MRSA in companion animals is low (<2%) (Leonard and Markey, Reference Leonard and Markey2008). Epidemiology of MRSA in companion animals was recently reviewed (Loeffler and Lloyd, Reference Loeffler and Lloyd2010).
MRSA strains isolated from companion animals are primarily HA-MRSA (Abbott et al., Reference Abbott, Leggett, Rossney, Leonard and Markey2010a) and these have been detected in therapy dogs and cats visiting human long-term care facilities (Lefebvre and Weese, Reference Lefebvre and Weese2009; Coughlan et al., Reference Coughlan, Olsen, Boxrud and Bender2010). However, both CA-MRSA (Van Duijkeren et al., Reference Van Duijkeren, Wolfhagen, Heck and Wannet2005) and LA-MRSA (Witte et al., Reference Witte, Strommenger, Stanek and Cuny2007; Floras et al., Reference Floras, Lawn, Slavic, Golding, Mulvey and Weese2010) have caused infections in dogs. Similar MRSA strains have been detected in dogs and their owners but surveys of dogs or humans colonized with MRSA have demonstrated that only a small number of human–dog pairs are infected with the same MRSA strain (Boost et al., Reference Boost, O'Donoghue and James2008; Faires et al., Reference Faires, Tater and Weese2009b; Morris et al., Reference Morris, Lautenbach, Zaoutis, Leckerman, Edelstein and Rankin2012). MRSA does not appear to spread easily from dog to dog (Loeffler et al., Reference Loeffler, Pfeiffer, Lindsay, Soares-Magalhães and Lloyd2010).
Other animals
In addition to the companion animals and livestock described above, MRSA has been detected in avian pets, including a parrot (Rich and Roberts, Reference Rich and Roberts2006; Rodriguez et al., Reference Rodriguez, Flores and Farinas2007), goats (Alves et al., Reference Alves, McCulloch, Even, Le Maréchal, Thierry, Grosset, Azevedo, Rosa, Vautor and Le Loir2009), sheep (Goñi et al., Reference Gońi, Vergara, Ruiz, Albizu, Vila and Gómez2004), farmed fish (Abrahim et al., Reference Abrahim, Sergelidis, Kirkoudis, Anagnostou, Kaitsa-Tsiopoulou, Kazila and Papa2010), wild rats living on a farm (Van De Giessen et al., Reference Van De Giessen, Van Santen-Verheuvel, Hengeveld, Bosch, Broens and Reusken2009), a zoo elephant (Janssen et al., Reference Janssen, Lamberski, Dunne, Ginsberg, Roach, Tweeten, Gorwitz, Waterman, Bensyl and Sugerman2009), seals, dolphins and walrus from marine parks/sanctuaries (O'Mahony et al., Reference O'Mahony, Abbott, Leonard, Markey, Quinn, Pollock, Fanning and Rossney2005; Faires et al., Reference Faires, Gehring, Mergl and Weese2009a), and guinea pig, rabbit, bat, and turtle in a veterinary hospital (Walther et al., Reference Walther, Wieler, Friedrich, Hanssen, Kohn, Brurmberg and Lübke-Becker2008). Origins of MRSA were unknown in some cases, but appeared to be from human caretakers for the birds, seal, and elephant calf and from pigs for the farm rats.
MRSA in foods
MRSA has been detected in raw meat (pork, poultry, and beef) and raw milk from countries throughout the world (Table 3). In some cases, the MRSA isolates were identified as HA- or CA-MRSA indicating that meat processors and other food handlers were likely to be the source of the bacteria (Pu et al., Reference Pu, Han and Ge2009; Hata et al., Reference Hata, Katsuda, Kobayashi, Uchida, Tanaka and Eguchi2010; Türkyilmaz et al., Reference Türkyilmaz, Tekbiyik, Oryasin and Bozdogan2010; Weese et al., Reference Weese, Avery and Reid-Smith2010a). In other surveys, LA-MRSA strains were the primary isolates, indicating an animal source of contamination (De Boer et al., 2008; Benedetti et al., Reference Benedetti, Cremonesi, Ferrari, Castiglioni, Fabbi, Vicari, Garbarino, Battisti, Franco, Feltrin and Luini2010; Vanderhaeghen et al., Reference Vanderhaeghen, Cerpentier, Adriaensen, Vicca, Hermans and Butaye2010a; Weese et al., Reference Weese, Avery and Reid-Smith2010a, Reference Weese, Reid-Smith, Rousseau and Averyb; Fessler et al., Reference Fessler, Kadlec, Hassel, Hauschild, Eidam, Ehricht, Monecke and Schwarz2011; O'Brien et al., Reference O'Brien, Hanson, Farina, Wu, Simmering, Wardyn, Forshey, Kulick, Wallinga and Smith2012). LA-MRSA has been detected in lymph nodes of pigs and if these tissues are included in ground pork, they could be a source of MRSA in meat (Jouy et al., Reference Jouy, Le Roux, Kéranflec'h, Granier, Laurent, Kempf, Brisabois, Cariolet and Chauvin2012; Szabó et al., Reference Szabó, Beck, Friese, Fetsch, Tenhagen and Roesler2012).
