“A meal without grease is not a real meal.”
—Mathieu Mestokosho, Innu hunter, in Serge Bouchard, Caribou Hunter: A Song of a Vanished Innu Life, 2006 (author's translation)Animal fat is a scarce resource highly valued by human foragers. In wild terrestrial ungulates, this resource is primarily found directly under the skin, around organs, and within skeletal elements (Bourre Reference Bourre1991; Pond Reference Pond and Symonds2017). Among these anatomical sites, skeletal fat is critical because it shows relatively small seasonal variations in comparison to most other fat depots (Malet Reference Malet, Beyries and Vaté2007). Moreover, skeletal fat may remain relatively unaffected even in animals that have metabolized their other main reservoirs of fat, with distal limb elements retaining fat longer than proximal elements (Dauphiné Reference Dauphiné1976; Raglus et al. Reference Raglus, De Groef, Rochfort, Rawlin and McCowan2019; see Outram [Reference Outram and Rowley-Conwy2000] for an archaeological application of this observation). These features largely explain why skeletal fat is universally exploited by human foragers who have limited access to commercial food (Speth and Spielmann Reference Speth and Spielmann1983). Given the crucial role that this resource plays in the subsistence and worldviews of foraging societies, better understanding the contexts that led to the emergence of skeletal fat procurement is an important goal in prehistoric research.
In the literature, a distinction is generally made between two forms of skeletal fat. One form consists of “marrow”—the fatty substance stored in the central hollow cavity of certain elements such as the long bones of mammals—whereas the other is made of the “grease” found in the cancellous (spongy) portion of bones. From a biochemical perspective, however, this distinction is largely arbitrary, as both substances are compositionally similar when sampled in adjacent loci of the same element (Emerson Reference Emerson1990). Although only a few blows are needed for successful marrow extraction, ethnographic and experimental sources indicate that the comminution of the spongy ends of the same bone is a strenuous and time-consuming task (Bacon and Vincent Reference Bacon and Vincent1979; Baker Reference Baker2009; Binford Reference Binford1978; Brink Reference Brink2008; Church and Lyman Reference Church and Lyman2003; Costamagno and David Reference Costamagno and David2009; Janzen et al. Reference Janzen, Reid, Vasquez and Gifford-Gonzalez2014; Karlin and Tchesnokov Reference Karlin, Tchesnokov, Beyries and Vaté2007; Kulchyski et al. Reference Kulchyski, McCaskill and Newhouse1999; Leacock and Rothschild Reference Leacock and Rothschild1994; Michelsen Reference Michelsen1967; Pasda and Odgaard Reference Pasda and Odgaard2011; Sverdrup Reference Sverdrup1939; Uhlenbeck and Tatsey Reference Uhlenbeck and Tatsey1912). In traditional settings, long-bone ends and other spongy elements are generally crushed with simple tools, typically a hammer stone (or an axe when industrial goods became widely available) and an anvil stone.
Although not as physically demanding, rendering grease from bones—a practice that consists of boiling pounded bone fragments to dislodge their fat content—is laborious because it necessitates nearly constant tending of the fire and water temperature. Moreover, the congealed grease floating on top of the water must periodically be scooped out, often over a period of several hours, if not days (Abe Reference Abe2005; Burch Reference Burch1998; Leechman Reference Leechman1951; Lupo and Schmitt Reference Lupo and Schmitt1997; Wilson Reference Wilson1924). As performed traditionally, bone grease manufacture entails significant costs ensuing from the procurement of containers, water, fuel, and, when relevant, suitable stones (e.g., hammer, anvil, boiling stones) or substitutes (e.g., baked clay objects used for boiling) because these resources are frequently limited in abundance at the locus of production (Binford Reference Binford1978; Brink and Dawe Reference Brink and Dawe2003; Janzen et al. Reference Janzen, Reid, Vasquez and Gifford-Gonzalez2014; Murdoch Reference Murdoch1892).
Given the substantial costs for small yields, the tool-assisted extraction of fat from spongy bone may yield critical insights about the evolution of intensification practices (e.g., Manne Reference Manne2014; Manne et al. Reference Manne, Stiner, Bicho and Bicho2006; Nakazawa et al. Reference Nakazawa, Straus, González-Morales, Solana and Saiz2009; Outram Reference Outram2001; Wolverton et al. Reference Wolverton, Nagaoka, Densmore and Fullerton2008). However, because it is difficult to identify this practice in faunal assemblages, the timing of its emergence in the archaeological record remains controversial (Castel et al. Reference Castel, Discamps, Soulier, Sandgathe, Dibble, McPherron, Goldberg and Turq2017; Costamagno Reference Costamagno, Speth and Clark2013; Delpech and Rigaud Reference Delpech, Rigaud and Camps-Fabrer1974; Manne et al. Reference Manne, Stiner, Bicho and Bicho2006; Marean Reference Marean, d'Errico, Backwell and Tobias2005; Nakazawa et al. Reference Nakazawa, Straus, González-Morales, Solana and Saiz2009). The goal of the present article is to revisit the archaeological correlates for bone grease rendering using a new experiment that involved severe fragmentation of long-bone ends. The experimental observations highlight several trends with regard to the identification and size distribution of bone fragments that should aid in constructing improved models of skeletal fat processing in archaeological contexts.
Tool-Assisted Extraction of Skeletal Fats
Archaeologists frequently focus on the tool-assisted extraction of fats deposited within the skeleton of terrestrial herbivores because this practice sets humans apart from most other species, which use their teeth and/or digestive enzymes to dislodge this valuable resource. However, distinguishing tool-assisted fat extraction from other agents of bone fragmentation such as carnivores, trampling, fire, and weathering poses an interpretive challenge as a result of sometimes significant overlap in their respective archaeological signatures (Bovy et al. Reference Bovy, Etnier, Butler, Campbell and Shaw2019; Castel et al. Reference Castel, Discamps, Soulier, Sandgathe, Dibble, McPherron, Goldberg and Turq2017; Costamagno et al. Reference Costamagno, Théry-Parisot, Brugal, Guibert, Mulville and Outram2005; Heinrich Reference Heinrich2014; Lam et al. Reference Lam, Pearson, Marean and Chen2003; Manne et al. Reference Manne, Stiner, Bicho and Bicho2006; Morin and Soulier Reference Morin and Soulier2017; Munro and Bar-Oz Reference Munro and Bar-Oz2005; Outram Reference Outram2001; Pavao-Zuckerman Reference Pavao-Zuckerman2011; Sunseri Reference Sunseri2015). The interpretation of faunal assemblages is further complicated by the fact that these agents of fragmentation are not mutually exclusive, and they can collectively modify markers of tool-assisted extraction of fats.
