Research into early lithic assemblages in North America shows that prismatic blades (hereafter referred to as blades) were an essential part of Clovis lithic technology during the Late Pleistocene. The discovery of a cache of 17 blades at Blackwater Draw in 1962 and subsequent discoveries of blades in association with butchered mammoths led Green (Reference Green1963:157) to conclude that there was “unequivocal proof of a blade industry as an integral part of the [Clovis] complex.” Later research at sites like Anadarko, Keven Davis, and Gault further solidified Green's hypothesis that blades struck from prepared cores were a significant component of Clovis lithic technology (Bradley et al. Reference Bradley, Collins and Hemmings2010; Collins Reference Collins1999; Hammatt Reference Hammatt1970; Waters et al. Reference Waters, Pevny, Carlson, Collins, Jennings, Dickens, Minchak, Smallwood, Wiersema and Bartelink2011).
Kilby (Reference Kilby, Smallwood and Jennings2015) has demonstrated that blades and blade cores were a frequent component of utilitarian Clovis caches across much of the Southern Plains. Blades from Clovis contexts are documented from Texas and Oklahoma at the Gault, Keven Davis, Pavo Real, Aubrey, and Anadarko sites (Bradley et al. Reference Bradley, Collins and Hemmings2010; Collins Reference Collins1999; Ferring Reference Ferring2001; Hammatt Reference Hammatt1970; Waters et al. Reference Waters, Pevny, Carlson, Collins, Jennings, Dickens, Minchak, Smallwood, Wiersema and Bartelink2011). Extensive evidence of blade production has been documented in the Allendale Brier Creek Complex and other locations throughout South Carolina and Georgia (Sain and Goodyear Reference Sain and Goodyear2016; Smallwood Reference Smallwood2015). Clovis blade technology is also documented in the Tennessee and Ohio River drainages (Ellerbusch Reference Ellerbusch2004; Norton et al. Reference Norton, Broster, Burgess and Mabrey2011; Sanders Reference Sanders1990; Stanford et al. Reference Stanford, Canales, Broster and Norton2006). Though not as thoroughly reported as other sites, the Carson-Conn-Short site in Tennessee and the Adams site in Kentucky demonstrate extensive Clovis prismatic blade production in the Midsouth (Haag et al. Reference Haag, Bergman and Carr2014; Norton et al. Reference Norton, Broster and Jones2020; Sanders Reference Sanders1990; Stanford et al. Reference Stanford, Canales, Broster and Norton2006).
The blade assemblage from the Sinclair site in Tennessee provides a unique opportunity to comprehensively study blade technology and how it may have fit within a broader Clovis technological organization. The Sinclair assemblage is characterized here based on quantitative and qualitative attributes and is compared to other known Clovis and post-Clovis blade assemblages. We hypothesize the objective of Clovis blade making, examine whether variation in blade morphology can be explained by site type, and place the blades from the Sinclair site within a broader Clovis technological context. Finally, we assess whether Clovis blades can be statistically differentiated from non-Clovis blade technologies.
The Sinclair Assemblage
The Sinclair site (40WY111) overlooks the Buffalo River in Wayne County, Tennessee. Large, tabular, rectangular-to-angular chert nodules are readily accessible on-site. Broster and Norton's (Reference Broster and Norton2009) investigation documented at least 10 discrete activity areas and hundreds of Clovis and other Paleoindian artifacts. The site area was severely disturbed in 2007 and 2008 by bulldozing and plowing to convert the wooded landscape into pastureland. Because of the limited spatial information available, we cannot evaluate how discrete activity areas may have been affected by recent postdepositional activities.
Broster and Norton's (Reference Broster and Norton2009) surface survey was conducted opportunistically, resulting in a collection of complete and broken blades and cores, which are curated at the Tennessee Division of Archaeology (TDOA). Photographs were taken of blade cores, and representative examples were collected as part of the TDOA's investigations; however, the limited number of cores collected does not allow a meaningful assessment of core-reduction techniques used at Sinclair at this time. Additionally, broken Clovis preforms and fluted points, bifaces, and debitage were recovered in 2008. Broster and Norton (Reference Broster and Norton2009) note that Late Paleoindian bifaces and debitage occurred in a single discrete area. The site assemblage also includes previously unreported Early and Middle Archaic artifacts.