Table 3. Reports of MRSA detected in foods
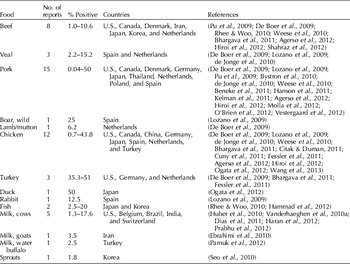
Reports of MRSA contamination in meats in Europe, North America and Asia have been published in an increasing number of scientific articles. Prevalence of contamination in samples tested ranges from relatively low (1–3%) (Bhargava et al., Reference Bhargava, Wang, Donabedian, Zervos, da Rocha and Zhang2011; Hanson et al., Reference Hanson, Dressler, Harper, Scheibel, Wardyn, Roberts, Kroeger and Smith2011; Agersø et al., Reference Agersø, Hasman, Cavaco, Pedersen and Aarestrup2012) to as many as a quarter to a third of the meat samples (Knödl et al., Reference Knödl, Yilmaz, Lohneis, Noack and Sabrowski2010; Fessler et al., Reference Fessler, Kadlec, Hassel, Hauschild, Eidam, Ehricht, Monecke and Schwarz2011). Since sample sizes and sampling and culture methods differed among the studies, results are not strictly comparable. Most of this research was aimed at detecting the presence of MRSA and there was no effort to quantify the bacteria. A recent Canadian study found that most positive meat samples contained <100 cfu g−1 (Weese et al., Reference Weese, Avery and Reid-Smith2010) and a Dutch study reported that most probable numbers (MPN) of MRSA in meat ranged from 0.06 (veal) to >10 (pork) bacteria per gram (de Jonge et al., Reference de Jonge, Verdier and Havealaar2010).
MRSA carried in pigs during lairage, prior to slaughter, can be detected at different stages during processing and in commercial pork products (Molla et al., Reference Molla, Byrne, Abley and Gebreyes2010). A study investigating the fate of MRSA on German pigs at slaughter and at several steps during processing found that 65% of pigs were positive at stunning. However, only 6% of carcasses on the slaughter line, 4.2% of meat samples during processing, and 3% of finished meat products tested positive (Beneke et al., Reference Beneke, Klees, Stuehrenberg, Fetsch, Kraushaar and Tenhagen2011). A Dutch survey of meat handlers found that they were not colonized with MRSA, but LA-MRSA was detected on meat (de Jonge et al., Reference de Jonge, Verdier and Havealaar2010).
S. aureus is a known cause of mastitis in ruminants and several studies reported MRSA in raw milk from cows in the U.S. and Europe (Benedetti et al., Reference Benedetti, Cremonesi, Ferrari, Castiglioni, Fabbi, Vicari, Garbarino, Battisti, Franco, Feltrin and Luini2010; Vanderhaeghen et al., Reference Vanderhaeghen, Cerpentier, Adriaensen, Vicca, Hermans and Butaye2010a; Haran et al., Reference Haran, Godden, Boxrud, Jawahir, Bender and Sreevatsan2012), from milk in South Africa, India, and Brazil (Prabhu et al., Reference Prabhu, Wilfred, Hegde and Kumar2012), and from goats (Ebrahimi et al., Reference Ebrahimi, Shams, Shahrokh and Mirshokraei2010) and water buffalo (Ateba et al., Reference Ateba, Mbewe, Moneoang and Bezuidenhout2010; Dias et al., Reference Dias, Silva, Oliveira, Fonseca, Sales and Silva2011; Pamuk et al., Reference Pamuk, Yildirim, Seker, Gurler and Kara2012). Some of these strains also produced enterotoxins. MRSA has also been detected in other foods, including lamb and mutton, rabbit, duck, wild boar meat, minimally processed vegetables, and fresh fish. Although S. aureus is not part of the normal flora of fish, MRSA and some other methicillin-resistant staphylococci have been detected on raw fish in Japan. These contaminants may be deposited on fish by workers with poor personal hygiene (Hammad et al., Reference Hammad, Watanabe, Fujii and Shimamoto2012).
MRSIG infection in animals and humans
Published reports generally indicate a low prevalence of MRSP in dogs and cats, but the reported prevalence of MRSP appears to be increasing in some areas (Jones et al., Reference Jones, Kania, Rohrbach, Frank and Bemis2007; Weese and Van Duijkeren, Reference Weese and Van Duijkeren2010; Nienhoff et al., Reference Nienhoff, Kadlec, Chaberny, Verspohl, Gerlach, Kreienbrock, Schwarz, Simon and Nolte2011a, Reference Nienhoff, Kadlec, Chaberny, Verspohl, Gerlach, Schwarz, Kreienbrock, Nolte and Simonb). Recent hospitalization and antibiotic use are positively correlated with the presence of MRSP in dogs (Nienhoff et al., Reference Nienhoff, Kadlec, Chaberny, Verspohl, Gerlach, Kreienbrock, Schwarz, Simon and Nolte2011a; Weese et al., Reference Weese, Faires, Frank, Reynolds and Battisti2012a). MRSP can persist in dogs for many months after clinical infections. Treatment of MRSP-infected dogs with systemic antibiotics to which the MRSP strains were resistant appeared to prolong carriage of these strains (Windahl et al., Reference Windahl, Reimegard, Holst, Egenvall, Fernstrom, Fredriksson, Trowald-Wigh and Andersson2012).