Early studies aimed at identifying bone grease rendering have pointed out that the practice produces a high proportion of very small fragments (Delpech and Rigaud Reference Delpech, Rigaud and Camps-Fabrer1974; Kehoe Reference Kehoe1967; Leechman Reference Leechman1951; Rood Reference Rood, Metcalf and Black1991). Indeed, although experiments have shown that coarse fragments can be used to obtain grease (Church and Lyman Reference Church and Lyman2003), boiling smaller specimens is advantageous because it decreases labor and fuel costs (Janzen et al. Reference Janzen, Reid, Vasquez and Gifford-Gonzalez2014). In addition to average fragment size, other criteria such as the relative frequency of green bone fractures, whole long bones, spongy bone fragments, whole articular ends, and burned specimens in an assemblage may inform the identification of skeletal fat extraction (Baker Reference Baker2009; Costamagno Reference Costamagno, Speth and Clark2013; Munro and Bar-Oz Reference Munro and Bar-Oz2005; Outram Reference Outram2001, Reference Outram, Mulville and Outram2005; Wolverton et al. Reference Wolverton, Nagaoka, Densmore and Fullerton2008). Skeletal abundances may also show a positive relationship with grease utility and a negative relationship with bone density (Binford Reference Binford1978; Brink Reference Brink1997; Emerson Reference Emerson and Hudson1993; Morin Reference Morin2007, Reference Morin2010; Sunseri Reference Sunseri2015). Other potential lines of evidence include the frequent occurrence of fire-cracked rocks and pitted stone anvils at a site as well as the spatial distribution and composition of hearths and bone dumps (Binford Reference Binford1978; Brink and Dawe Reference Brink and Dawe2003; Manne et al. Reference Manne, Stiner, Bicho and Bicho2006; Munro and Bar-Oz Reference Munro and Bar-Oz2005; Pavao-Zuckerman Reference Pavao-Zuckerman2011; Vehik Reference Vehik1977). From this perspective, in situ archaeological features possibly indicative of bone grease rendering (Chomko and Gilbert Reference Chomko and Gilbert1991; Karr et al. Reference Karr, Short, Adrien Hannus and Outram2015) may provide additional support for the identification of this activity at a site.
The above lines of evidence are of limited use, however, because they can occur in a wide range of behavioral contexts. For instance, fire-cracked rocks may accumulate at a site as a result of cooking and smoking activities, whereas pitted anvils could have been used for cracking nuts, grinding ochre, or processing shellfish, among other tasks. Likewise, the interpretation of fragment-size distribution can be confounded by various syndepositional (e.g., use of bone as fuel, trampling) and postdepositional (e.g., sediment compaction, density-mediated attrition) processes (Costamagno Reference Costamagno, Speth and Clark2013; Heinrich Reference Heinrich2014; Morin Reference Morin2010; Munro and Bar-Oz Reference Munro and Bar-Oz2005; Outram Reference Outram2001; Prince Reference Prince2007; Théry-Parisot et al. Reference Théry-Parisot, Costamagno, Brugal, Fosse, Guilbert, Mulville and Outram2005).
In an attempt to improve our identification of bone grease rendering in archaeological contexts, Morin and Soulier (Reference Morin and Soulier2017) presented new, bone-specific criteria that focus on the morphology of bone fragments and the distribution of crushing and tear marks on particular anatomical features. Lithic micro-inclusions—defined as microscopic stone fragments from the hammer or anvil that become embedded in the bone matrix during the comminution process—provide an additional line of evidence, as they have been observed at high frequencies in experimental samples associated with bone grease rendering. Although useful, the published experimental data only addressed faunal signatures in moderately comminuted assemblages. To expand our knowledge on variability in bone grease processing, results from a new grease-rendering experiment focused on the production of very small fragments are presented below.
Materials and Methods
To better outline the archaeological signatures of bone grease rendering, the present study compares trends observed in three bone samples that differ in degree of processing (Figure 1). Two of these samples were the focus of a blind test on faunal identification that involved red deer (Cervus elaphus) long bones (Table 1). The first sample, called the MCE (for “Marrow Cracking Experiment,” n = 5,188 specimens from 162 long bones), consists of bones only fractured for marrow. In contrast, the second blind test sample, called the BGRE (for “Bone Grease Rendering Experiment,” n = 10,370 specimens from 155 long bones), involved both the marrow cracking of long bones and the comminution of articular ends. Three skilled faunal analysts with no prior knowledge of the samples were asked to produce independent NISP, MNE, and MNI counts for these two samples. Because the implications of their different counts have been analyzed elsewhere (Morin et al. Reference Morin, Ready, Boileau, Beauval and Coumont2017a, Reference Morin, Ready, Boileau, Beauval and Coumont2017b), the results of the blind test are not discussed further here. Instead, the faunal samples that formed the basis of the blind test are compared here with new experimental data to cast additional light on the archaeological identification of bone grease processing.