Clovis Blade Technology
The term “blade” refers here to a flake with parallel to slightly convergent edges and with parallel or subparallel flake scars on the dorsal face running parallel to the long axis, having a length-to-width ratio of at least 2:1, and removed from a prepared core (Collins Reference Collins1999:191). Clovis blades are typically prismatic-like in cross section and possess small striking platforms, diffuse bulbs of percussion, a smooth interior surface, and subparallel scars on the exterior surface (Collins Reference Collins1999). Crabtree (Reference Crabtree1968) adds that blades are associated with a specialized lithic reduction technique requiring the use of carefully prepared cores; that is, they are not fortuitously produced long, narrow flakes.
The production of blades is one of two formal core-reduction strategies known to have been used by Clovis flintknappers, the other being bifacial core reduction. Conical and wedge-shaped cores were used by Clovis flintknappers to produce blades (Bradley et al. Reference Bradley, Collins and Hemmings2010; Collins Reference Collins1999; Waters et al. Reference Waters, Pevny, Carlson, Collins, Jennings, Dickens, Minchak, Smallwood, Wiersema and Bartelink2011). Given the typical roundness of river cobbles, these raw material packages naturally lent themselves to the production of conical blade cores. Rectangular to subrectangular tabular raw material packages were more conducive to producing wedge-shaped blade cores. This pattern in raw material package shape and blade core morphology is supported by data from the Gault site (Waters et al. Reference Waters, Pevny, Carlson, Collins, Jennings, Dickens, Minchak, Smallwood, Wiersema and Bartelink2011). Although Collins (Reference Collins1999) recognized that conical and wedge-shaped cores were used by Clovis flintknappers for blade production, his proposed six-stage reduction sequence is most applicable for assessing conical blade cores.
Waters and coauthors (Reference Waters, Pevny, Carlson, Collins, Jennings, Dickens, Minchak, Smallwood, Wiersema and Bartelink2011; also see Bradley et al. Reference Bradley, Collins and Hemmings2010) conducted a detailed study of Clovis blade technology at the Gault lithic workshop where wedge-shaped, rather than conical, cores were mainly used. The rectangular shape of tabular Edwards Plateau chert nodules at Gault may have favored wedge-shaped reduction and may explain why wedge-shaped cores outnumber conical cores by 9 to 1 (Waters et al. Reference Waters, Pevny, Carlson, Collins, Jennings, Dickens, Minchak, Smallwood, Wiersema and Bartelink2011). Most notably, Waters and coauthors (Reference Waters, Pevny, Carlson, Collins, Jennings, Dickens, Minchak, Smallwood, Wiersema and Bartelink2011) documented that late-stage blades produced from wedge-shaped cores retain a straighter profile, whereas on conical cores, late-stage blades typically exhibit a high degree of curvature, as noted by Collins (Reference Collins1999).
Methods
Summary statistics were calculated for a sample of 117 blades and blade fragments from the Sinclair assemblage. Following Collins (Reference Collins1999) and others (Eren and Redmond Reference Eren and Redmond2011; Waters et al. Reference Waters, Pevny, Carlson, Collins, Jennings, Dickens, Minchak, Smallwood, Wiersema and Bartelink2011), we documented the overall size of blades by the sum of length, width, and thickness. The results are summarized and presented here, with raw measurements provided in Supplemental Table 1 to aid future additional comparative analyses.
Qualitative attributes of complete blades were documented to assess relative stages of blade removal. We combined the six stages of reduction defined by Collins (Reference Collins1999) into early (1–2), middle (3–4), and late (5–6) stages to better reflect the continual variation observed between blades from successive removal episodes. Early-stage blades are characterized by a high percentage of dorsal surface cortex and few flake scars, large bulbs of percussion, large striking platforms, and a relatively straight longitudinal profile. Middle-stage blades are characterized by numerous dorsal flake scars and less cortex, moderate bulbs of percussion, and slight-to-moderate longitudinal curvature. Late-stage blades are characterized by dorsal surfaces with numerous flake scars and minimal to no cortex, diffuse bulbs of percussion, small platforms, and strong longitudinal curvature. The rate of reduction for blade width and length is assessed by calculating the percent loss after each stage of the reduction process.