Dogs with clinical infections can contaminate their environment and bacteria can remain after ordinary cleaning and disinfection. Infected dogs can readily transmit MRSP to cats and other dogs. However, transmission to humans in the household and to veterinarians occurs at a lower rate than infection of other animals (Laarhoven et al., Reference Laarhoven, De Heus, Van Luijn, Duim, Wagenaar and Van Duijkeren2011; Van Duijkeren et al., Reference Van Duijkeren, Kamphuis, Van De Mije, Laarhoven, Duim, Wagenaar and Houwers2011b).
Methicillin resistance has been detected in other staphylococci including Staphylococcus schleiferi and Staphylococcus epidermidis from dogs (Kania et al., Reference Kania, Williamson, Frank, Wilkes, Jones and Bemis2004; Kawakami et al., Reference Kawakami, Shibata, Murayama, Nagata, Nishifuji, Iwasaki and Fukata2010), and several staphylococcal species on freshwater fish in Greece (Abrahim et al., Reference Abrahim, Sergelidis, Kirkoudis, Anagnostou, Kaitsa-Tsiopoulou, Kazila and Papa2010).
MRSP/MRSIG strains are seldom isolated from human food, but there is one report of MRSIG in camel meat in Jordan (Al-Tarazi et al., Reference Al-Tarazi, Albetar and Alaboudi2009). Methicillin-resistant coagulase-negative staphylococci have been detected in bulk tank milk and minced meat in Switzerland. These bacteria are not normally pathogenic, but could transfer their antibiotic resistance to S. aureus or other bacteria (Huber et al., Reference Huber, Ziegler, Pfluger, Vogel, Zweifel and Stephan2011).
Routes of infection
Staphylococci are spread among humans and animals and between species by direct physical contact or indirectly through clothing, towels, equipment, food, air, or surfaces contaminated by infected or colonized persons or animals.
Person to person
Hospital outbreaks of MRSA have been traced to lax hygiene practices among health care workers. MRSA transmission in a UK hospital was audited by swabbing patients’ skin and their environment and the hands of healthcare workers. MRSA was transmitted from a source, most commonly a patient's skin, to other patient skin areas, furniture, or note pads by the hands of healthcare workers in 22 of 24 cases. In one case, a doctor entering an intensive care unit with MRSA on his/her hands contaminated a notes trolley near a patient (Ludlam et al., Reference Ludlam, Swayne, Kearns, Brown, Howard, Gunning, Burnstein, Nicholl and Brown2010). Another study found that the frequency of transfer of MRSA from the skin of a colonized patient to a gloved hand was 40% (Stiefel et al., Reference Stiefel, Cadnum, Eckstein, Guerrero, Tima and Donskey2011). A study in four Danish hospitals found that the transmission rate of HA-MRSA from an introduced non-isolated patient to others in the hospital was about nine times greater than the transmission rate of CA-MRSA (Hetem et al., Reference Hetem, Westh, Boye, Jarlov, Bonten and Bootsma2012). A mathematical model has been developed to estimate the importance of numerous factors influencing the persistence and spread of MRSA in nursing homes. This model may aid in determining the most effective ways of controlling this pathogen in such communities (Chamchod and Ruan, Reference Chamchod and Ruan2012).
MRSA outbreaks in the community often occur in groups of people living in close quarters where they may transmit MRSA through direct physical contact or through commonly used items. Athletes participating in team sports are a recognized at-risk group. MRSA may be transmitted during direct physical contact during practices and games, and may also be present on towels or other equipment (Buss et al., Reference Buss, Mueller, Theis, Keyser and Safranek2009; Redziniak et al., Reference Redziniak, Diduch, Turman, Hart, Grindstaff, MacKnight and Mistry2009; Creech et al., Reference Creech, Saye, McKenna, Johnson, Jimenez, Talbot, Bossung, Gregory and Edwards2010). Several outbreaks of HA-MRSA have occurred in prisons where inmates share soap, shower infrequently, and are not well informed about prevention of staphylococcal infections. Other risk factors are related to pre-incarceration factors such as a low educational level and little contact with the health care system (Maree et al., Reference Maree, Eells, Tan, Bancroft, Malek, Harawa, Lewis, Santana and Miller2010; Malcolm, Reference Malcolm2011).