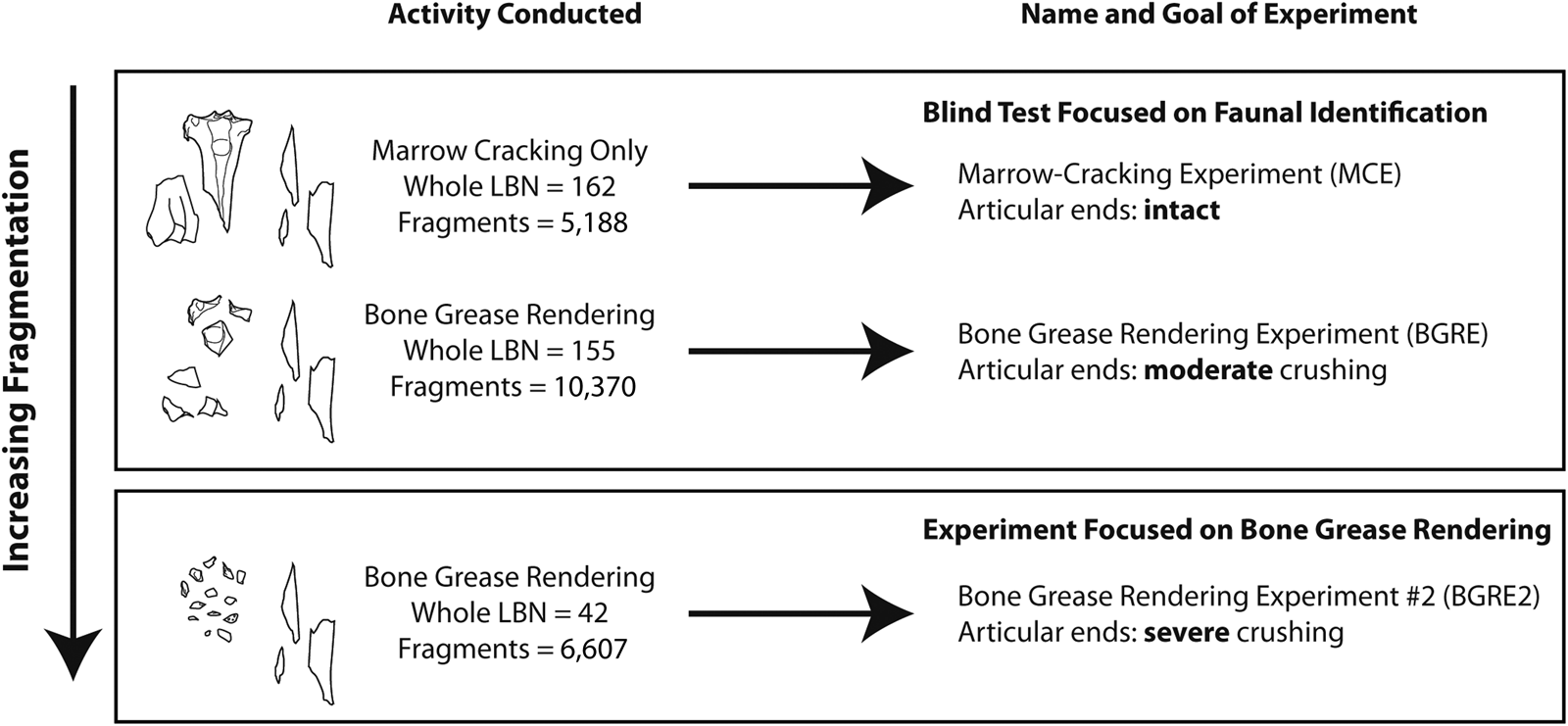
Figure 1. Experimental design for the faunal samples. Counts are for long bones only (ulna counted separately from the radius). “LBN” stands for long bones.
Table 1. Numbers of Long Bones and Long-Bone Fragments in the Blind Test Samples and in a New Experiment Focused on Bone Grease Rendering.

Notes: MCE = Marrow-Cracked Experiment. BGRE = Bone Grease Rendering Experiment. BGRE2 = Bone Grease Rendering Experiment #2. ANE = Actual Number of Elements used in the experiment. NSP = Number of Specimens, including identifiable and indeterminate fragments. NSP counts for the radius include ulna fragments. For this reason, there are no NSP counts for the ulna. Fragmentation is moderate in the BGRE and severe in the BGRE2.
All of the long bones in the blind test samples were marrow-cracked using a hornfels hammer and anvil. Although no further processing occurred in the MCE, the epiphyses from the BGRE were pounded until the fragments formed a flat “cake” with the goal of replicating traditional methods of bone grease manufacture. Once fractured, specimens were boiled, cleaned, and labeled separately according to element and experiment. Although other classes of spongy bones (e.g., vertebrae, carpals, tarsals) are known to have been exploited by recent foragers, only long-bone ends were crushed in the experiments presented here because the ethnographic record indicates that these parts were the prime targets for grease extraction (Anderson Reference Anderson1918; Binford Reference Binford1978; Comeau Reference Comeau1909; Eggermont-Molenaar Reference Eggermont-Molenaar2005; Hadleigh-West Reference Hadleigh-West1963; Irimoto Reference Irimoto1979; Nelson Reference Nelson1983; Pasda and Odgaard Reference Pasda and Odgaard2011; Rogers Reference Rogers1973; Skinner Reference Skinner1912; Wilson Reference Wilson1924; Yukon Wildlands Project 1998). Importantly, the degree of comminution is considered “moderate” in the BGRE, as suggested by comparisons with published ethnographic and ethnohistorical accounts (Morin and Soulier Reference Morin and Soulier2017:112–113, Table 6).
For this study, the MCE and BGRE material was compared with a new experimental collection hereafter referred to as the BGRE2 (for “Bone Grease Rendering Experiment #2”; Figure 1) sample. This new material (n = 6,607 specimens >1 cm from 42 long bones; Table 1) derives from an experiment conducted in May and June 2019. The main goal of this new experiment was to produce fragments that are substantially smaller than the BGRE. More specifically, the BGRE2 epiphyses were “pulverized” into small fragments, as “big as finger nails” (Leechman Reference Leechman1951:355). In practice, this means that pounding was terminated for an articular end when all cancellous fragments appeared to be no larger than approximately 1–2 cm. Given that the goal of the experiment was to extract grease, the shaft portion of the bone was avoided as much as possible during comminution.
As in blind test experiments, bones in the BGRE2 were struck with a hammer stone following the routine described in Morin and Soulier (Reference Morin and Soulier2017), with the surface opposite to the point of impact resting on the anvil. The periosteum was not scraped off. Once the bone cavity was breached, the marrow plug was removed with a metal knife and the epiphyses subsequently crushed. Long shanks were usually snapped off from the epiphyses prior to comminution, as was done by the Nunamiut of Alaska (Binford Reference Binford1978). Articular ends were pounded on the same hornfels anvil in the BGRE and BGRE2 experiments. In the BGRE2, however, a moderate-sized granite hammer (initial weight: ~1,975 g; end weight: 1,702 g) replaced the very large hornfels hammer (7,500 g) used in the BGRE, as the smaller hammer better fit ethnographic descriptions.