Eren and coauthors (Reference Eren, Meltzer and Andrews2018) use the presumably Archaic period Gibson assemblage (Tunnell Reference Tunnell1978) to highlight the potential utility of using a known non-Clovis assemblage to help identify Clovis blades in assemblages without clearly associated Clovis points. We chose not to use the Gibson assemblage here because, as Collins (Reference Collins1999:171) notes, “So many of the Gibson specimens are retouched that the cache might more accurately be referred to as a cache of scrapers.” Nor do we include the Goodson Rockshelter assemblage as an outgroup because that site is so newly reported and the affinity of the artifacts is currently being debated (Eren et al. Reference Eren, Meltzer and Andrews2021; Huckell et al. Reference Huckell, Vance Haynes and Holliday2019). Instead, we use two blade assemblages—Barton (Ricklis Reference Ricklis, Ricklis and Collins1994) and Kirchmeyer (Hester and Shafer Reference Hester and Shafer1975) associated with Perdiz points—that are diagnostic of the Late Prehistoric Toyah phase in Texas (Collins Reference Collins1999). Toyah blades have been shown elsewhere to be morphologically distinct from Clovis blades (Collins Reference Collins1999; Jennings and Smallwood Reference Jennings, Smallwood, O'Brien, Buchanan and Eren2018). Following Eren and coauthors (Reference Eren, Meltzer and Andrews2018), we use discriminant function analysis (DFA) to compare blades. We use the Gault Clovis assemblage to define Clovis blades and group Barton and Kirchmeyer in a Toyah group as the non-Clovis outgroup.
We then compared all Clovis blade assemblages discussed here based on site type. Assemblages from lithic workshops (Sinclair, Gault, and Pavo Real) were compared to blade caches (Blackwater Draw, Keven Davis, and Anadarko) and multi-use campsites (Murray Springs, Paleo Crossing, and Nuckolls; Figure 1). However, the Blackwater Draw (Green cache), Keven Davis, and Anadarko assemblages do not have radiometric dates or Clovis points directly associated with them. We tested for broader technological patterns related to Clovis band movement across the landscape. Coefficients of variation (CVs) were calculated to compare relative standardization among assemblages (Eerkens and Bettinger Reference Eerkens and Bettinger2001). Comparative statistical tests included parametric ANOVA and nonparametric Kruskal-Wallis depending on the outcomes of normality and variance tests (VanPool and Leonard Reference VanPool and Leonard2011).

Figure 1. Distribution of Clovis prismatic blade assemblages compared in this study. Triangles denote workshops, circles denote campsites, and squares denote caches.
Results
Sinclair Blades
All blades analyzed from Sinclair are macroscopically consistent with Fort Payne chert. The assemblage consists of 47 complete blades, 33 proximal fragments, 11 medial fragments, and 26 distal fragments. Although all stages of blade production are represented at Sinclair, the assemblage is dominated (57.45%; n = 27) by early-stage blades (Figure 2; Supplemental Table 2).
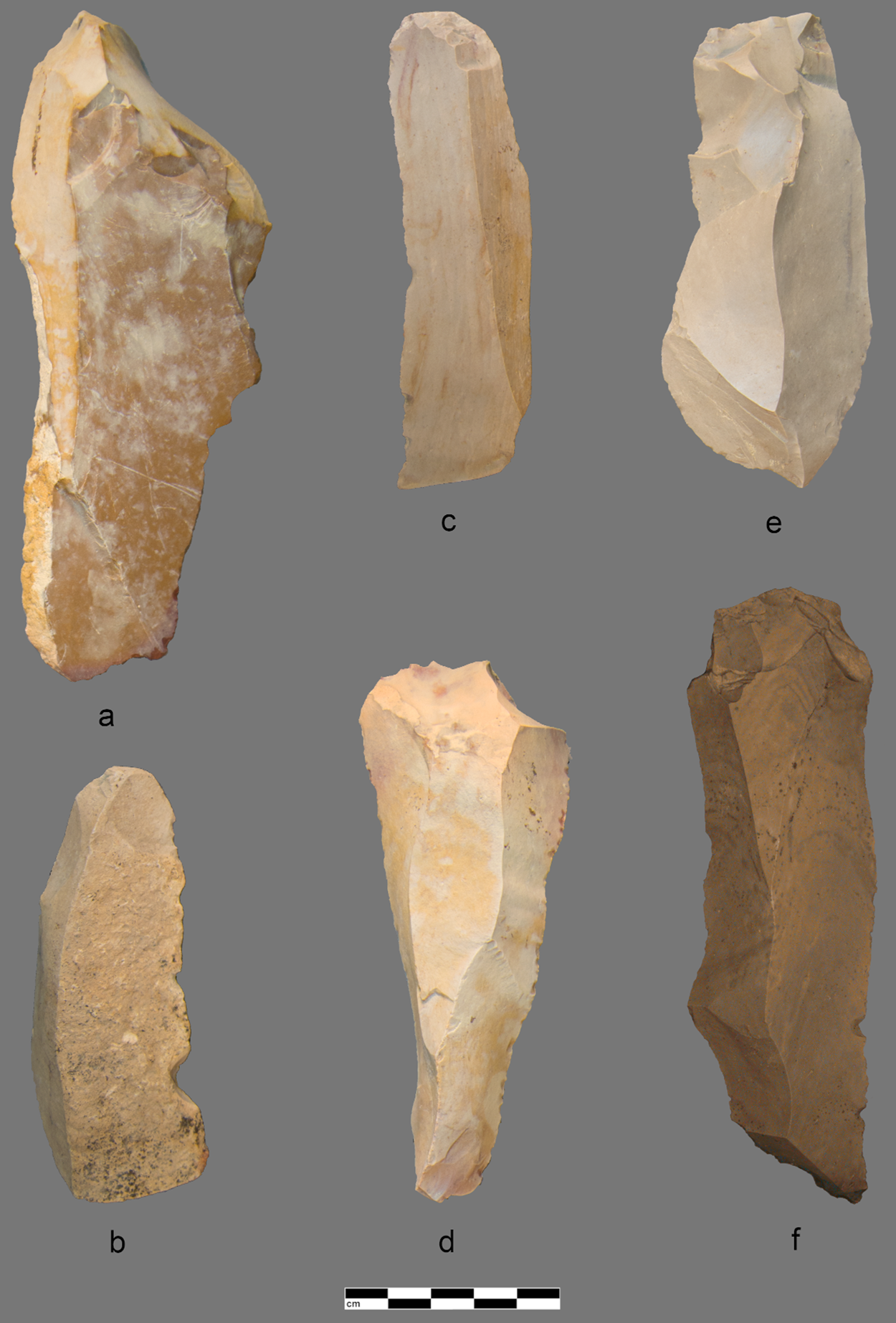
Figure 2. Representative selection of prismatic blades from the Sinclair site. Early-stage blades (a) 08-49-40 and (b) 09-5-94. Middle-stage blades (c) 08-49-690 and (d) 08-49-104. Late-stage blades (e) 08-49-41 and (f) 09-5-98. Catalog numbers correspond to the inventory provided in Supplemental Table 1. (Color online)