A cluster of CA-MRSA cases (strain USA300) in the Netherlands occurred in a beautician, her customers, family members, and contacts. Skin treatments (waxing) performed by the beautician were identified as the likely mode of transmission (Huijsdens et al., Reference Huijsdens, Janssen, Renders, Leenders, Van Wijk, Van Santen-Verheuvel, Van-Driel and Morroy2008).
Airborne transmission
MRSA is present in the nose and on the skin, and is shed into the environment by infected or colonized people and animals, indicating that airborne transmission is a possible route for infection. MRSA strains, identical to clinical isolates from patients, were detected in the air of hospital rooms (Gehanno et al., Reference Gehanno, Louvel, Nouvellon, Caillard and Pestel-Caron2009). LA-MRSA was detected in the air inside commercial pig barns in Germany at concentrations ranging from 6 to 3619 CFU m−3 and at lower concentrations (11–14 CFU m−3) in air samples taken 150 m downwind from the barns. LA-MRSA was also present on soil surfaces 300 m downwind from the barns. Airborne transmission and deposition does occur around barns containing MRSA-positive pigs and this is likely affected by wind speed and direction as well as temperature and rainfall (Friese et al., Reference Friese, Schulz, Hoehle, Fetsch, Tenhagen, Hartung and Roesler2012; Schulz et al., Reference Schulz, Friese, Klees, Tenhagen, Fetsch, Rosler and Hartung2012).
Animal contact
LA-MRSA ST398 was first described in pigs in the Netherlands in 2003 (Voss et al., Reference Voss, Loeffen, Bakker, Klaassen and Wulf2005; De Neeling et al., Reference De Neeling, Van Den Broek, Spalburg, Van Santen-Verheuvel, Dam-Deisz, Boshuizen, De Giessen, Van Duijkeren and Huijsdens2007). Subsequent studies reported detection of this strain among farmers and a survey indicated that human carriers of ST398 were 12.2–19.7 times more likely to be pig or cattle farmers than to be workers at other jobs (Van Loo et al., Reference Van Loo, Huijsdens, Tiemersma, De Neeling, Van De Sande-Bruinsma, Beaujean, Voss and Kluytmans2007a, Reference Van Loo, Diederen, Savelkoul, Woudenberg, Roosendaal, Van Belkum, Toom, Verhulst, Van Keulen and Kluytmansb). While the overall number of MRSA infections in the Netherlands appears to have stabilized, an increasing percentage of MRSA infections in the country are caused by this LA strain, even among people without known exposure to pigs or veal calves. Total MRSA isolates submitted to the national laboratory in the Netherlands in 2008 numbered 2693. Of these, 42% were identified as the LA-MRSA ST398 strain as compared to 30% in 2007 and 14% in 2006. Only 29% of people surveyed indicated contact with live pigs or veal calves (Haenen et al., Reference Haenen, Huijsdens, Pluister, van Luit, Bosch, van Santen-Verheuvel, Spalburg, Heck, de Neeling and Mulders2010). A recent study in Germany found that 86% of farmers and 45% of veterinarians exposed to pigs with ST398 also carried this strain. However, it was not readily transmitted from the workers to others as only 4–9% of family members and other close contacts tested positive for ST398 (Cuny et al., Reference Cuny, Nathaus, Layer, Strommenger, Altmann and Witte2009). LA-MRSA ST398 was reported in 1.4% of Danish livestock veterinarians and in 7.5% of Belgian veterinarians (Garcia-Graells et al., Reference Garcia-Graells, Antoine, Larsen, Catry, Skov and Denis2012). An increased risk for MRSA ST398 colonization was observed in persons who visited farms to purchase milk or eggs (Bisdorff et al., Reference Bisdorff, Scholhölter, Claussen, Pulz, Nowak and Radon2012). A survey of 51 veal calf farms in the Netherlands indicated that an average of 38% of farmers and 16% of family members were colonized with LA-MRSA. However, carrier status decreased dramatically when farmers took a break from direct animal care duties. Farm hygiene and antibiotic administration to calves were related to MRSA carriage in calves (Graveland et al., Reference Graveland, Wagenaar, Heesterbeek, Mevius, Van Duijkeren and Heederik2010, Reference Graveland, Wagenaar, Bergs, Heesterbeek and Heederik2011). Another study of pig farmers on holiday found that 59% did not clear carriage of MRSA while on vacation (Köck et al., Reference Köck, Loth, Köksal, Schulte-Wülwer, Harlizius and Friedrich2012). LA-MRSA can be acquired by humans with short-term occupational exposure to infected/colonized animals, but in most cases the strain is lost after a day (Van Cleef et al., Reference Van Cleef, Graveland, Haenen, Van De Giessen, Heederik, Wagenaar and Kluytmans2011). Studies of carriage of ST398 in workers at Dutch pig slaughterhouses found that 11 of 341 workers tested positive and that those working in lairage and the scalding and dehairing areas were most likely to be colonized (Gilbert et al., Reference Gilbert, Bos, Duim, Urlings, Heres, Wagenaar and Heederik2012).