The BGRE2 used bones of white-tailed deer (Odocoileus virginianus) because the author no longer had access to red deer carcasses. From a taxonomic standpoint, this substitution probably has only a minor effect on the data, given that osteological differences between cervids are small. In order to compensate for the smaller body size of white-tailed deer relative to red deer, only the largest white-tailed deer bones were selected. Although ages at death are unknown, patterns of epiphyseal closure (Purdue Reference Purdue1983), season of procurement (fall), and bone size suggest that 1.5- and 2.5-year-old individuals dominate the BGRE2 sample. This age profile is slightly older than the BGRE collection (dominated by 6-month-old and 1.5-year-old individuals), which further reduced the gap in average element size between the BGRE and BGRE2. Nonetheless, because the bones used in the BGRE2 were slightly smaller than those included in the BGRE—perhaps as much as 15%–20% shorter in maximum length—the number of small fragments produced in the BGRE2 should be considered a conservative estimate.
Although fragments resulting from marrow cracking and the comminution of the epiphyses were collected together during the BGRE, these specimens were kept separate in the BGRE2 for comparison of bone-size distributions between shaft and spongy fragments. To derive bone-size distributions, all fragments were counted according to 1 cm size increments. Specimens with a maximum dimension smaller than 1 cm were excluded from the calculations due to highly variable recovery rates for this size class in archaeological excavations. Due to their great abundance, small fragments (1–2 and 2–3 cm size classes) in the BGRE were photographed and counted using the particle analysis function of ImageJ (Schneider et al. Reference Schneider, Rasband and Eliceiri2012; see Cannon [Reference Cannon2013] for an application in archaeology). The other fragments—including all of the BGRE2 specimens—were counted manually (note that both approaches produced very similar counts when we compared them). The bone counts presented below make a distinction between three types of bones. “Articular” fragments preserve any portion of an articular surface, irrespective of surface area. All other fragments were either classified as “shaft” or “spongy” bones, depending on whether the trabecula was visible on less (shaft) or greater (spongy) than 50% of the internal surface of the specimen. Counts by bone types are provided for all long bones, except for the BGRE where the radio-ulna had to be excluded due to loss or misplacement of material, a problem likely caused by the fact that the material was handled by several people for training purposes.
The BGRE and BGRE2 are used here as frameworks to assess whether widely held assumptions about bone grease rendering are consistent with experimental results. In these experiments, specimens were all tentatively identified to taxon, except for the BGRE2, in which remains were only identified to skeletal element. This change in level of identification is explained by the fact that the analyst who examined the BGRE2 fragments—the author—was aware of the monospecific nature of the sample, which would have biased the results (this is unlike the MCE and BGRE, where the three analysts who identified the fragments were participating in a blind test). In the following analyses, rank order correlations were computed using Spearman's p, whereas differences in percentages were tested after arcsine transformation of the data using Sokal and Rohlf's (Reference Sokal and Rohlf1969) t statistic. The existence of linear trends was verified using the Cochran-Armitage test (denoted χ 2trend [Cannon Reference Cannon2001]).
Results
As expected, there are marked differences in fragment size distribution between the two grease rendering experiments (Table 2; Figure 2). Whereas 33.2% of the BGRE fragments belong to the 1–2 cm size class, this proportion increases significantly to 73.0% in the BGRE2 sample (ts = 50.00, p < 0.0001). Only 3.2% (213/6,607) of the BGRE2 sample consists of spongy and articular specimens larger than 3 cm, a significantly lower value relative to the BGRE (1,311/8,472, or 15.5%, ts = 27.39, p < 0.0001). These data confirm that the two samples differ markedly with respect to overall level of fragmentation.

Figure 2. Fragment size distribution in the BGRE and BGRE2 experiments. Data from Table 2.
Table 2. Fragment Size Distribution in the BGRE and BGRE2 Samples.

Notes: The BGRE counts excludes the radio-ulna because some of the material for this bone seems to have been misplaced since the blind test was published (see text). For a breakdown by element, see Supplemental Tables 1 and 2.
a n are simple bone counts
It has frequently been argued that bone grease rendering produces a large quantity of spongy fragments (e.g., Binford Reference Binford1978; Davis and Fisher Reference Davis, Fisher, Davis and Reeves1990; Delpech and Rigaud Reference Delpech, Rigaud and Camps-Fabrer1974). Although this may well be true for severely fragmented samples, it remains to be demonstrated whether this assumption is valid at moderate levels of fragmentation and regardless of skeletal composition. The fact that spongy and articular fragments represent slightly less than half of the material (3,895/8,472, or 46.0%) in the BGRE is not entirely consistent with the view that bone grease rendering systematically produces epiphyseal-dominated samples (Table 2; Supplemental Tables 1–2). This trend contrasts with that for the BGRE2 sample, in which spongy and articular fragments account for over two-thirds of the material (4,603/6,607, or 69.7%, ts = 29.55, p < 0.0001). These results indicate that moderately fragmented samples may, assuming a randomized sample (see below), contain a higher proportion of shaft as opposed to epiphyseal specimens. In contrast, epiphyseal fragments clearly prevail in highly fragmented samples.
The above trends are for samples that comprise roughly equal proportions of all types of long bones. However, because sample composition may vary with context—depending, among other factors, on transport decisions and bone availability at the time of comminution—it is critical to explore variation in tissue representation across limb elements. According to the experimental data, the percentage of spongy and articular fragments decreases steadily distally in cervid legs, a pattern upheld irrespective of the level of fragmentation (Figure 3; percentages calculated relative to the fragment total). These linear trends are highly significant in both the BGRE (hindleg: χ 2trend 369.4, p < 0.001; foreleg: χ 2trend = 365.1, p < 0.001) and BGRE2 (hindleg: χ 2trend 189.6, p < 0.001; foreleg: χ 2trend = 79.6, p < 0.001; spongy and articular fragments vs. shaft fragments in all tests). These results indicate that, in cervids, the largest cancellous reservoirs occur in the proximal bones of the limbs.

Figure 3. Anatomical variation in the proportion of spongy and articular specimens in the BGRE and BGRE2 experiments. Percentages were calculated relative to the total number of fragments for that specific class of long bone. The data were taken from Supplemental Tables 1 and 2. Hum = Humerus, Rul = Radio-ulna, Fem = Femur, Tib = Tibia, Mt = Metatarsal, Mc = Metacarpal.
Focusing on the smallest fragments (1–2 cm)—those that most archaeologists consider typical of bone grease rendering—conveys further light on inter-element differences in epiphyseal fragment production. In the moderately fragmented BGRE sample, epiphyseal specimens attributed to this size class only account for 22.5% (229/1,018) of the metapodial fragments. This proportion is markedly higher for the femur and humerus (626/1,093, or 57.3%, ts = 16.73, p < 0.0001). These observations mean that a metapodial-dominated sample with a degree of comminution similar to the BGRE may be strongly dominated by shaft rather than epiphyseal fragments. In the severely fragmented BGRE2 sample, the proportion of epiphyseal fragments within the 1–2 cm size class is relatively high for metapodials (603/968, or 62.3%), yet significantly lower than for the femur and humerus (1,827/2,226, or 82.1%, ts = 11.65, p < 0.0001). The wide variations observed between and within these experiments emphasize the importance of controlling for level of fragmentation and skeletal representation when assessing bone processing in an archaeological assemblage.
Data collected during the BGRE2 experiment indicate that the type of skeletal element has a strong impact on processing per se. This is because some elements proved far more difficult to break (e.g., tibia, femur) than others (e.g., metapodials), an inference confirmed by wide differences in the number of delivered blows (Figure 4; Supplemental Table 3). Moreover, blow counts highlight dramatic contrasts in terms of energy allocation between marrow cracking (mean: 6.8, including shank removal) and epiphysis pounding (mean: 166.3). These two activities also differ qualitatively: most marrow-cracking routines require precision rather than power, whereas both factors are important when crushing epiphyses.

Figure 4. Number of blows delivered during marrow extraction and epiphysis comminution in the BGRE2 experiment. Hum = Humerus, Rul = Radio-ulna, Fem = Femur, Tib = Tibia, Mt = Metatarsal, Mc = Metacarpal.
Analytical Absence of Articular Ends
The notion that articular ends and other spongy elements become unidentifiable during the comminution process has been emphasized by several authors (e.g., Bethke Reference Bethke, Zedeño, Jones and Pailes2018:887; Davis and Fisher Reference Davis, Fisher, Davis and Reeves1990:264; Pillaert Reference Pillaert1969:101; Prince Reference Prince2007:18; Rood Reference Rood, Metcalf and Black1991:175; Vehik Reference Vehik1977:172; White Reference White1954:256). Perhaps this view is best expressed in Binford's review of Nunamiut bone processing:
In the case of bone grease and bone juice manufacture, there is an accompanying destruction of parts beyond recognition. This activity clearly can distort the picture remaining for the archaeologist regarding the relative frequencies of faunal elements originally present on the site [Binford Reference Binford1978:463].
This statement, however, partly conflicts with identification data provided by the same author (Binford Reference Binford1978:165, Table 4.8) for pot contents associated with bone juice preparation. Therefore, because the notion that parts are destroyed “beyond recognition” during bone grease extraction may simply be a figure of speech, experimental data convey useful control information on this issue.
Considering the entire BGRE assemblage, the average proportion of long-bone specimens that the blind test participants identified as articular ends increases from 7.8% in the marrow-cracked sample (MCE) to 15.9% in the moderately fragmented BGRE sample (Supplemental Table 4). This significant increase (ts = 14.38, p < 0.0001) means that articular-end comminution produced many epiphyseal specimens identifiable to taxon in the BGRE. In contrast, the proportion of epiphyseal specimens considered identifiable is very low in the more severely fragmented BGRE2 collection (162/6,607, or 2.5%, ts = 30.54, p < 0.0001), in spite of the fact that the specimens were only identified to element and not to taxon (see Materials and Methods). The more severe comminution in the BGRE2 led to a marked decline in the identification of epiphyseal specimens.
These percentages were calculated using whole samples. Different patterns emerge when comparisons focus exclusively on identified remains. In the BGRE2 collection, epiphyseal specimens account for nearly half of the identified sample (162/369, or 43.9%). A similar proportion is seen in the BGRE collection (1,330/2,826, or 47.1%, average for three faunal analysts; Supplemental Table 5). Therefore, although the proportion of identified epiphyseal remains is low relative to the total sample in the BGRE and BGRE2 assemblages, epiphyseal specimens nonetheless account for almost half of the identified specimens.
Overall, the percentage of identified epiphyseal specimens initially increases before falling as fragmentation intensifies (Figure 5a). This trend fits the expected behavior for the relationship between NISP and fragmentation (Cannon Reference Cannon2013; Marshall and Pilgram Reference Marshall and Pilgram1993). As shown in Figure 5b, slight inflections in the curves of identification for the BGRE2 sample suggest that 4–5 cm is somewhat of a threshold with respect to the identification of shaft and epiphyseal specimens.

Figure 5. Relationship between representation and fragmentation: (a) percentage of articular ends relative to the total sample in the three experimental samples (see Supplemental Table 4); (b) the proportion of identified shaft and epiphyseal specimens according to fragment size classes in the BGRE2 sample (values calculated relative to the total shaft or epiphyseal sample for a given size class).
Evolution of Fragment Morphology and Forms of Damage
Several criteria that focus on morphology and types of damage have been published for the BGRE (Figures 6 and 7). As noted in the study of the BGRE material (Morin and Soulier Reference Morin and Soulier2017), however, several of these criteria—particularly those based on shape—are size dependent, an intuition confirmed by the BGRE2 sample. Eleven out of the 28 morphological criteria (39.3%) observed in the BGRE sample were not documented in the BGRE2. Yet, it is encouraging to note that among the 17 criteria (60.7%) that were positively identified in the BGRE2, 11 of these (64.7%) are represented by an NISP ≥ 5 (Table 3). Within this sample, bayonets (M1-Mc and M1-Mt) and half-moons (M2-Mc and M2-Mt) stand out because they are very common in both the BGRE and BGRE2, although the fragments tend to be smaller and slightly different in appearance in the BGRE2 (Supplemental Texts 1 and 2).