Children and others interacting with animals at fairs and petting zoos are known to be exposed to zoonotic bacteria and viruses and some Escherichia coli and Salmonella outbreaks have been traced to these locales. A survey of 157 pigs at two state fairs detected S. aureus in 25 animals and MRSA in two pigs (Dressler et al., Reference Dressler, Scheibel, Wardyn, Harper, Hanson, Kroeger, Diekema, Bender, Gray and Smith2012). Although MRSA infections have not been traced to fairs, this is a potential site for transmission.
Horses may be colonized or infected with an uncommon horse-adapted MRSA strain, CMRSA-5, and several studies have reported this strain in horse caretakers and veterinarians (Weese et al., Reference Weese, Archambault, Willey, Dick, Hearn, Kreiswirth, Said-Salim, McGeer, Likhoshvay, Prescott and Low2005; Abbott et al., Reference Abbott, Leonard and Markey2010b). Australian veterinarians whose major emphasis was treatment of horses were found to be more likely than other veterinarians to be carriers of MRSA (Jordan et al., Reference Jordan, Simon, Fury, Moss, Giffard, Maiwald, Southwell, Barton, Axon, Morris and Trott2011). Suspected transmission of MRSA ST398 from a horse to a human has also been reported (Van Duijkeren et al., Reference Van Duijkeren, Ten Horn, Wagenaar, De Bruijn, Laarhoven, Verstappen, De Weerd, Meessen and Duim2011).
Companion animals may also be carriers of MRSA and MRSP. MRSA was first detected in a companion animal in a ward cat in a geriatric rehabilitation unit in England. The cat was apparently infected by a resident and then served as a reservoir spreading the infection to human residents (Scott et al., Reference Scott, Thomson, Malonelee and Ridgway1988). HA-MRSA strains have been detected in therapy dogs and cats visiting human long-term care facilities and may be a source of infection to residents (Lefebvre and Weese, Reference Lefebvre and Weese2009; Coughlan et al., Reference Coughlan, Olsen, Boxrud and Bender2010).
Similar MRSA and MRSP strains have been detected in dogs and their owners, but surveys of dogs or humans colonized with MRSA have demonstrated that only a small number of human–dog pairs are infected with the same MRSA strain. Evidence indicates that pets can acquire MRSA from humans and dog owners and veterinarians may also acquire MRSP from dogs (Boost et al., Reference Boost, O'Donoghue and James2008; Faires et al., Reference Faires, Tater and Weese2009b; Paul et al., Reference Paul, Moodley, Ghibaudo and Guardabassi2011; Soedarmanto et al., Reference Soedarmanto, Kanbar, Ulbegi-Mohyla, Hijazin, Alber, Lammler, Akineden, Weiss, Moritz and Zschock2011; Walther et al., Reference Walther, Hermes, Cuny, Wieler, Vincze, Abou Elnaga, Stamm, Kopp, Kohn, Witte, Jansen, Conraths, Semmler, Eckmanns and Luebke-Becker2012).
Contaminated equipment and surfaces
Surfaces and equipment in healthcare facilities may be contaminated with MRSA for extended periods. CA-MRSA (USA300) inoculated on to various fomites (towels, sheets, ceramic, wood, vinyl, and plastic) survived for extended periods and were transmissible for up to 8 weeks. Transmissibility decreased more rapidly from porous surfaces. HA-MRSA tested were not as readily transmitted from fomites (Desai et al., Reference Desai, Pannaraj, Agopian, Sugar, Liu and Miller2011). MRSA was detected on about 10% of 183 mobile phones belonging to nurses, laboratory workers and other health care staff (Ustun and Cihangiroglu, Reference Ustun and Cihangiroglu2012). Investigations at a veterinary hospital found 12% of environmental samples harbored MRSA (usually USA100) and that surfaces frequently touched by people, such as doors, or by animals, for example carts, were most likely to be contaminated with MRSA (Scott et al., Reference Scott, Duty and Callahan2008; Hoet et al., Reference Hoet, Johnson, Nava-Hoet, Bateman, Hillier, Dyce, Gebreyes and Wittum2011). Transmission of MRSA in healthcare facilities can occur by touching contaminated surfaces; gloved hands can pick up MRSA from bedrails, call buttons, tables, and phones at a frequency of 45% (Stiefel et al., Reference Stiefel, Cadnum, Eckstein, Guerrero, Tima and Donskey2011).
Patients with end stage renal disease (ESRD) are particularly vulnerable to invasive S. aureus infections because their blood must be treated using dialysis machines at least three times per week. These patients are frequently hospitalized, receive long courses of antibiotic treatment, and 14% die annually as a result of infections. Incidence of invasive MRSA was estimated at 45.2 cases/1000 population among dialysis patients, the highest for any patient population and about 100 times greater than the incidence in the general population (Collins et al., Reference Collins, Forrest, Klevens, Patel, Arduino, Fosheim, Morrison and Lucero2007). An increasing proportion of MRSA infections in ESRD patients is due to CA-MRSA strains (Johnson et al., Reference Johnson, Venugopal, Pawlak and Saravolatz2006).