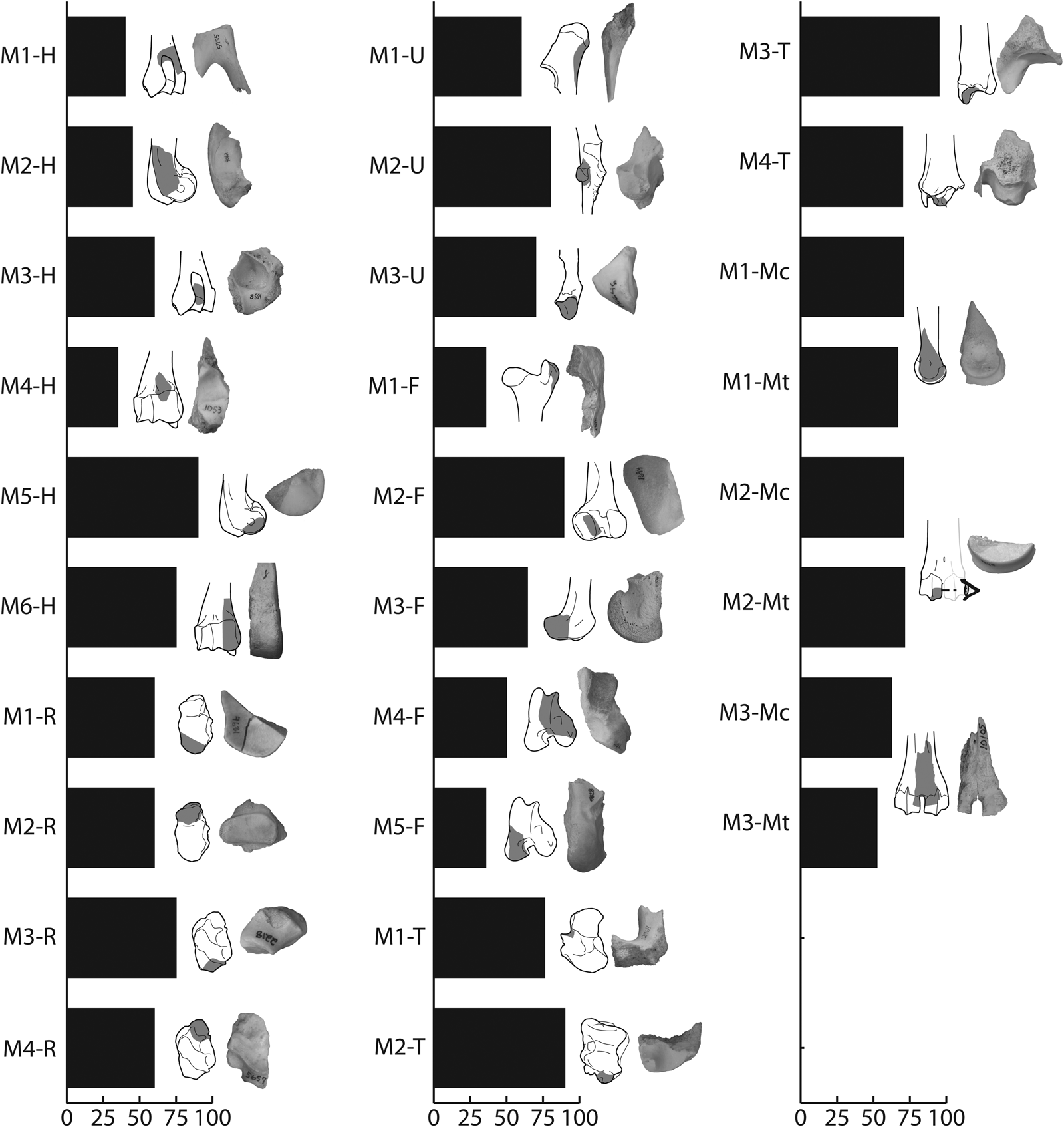
Figure 6. Morphological criteria and their occurrence in the BGRE sample. The shaded areas indicate the position of the fragments on the elements. Percentages were obtained by dividing the MNE by the known number of elements × 100. Data from Morin and Soulier (Reference Morin and Soulier2017:109).

Figure 7. Types of crushing marks and tear marks and their occurrence in the BGRE sample. Zones of crushing marks are indicated by small x's, whereas tear marks were observed in zones marked by dark shaded areas (the pale shaded areas indicate the position of a typical fragment associated with these marks). Percentages were obtained by dividing the MNE by the known number of elements × 100. Data from Morin and Soulier (Reference Morin and Soulier2017:109).
Table 3. Criteria Documented in the BGRE2 Sample.

Note: New criteria are identified by an asterisk. The other criteria are those defined by Morin and Soulier (Reference Morin and Soulier2017).
The BGRE2 permitted the identification of 10 new criteria (Figure 8; Supplemental Texts 1 and 2). By far, the most common morphologies include subconical fragments from the head and condylar portions of the humerus and femur (M7-H, NISP:56; M7-F, NISP:60), and to a lesser extent, subtriangular fragments from the proximal epiphyses of the metapodials (M4-Mc, NISP:17; M4-Mt, NISP:22; Table 3). Moreover, the analysis of the mostly unfused metapodials permitted the identification of a large number of isolated cones deriving from the metaphysis (M5-Mc, NISP:16; M5-Mt, NISP:20). Because isolated cones were relatively easy to identify, they may prove to be particularly useful criteria in assemblages dominated by juveniles and young adults.
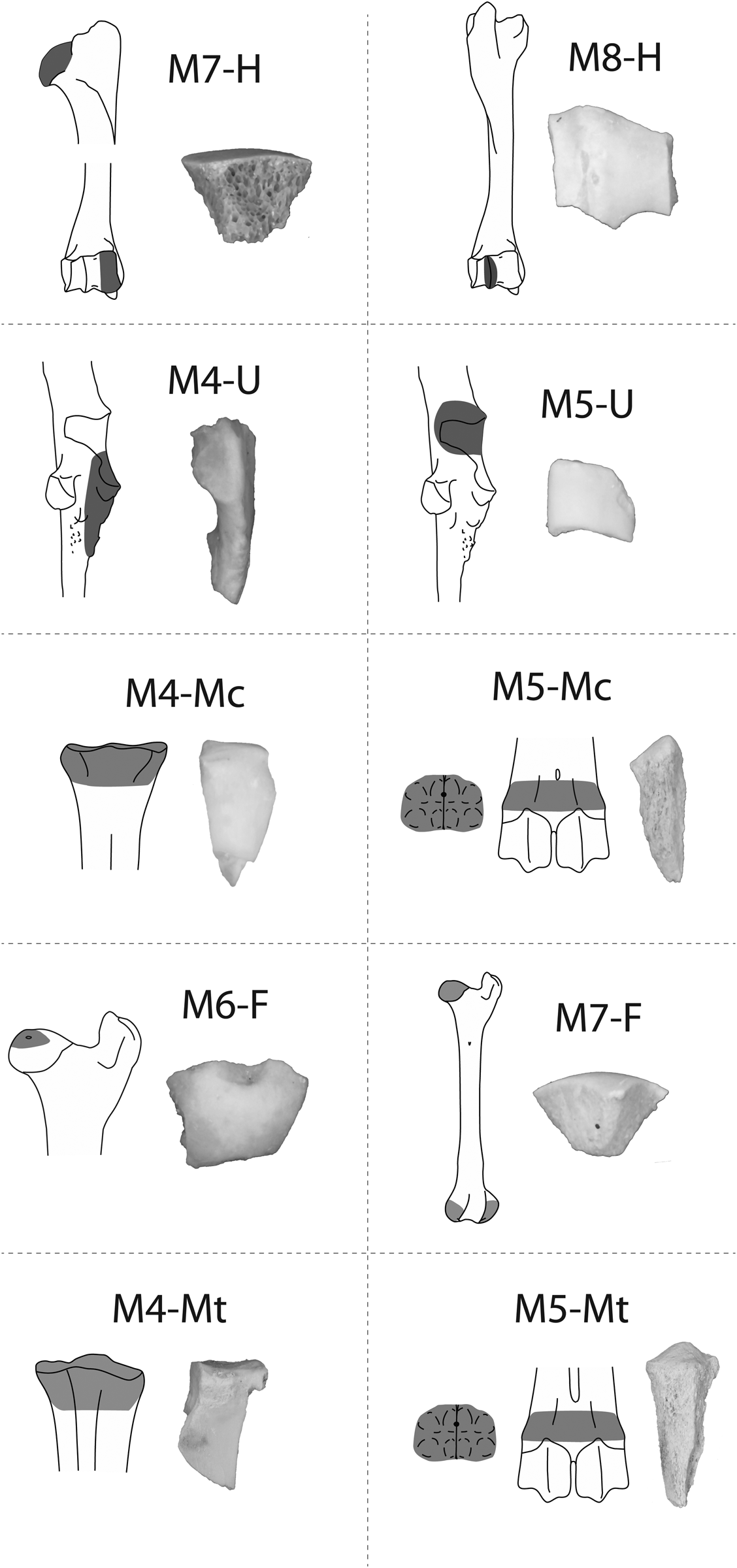
Figure 8. The new morphological criteria identified in the BGRE2 experiment.
Several forms of damage were recorded in the BGRE, including crushing marks and tear marks (the latter are slivers of bones that are detached when bone “peels” off from an articular surface; Figure 9). Counterintuitively, crushing marks appear to be less common in the extensively fragmented BGRE2 relative to the BGRE assemblage, perhaps because identification was made difficult by the small size of the fragments (Table 3). In the BRGE2 assemblage, tear marks were most commonly observed on the ulna and metapodials. These marks are important, as they appear to be rare in contexts other than those involving dynamic loading with tools or bending force applied on green bones (Morin and Soulier Reference Morin and Soulier2017). Unlike the preceding forms of damage, lithic micro-inclusions are ubiquitous in the BGRE2 collection (28/30, or 93.3%, of the specimens, random sample drawn from all six categories of long bones). Although this proportion is greater than in the BGRE (149/180, or 82.8%), the difference is not significantly different (ts = 1.68, p = 0.09). No previously undocumented forms of damage were observed in the new experimental sample.

Figure 9. An example of tearing (or peeling) observed in the BGRE2 experiment. The black dots show a tearing zone. (Color online)
The Problem of Discard Behavior
The above analyses assume that the residues of marrow-cracking and grease-rendering activities are discarded together or are likely to become mixed through the action of various processes. The data presented in Table 2—which combines counts for both marrow and grease extraction—can be used as an interpretive framework in these circumstances. In other contexts, however, intervals of days, weeks, or even months may have separated these activities, especially in cool environments where fat preserves longer. These time intervals increase the likelihood that residues of marrow procurement and bone grease manufacture were discarded in separate locations. Abandoning faunal debris in distinct zones of a site may be deliberate because it facilitates the retrieval of grease-rich bones in periods of food insecurity (Binford Reference Binford1978).
The material correlates of bone grease manufacture can be conspicuous when debris is discarded in isolation from other types of residues. Among the Nunamiut, these remains generally take the form of “a large pile of pulverized bone approaching the appearance of bone meal. This is generally a dump to one side of a substantial hearth containing large quantities of ash” (Binford Reference Binford1978:159). Similar patches of bone grease rendering residues have been documented among Native groups in Siberia (Karlin and Tchesnokov Reference Karlin, Tchesnokov, Beyries and Vaté2007:319–320; Vaté and Beyries Reference Vaté, Beyries, Beyries and Vaté2007:414). Data presented in Supplemental Table 6 simulate a situation where remains of marrow cracking and bone grease rendering are dumped in separate locations. The counts provided in this table correspond to the total BGRE2 assemblage separated into two subsamples as a function of activity: (1) the fragments produced while marrow cracking, and (2) those generated while comminuting the epiphyses. As expected, the proportion of spongy and articular fragments is very high in the bone grease component (4,539/5,450, or 83.3%) of the BGRE2, which stands in sharp contrast to their low representation in the marrow-cracked fraction (64/1,157, or 5.5%, ts = 56.41, p < 0.0001). The bone-size distributions are also strikingly different: only 5.5% (301/5,450) of the specimens are larger than 3 cm in the bone grease component of the BGRE2 compared to 37.8% in the marrow-cracked fraction (437/1,157, ts = 26.28, p < 0.0001). These dramatic differences imply that marrow cracking can easily be distinguished from bone grease manufacture when their respective debris is discarded in distinct areas of a site.
Discussion
Excavated assemblages remain to be analyzed using the above criteria. Nonetheless, it is possible to explore the archaeological implications of the experimental findings. Although the specimens that result from bone grease rendering have frequently been described as being smashed “beyond recognition,” this is clearly an overstatement. The experimental dataset presented here shows that even in conditions of severe fragmentation, some epiphyseal specimens remain identifiable. In fact, these specimens are nearly as abundant as shaft fragments in the sample of remains that were identified to skeletal element.