MRSA has also been detected on a variety of surfaces outside of healthcare facilities. Contamination of household surfaces is a source of infection for people and pets (Bocher et al., Reference Bocher, Skov, Knudsen, Guardabassi, Molbak, Schouenborg, Sorum and Westh2010; Davis et al., Reference Davis, Iverson, Baron, Vasse, Silbergeld, Lautenbach and Morris2012). A swab survey of 400 ATM machines in Hong Kong found that over 15% had detectable levels of S. aureus, whereas MRSA was present on only two machines. Many of the antibiotic-resistant S. aureus isolates also carried genes encoding resistance to antiseptics (Zhang et al., Reference Zhang, O'Dononghue and Boost2012). CA-MRSA strains on contaminated needles have been transmitted among illicit drug users (Kreisel et al., Reference Kreisel, Johnson, Stine, Shardell, Perencevich, Lesse, Gordin, Climo and Roghmann2010) and non-sterile equipment used in tattooing was cited as the cause of CA-MRSA infections in tattoo recipients in several states (Long et al., Reference Long, Coleman, Dietsch, McGrath, Brady, Thomas, Corzatt, Ruta, Duffy, Koch, Trent, Thayer, Heath, Lohff, Hageman, Jernigan and LeMaile-Williams2006).
Numerous reports have detailed outbreaks in high school and collegiate athletes where MRSA was detected on equipment and surfaces in athletic facilities and on towels and clothing (Bowers et al., Reference Bowers, Huffman and Sennett2008; Redziniak et al., Reference Redziniak, Diduch, Turman, Hart, Grindstaff, MacKnight and Mistry2009). Experiments testing survival of CA-MRSA on artificial turf indicated that these bacteria could survive for at least a week in large numbers and for a month at lower concentrations, depending on environmental conditions (Waninger et al., Reference Waninger, Rooney, Miller, Berberian, Fujimoto and Buttaro2011). MRSA has been detected at relatively low levels in beach sand and water in California (Goodwin et al., Reference Goodwin, McNay, Cao, Ebentier, Madison and Griffith2012).
Contaminated food
MRSA strains have been detected in meat and milk and may also be present in a variety of other foods (Table 3). The origin of these contaminants has been traced to infected/colonized food handlers in some outbreaks (Kluytmans et al., Reference Kluytmans, van Leeuwen, Goessens, Hollis, Messer, Herwaldt, Bruining, Heck, Rost, van Leeuwen, van Belkum and Verbrugh1995; Jones et al., Reference Jones, Kellum, Porter, Bell and Schaffner2002). Studies have demonstrated that meat can also become contaminated during slaughter and processing of animals carrying MRSA (Molla et al., Reference Molla, Byrne, Abley and Gebreyes2010; Beneke et al., Reference Beneke, Klees, Stuehrenberg, Fetsch, Kraushaar and Tenhagen2011). In some surveys, MRSA detected on meat was identified as the LA strain, ST398 (de Jonge et al., Reference de Jonge, Verdier and Havealaar2010).
Significance of MRSA contamination of foods remains uncertain. If meat and other foods are cooked properly, MRSA cells will be killed. However, as with enterotoxigenic MSSA strains, under conditions of temperature abuse, MRSA cells could grow in foods, produce heat-stable enterotoxins, and cause food-borne intoxication. In some individuals, whose normal flora has been depleted by antibiotic treatment, MRSA cells on ready-to-eat foods, including processed meats, cheeses, and fresh produce, could cause staphylococcal enterocolitis. Finally, MRSA present on foods could potentially cause skin infections in food handlers. To date, there have been only two reported outbreaks associated with MRSA-contaminated food. A community outbreak of food-borne illness caused by CA-MRSA occurred in Tennessee in 2000 (Jones et al., Reference Jones, Kellum, Porter, Bell and Schaffner2002). Identical MRSA isolates were recovered from three ill persons, the coleslaw they purchased from a convenience store deli, and the nose of a food handler at the convenience store. This strain produced enterotoxin C. The second reported outbreak of MRSA occurred in a Dutch hospital and affected 27 patients and 14 health care workers from 1992 to 1993, resulting in five deaths. Epidemiological investigations indicated that a colonized food handler apparently contaminated food (a peeled banana tested positive for MRSA) served to hospital patients and some nurses may have inadvertently spread the bacteria to different wards (Kluytmans et al., Reference Kluytmans, van Leeuwen, Goessens, Hollis, Messer, Herwaldt, Bruining, Heck, Rost, van Leeuwen, van Belkum and Verbrugh1995).
MRSA does not appear to be transferred readily from meat to meat handlers. It was not detected on hands or in noses of 89 persons working in cold meat processing facilities or institutional kitchens in the Netherlands even though 14% of samples of meat (veal, pork, and chicken) that they worked with did contain MRSA. Most of the MRSA isolates were identified as ST398, LA MRSA (de Jonge et al., Reference de Jonge, Verdier and Havealaar2010). However, MRSA swabbed on pork loins was transferred at low levels to knives and cutting boards, indicating the potential for cross contamination and exposure in persons working with pork (Snyder et al., Reference Snyder, Dickson and Niebuhr2012).
Other methicillin-resistant staphylococci could potentially cause food-borne intoxication, but no cases have been reported. Methicillin-resistant S. intermedius was detected in camel meat in Jordan (Al-Tarazi et al., Reference Al-Tarazi, Albetar and Alaboudi2009). MRSIG strains have been detected in horses, but are not usually present in livestock (Weese and Van Duijkeren, Reference Weese and Van Duijkeren2010).
Control and prevention
Prevention of staphylococcal infections/intoxication requires strategies to interrupt various modes of transmission. Essentially these control programs include improvements in personal hygiene practices among health care workers and food handlers, decontamination of equipment, surfaces, and clothing, judicious use of antibiotics, proper cooking and storage of foods, and screening programs (Skov et al., Reference Skov, Christiansen, Dancer, Daum, Dryden, Huang and Lowy2012).
Hospital and healthcare programs
Increased morbidity and mortality among hospital patients infected with MRSA has led to development of some effective control procedures and strict enforcement of MRSA control policies has been found to decrease rates of MRSA infection in hospitals in 10 European countries (Hansen et al., Reference Hansen, Schwab, Asensio, Carsauw, Heczko, Klavs, Lyytikäinen, Palomar, Riesenfeld-Orn, Savey, Szilagyi, Valinteliene, Fabry and Gastmeier2010). An important feature of these MRSA-control programs is screening of patients at admission to ascertain which patients are carriers of MRSA so they can be isolated and treated to prevent transmission to other patients. Intensive programs to improve hygiene and worker training have also been effective in reducing MRSA infections in veterinary hospitals (Bergstrom et al., Reference Bergstrom, Nyman, Widgren, Johnston, Gronlund-Andersson and Ransjo2012b).
For example, incidence of MRSA in the Netherlands is extremely low (0.7% in 2008) and this is attributed to effective implementation of the national MRSA guidelines in every healthcare setting for more than 20 years. These guidelines recommend prudent and restrictive use of antibiotics and an infection prevention program called ‘search and destroy.’ All patients and healthcare workers are screened for MRSA and if tests are positive they are isolated and treated to eliminate MRSA. Policies for cleaning and disinfection are also strictly followed (Van Knippenberg-Gordebeke, Reference Van Knippenberg-Gordebeke2010). A cost–benefit analysis in a Dutch hospital concluded that this program prevented 36 cases of bacteremia annually and 10 deaths and saved >200,000 euros per year (Van Rijen and Kluytmans, Reference Van Rijen and Kluytmans2009).
Similar programs have been implemented in other European countries with similar success in preventing HA infections. However, there has been discussion recently regarding the merits of adopting such a strict control program. The ‘search and destroy’ programs require significant resources to test all patients, keep them in isolation until test results come back, and continue to isolate them if tests are positive (Butterly et al., Reference Butterly, Schmidt and Wiener-Kronish2010). Some have questioned the cost effectiveness of testing all incoming patients and instead recommend testing only certain patient populations, such as those entering intensive care units or those scheduled for surgery. A recent model analyzed the estimated costs of implementing screening and isolation programs at U.S. hospitals, savings in terms of the number of infections prevented, and additional costs incurred by patients with MRSA. Costs for screening all incoming patients and for using PCR methods exceeded those of screening selected high-risk patients, using chromogenic media. This analysis indicated that at hospitals with a relatively high baseline of MRSA infections, all strategies were cost effective, but screening selected patients with PCR had the greatest net benefit (Hubben et al., Reference Hubben, Bootsma, Luteijn, Glynn, Bishai, Bonten and Postma2011).
Another strategy to reduce MRSA in healthcare facilities is the reduction of antibiotic use to lower selection pressure for MRSA. This may be a useful procedure as a decrease in antibiotic use in a Taiwanese hospital from 2004 to 2009 was significantly correlated with fewer MRSA infections in patients (Lee et al., Reference Lee, Tsao, Lin, Huang, Lee and Hsueh2010). Strengthening general infection control procedures throughout health care facilities can reduce all nosocomial infections. However, because increasing numbers of MRSA infections are acquired outside of health care settings, an effective MRSA control program will need to address prevention of infections arising in the community as well as methods to control infections in hospitals.
A multifaceted infection control system implemented in a Veterans Affairs hospital, emphasizing active surveillance culturing for MRSA and behavior change strategies to encourage workers to adhere to infection control precautions, resulted in sustained decreases in MRSA colonization and infection (Ellingson et al., Reference Ellingson, Muder, Jain, Kleinbaum, Feng, Cunningham, Squier, Lloyd, Edwards, Gebski and Jernigan2011). Effective implementation of infection control and antibiotic stewardship programs in hospitals requires behavioral changes in supervisors and health care workers. Therefore, attention must be paid to local/national cultural values in order to improve compliance (Borg et al., Reference Borg, Camilleri and Waisfisz2012). Prevalence of MRSA in the environment of different nursing homes varies substantially and investigations demonstrated that frequency of cleaning common rooms and length of time spent cleaning residents’ rooms were important factors affecting prevalence (Murphy et al., Reference Murphy, Eells, Quan, Kim, Peterson, Miller and Huang2012).