Additional experimental work needs to be carried out on the relationship between patterns of fragmentation and processing routines. The protocol followed here assumes that epiphysis comminution occurs after marrow extraction. Marrow removal may occur after the comminution of the epiphyses, however, as documented in some Innu groups (Henriksen Reference Henriksen1973; Lamothe Reference Lamothe1973). In fact, holding an uncracked bone by the shaft facilitates the comminution of the articular ends, which reduces energy costs. The resulting bone cylinders can subsequently be smashed open for marrow. Other approaches have also been documented. At residential camps, the Nunamiut observed by Binford (Reference Binford1981:159–163) produced bone cylinders by successively impacting the proximal and distal shaft regions of the long bone on a handheld tabular stone. Unlike the Innu, the Nunamiut pounded the epiphyses after the marrow was pushed out of the cylinders. How these variants affect epiphysis reduction remains to be ascertained.
The impact of taxonomic composition on fragmentation patterns also deserves further examination since most of the experimental and ethnoarchaeological data on bone grease manufacture were derived from cervid samples. Although there is no comparable information for larger taxa, such as equids or large bovines, it is useful to consider possible faunal patterns for these species, given that they often occur at high frequencies in archaeological assemblages. If we assume that a person comminuting bones to extract grease is concerned with obtaining specimens of a specific modal size—1 to 2 cm, for instance—then, a logical implication is that the rate of identification should decline in samples dominated by large taxa because the fragments would less likely include a diagnostic zone. In addition, because cervids have cancellous reservoirs that appear to be proportionally smaller relative to larger ungulates, the percentages of spongy fragments reported here are probably conservative estimates for these animals. Both issues need to be verified experimentally.
While removing the residual boiled soft tissue from the BGRE and BGRE2 fragments, the cleaning team noted an abundance of loose stone fragments in the sieves. These small stone fragments—most ranging between 0.1 and 10 mm in maximum length—are shatters that emanate from the hammer and anvil. The high frequency of micro-inclusions in the BGRE2 sample fits well with this observation. Consequently, the co-occurrence of spongy fragments, micro-inclusions, and stone shatters may represent an additional piece of evidence supporting bone grease manufacture at a site, once background contamination is controlled for. However, because small stone shatters can, among other possibilities, adhere to meat while butchering on a rocky substrate or as wind blows on drying meat or be produced while grinding plants or bones for direct consumption (Abbie Reference Abbie1970; Cleland Reference Cleland1939; Emmons Reference Emmons1991; Michelsen Reference Michelsen1967; Turner Reference Turner1894), this line of evidence must be examined carefully. The available data suggest that this background contamination should be associated with particles that are smaller—perhaps of “dust” size—than many of the stone shatters produced during bone grease manufacture.
This argument brings us to the problem of recovery methods. Bone grease rendering produces many epiphyseal fragments of very small size. The use of large mesh sizes during excavations may selectively remove many of these specimens from a sample. This issue is particularly critical in areas such as North America, where 6.4 mm (or ¼ in.) sieves are still regularly used in excavations. In fact, these mesh sizes are probably too coarse for obtaining reliable fragment size distributions and for deriving robust interpretations about cooking methods. As it has been pointed out concerning the recovery of small taxa such as fish and rodents (e.g., Shaffer and Sanchez Reference Shaffer and Sanchez1994; Val and Mallye Reference Val and Mallye2011), the use of finer mesh sieves (no larger than 2 mm) is strongly recommended for a proper study of skeletal fat processing.
A related problem concerns the impact of spatial patterns on the representativity of archaeological samples. This problem is most acute when the number of units that were opened during an excavation is very small (e.g., a few square meters). In these contexts, the chance of sampling discard zones that may contain an abundance of cancellous fragments is severely reduced. The fact that spatial patterning in the distribution of cancellous versus shaft fragments has been identified in some prehistoric settings (e.g., Fladerer et al. Reference Fladerer, Salcher-Jedrasiak and Händel2014; Karr et al. Reference Karr, Short, Adrien Hannus and Outram2015) emphasizes the importance of considering surface area as a limiting factor when interpreting skeletal fat processing at a site.
Conclusion
The experiment presented here permitted the identification of several new criteria that can be used to build more secure archaeological inferences about bone grease manufacture. The experimental results also challenge the widely held belief that skeletal elements are destroyed “beyond recognition” during bone grease extraction. Despite a low identification rate, epiphyseal fragments were almost as common as shaft specimens in the sample of identified remains. Moreover, patterns of skeletal representation were shown to be critical with respect to the production of cancellous bone fragments, given that proximal long bones contain larger reservoirs of grease than distal ones. The implication is that bone grease rendering samples may comprise a high proportion of spongy remains when dominated by proximal long bones but a low proportion of the same remains when dominated by metapodials. Discard behavior, processing traditions, and the spatial distribution of processing activities were emphasized as other key factors of variation. Furthermore, the experimental data indicate that, when dumped in separate locations, debris resulting from marrow extraction should easily be distinguished from that associated with bone grease rendering—assuming minimal postdepositional disturbance.
On a broader scale, the dramatic differences in blow counts recorded in the experiment between marrow extraction and articular-end comminution provide ample support for the notion that this last activity is associated with low returns. Because bone grease processing has important ramifications for our understanding of the evolution of foraging behavior and cooking technologies, further efforts are needed to identify its occurrence in the archaeological record more securely. Recovery methods that focus on the collection of very small finds emerge as especially crucial in this regard.
Acknowledgments
The author would like to thank Alison MacMillan for her valuable assistance during the BGRE2 experiment. Kristine Bovy, Marie-Cécile Soulier, and three anonymous reviewers made insightful suggestions that helped improve the manuscript.
Data Availability Statement
No original data were presented in this article.
Supplemental Materials
For supplementary material accompanying this article, visit https://doi.org/10.1017/aaq.2020.29.
Supplemental Table 1. Fragment size distribution in the BGRE by skeletal element.
Supplemental Table 2. Fragment size distribution in the BGRE2 by skeletal element.
Supplemental Table 3. Number of blows delivered during the BGRE2 experiment separated by activity.
Supplemental Table 4. Percentage of epiphyseal fragments that were identified by three subjects in the MCE and BGRE samples.
Supplemental Table 5. Percentage of epiphyseal fragments that were identified by three subjects calculated relative to all identified specimens.
Supplemental Table 6. Size distribution of specimens for the BGRE2 separated by activity.
Supplemental Text 1. Ten new morphological criteria identified in the present study.
Supplemental Text 2. Illustrations and photos for the morphological criteria identified in the present study.