Sanitizers and surface treatments
Several sanitizers can be used to control methicillin-resistant staphylococci. Use of alcohol hand rubs has been significantly correlated with decreasing rates of MRSA infections (Sakamoto et al., Reference Sakamoto, Yamada, Suzuki, Sugiura and Tokuda2010; Sroka et al., Reference Sroka, Gastmeier and Meyer2010). Chlorhexidine is also an effective antiseptic, but some strains of MRSA have developed resistance to it (Batra et al., Reference Batra, Cooper, Whiteley, Patel, Wyncoll and Edgeworth2010; Zhang et al., Reference Zhang, O'Dononghue and Boost2012). Use of chlorhexidine gluconate impregnated washcloths by marine recruits decreased acquisition of CA-MRSA (Whitman et al., Reference Whitman, Schlett, Grandits, Millar, Mende, Hospenthal, Murray and Tribble2012). Mist application of a mixture of chlorine dioxide and a quaternary ammonium compound was found to inactivate MRSA on several environmental surfaces (Callahan et al., Reference Callahan, Beck, Duffield, Shin and Meschke2010). Non-thermal plasmas were shown to be effective in inactivating both planktonic- and biofilm-associated MRSA (Burts et al., Reference Burts, Alexeff, Meek and McCullers2009; Joshi et al., Reference Joshi, Paff, Friedman, Fridman, Fridman and Brooks2010). Swimming pools containing chlorinated water, biguanide-treated water, or salt water did not permit survival of MRSA (Gregg and Lacroix, Reference Gregg and Lacroix2010).
Certain chemicals added to surface materials exert toxic effects on bacteria. Copper is a known bacteriocide and copper-based biocide solutions (Luna et al., Reference Luna, Hall, King and Cannons2010) and copper incorporated into surfaces (Weaver et al., Reference Weaver, Noyce, Michels and Keevil2010) both effectively killed MRSA. Silver is also bactericidal and a TiO2–Ag composite completely inactivated MRSA within 24 h (Necula et al., Reference Necula, Fratila-Apachitei, Zaat, Apachitei and Duszczyk2009). Data comparing bactericidal effects of Cu- and Ag-containing materials, under different conditions of temperature and humidity, indicate that Cu may be more effective in indoor environments (Michels et al., Reference Michels, Noyce and Keevil2009). A nanocomposite film incorporating a cell wall degrading enzyme has been developed and found to be effective in killing MRSA. This may prove useful in hospitals and other areas where infection control is critical (Pangule et al., Reference Pangule, Brooks, Dinu, Bale, Salmon, Zhu, Metzger, Kane and Dordick2010).
Since swine are colonized or infected with MRSA fairly frequently, strategies are being devised to protect those with occupational exposure to pigs and other livestock. A preliminary study tested a program based on improving employee hand hygiene and showering, treating wounds, providing clean boots and coveralls, and cleaning and disinfecting showers and eating areas. Results of the small study were inconclusive, but these practices are likely to be effective if used correctly and consistently and a larger trial has been recommended (Larson et al., Reference Larson, Wagstrom, Donham, Harper, Hanson, Male and Smith2012).
Prevention of food-borne intoxication
Preventing staphylococcal intoxication by MRSA strains requires the same precautions as for MSSA strains. Efforts should be made to prevent contamination throughout the food production, processing and preparation chain. Adherence to good hygiene practices during slaughter and processing of livestock can significantly reduce contamination of meat and meat products. Workers have been implicated in many outbreaks of foodborne disease. They may shed bacteria and viruses, even when asymptomatic and several weeks after they have recovered from an illness. Improved hygiene precautions, consistently practiced by persons in food preparation and processing would significantly improve safety of foods (Todd et al., Reference Todd, Greig, Bartleson and Michaels2008; Bystron et al., Reference Bystron, Podkowik, Korzekwa, Lis, Molenda and Bania2010). Foods also must be cooked properly and refrigerated or kept hot until consumption. A recent analysis of growth requirements noted that S. aureus can grow at a water activity of 0.867 and at temperatures as low as 8 °C (Valero et al., Reference Valero, Pérez-Rodríguez, Carrasco, Fuentes-Alventosa, García-Gimeno and Zurera2009). This requires education not only of workers in the food industry broadly but also of the general public. Many outbreaks of staphylococcal food intoxication result from allowing foods to remain at ambient temperature, for example at picnics or large banquets, thus giving S. aureus time to grow and produce enterotoxins.
Acknowledgements
The American Meat Institute Foundation and the Food Research Institute at the University of Wisconsin, Madison supported this research.