All variables are normally distributed with equal variances, with the exception of thickness. A series of ANOVA tests (and Kruskal-Wallis for the thickness comparison) were conducted with Bonferroni post-hoc comparisons to quantitatively identify differences between blade stages (Table 1). The only significant difference identified between reduction stages was blade width (p = 0.033). Post-hoc comparison shows that this significance is driven by the difference between early- and middle-stage blades (p = 0.042) This is not surprising: it likely reflects the fact that the production of blades occurred along a continuum in a lithic-reduction process, rather than in discrete steps.
Table 1. Results of ANOVA and Kruskal-Wallis Tests of Complete Prismatic Blades by Reduction Stages from the Sinclair Assemblage.

Notes: ANOVA measured length, width, length:width, and length + width + thickness; Kruskal-Wallis tests measured thickness.
Although the length-to-width ratios between stages are nearly significant (p = 0.055), there appears to be an inverse relationship between blade size and length-to-width ratios within the Sinclair assemblage. Earlier-stage blades have smaller length-to-width ratios and larger dimensions, and later-stage blades have higher length-to-width ratios and smaller dimensions. This suggests that, although all dimensions decrease throughout blade reduction, width decreases faster and to a greater extent than length. This pattern is reinforced when percent loss in dimensions is calculated throughout the blade-reduction process (Table 2). The average blade width is reduced by 20.08% from the early to middle stage, and by 2.01% from the middle to late stage; average blade length is reduced by 3.39% from the early to middle stage, and by 0.80% from the middle to late stage. However, the presumed removal of late-stage blades from the Sinclair site may influence these relationships by skewing the sample toward the earlier end of the reduction process.
Table 2. Comparison of Percent Change in Complete Blade Dimensions by Reduction Stage in the Sinclair Assemblage.

When assessing the blade dimensions and relationships between those dimensions by stages of reduction, notable patterns emerge. The CVs of individual blade dimensions increase throughout the reduction process (Supplemental Table 2), and the correlations between length-to-width and width-to-thickness become stronger (Table 3). Essentially, as blade cores are reduced, individual blade dimensions become more variable, and the correlations between those dimensions become stronger. The lack of statistical significance of correlations in late-stage blades is likely related to the very small sample size (n = 3).
Table 3. Results of Pearson's Correlations between Length, Width, and Thickness for Complete Prismatic Blades in the Sinclair Assemblage.

Comparison to other Blade Assemblages
DFA successfully distinguishes Clovis from non-Clovis (Toyah) blades (Supplemental Table 3). Of the 74 blades from sites previously described as Clovis, only 3 (4%) are incorrectly classified as non-Clovis. Of the 18 non-Clovis blades, 3 (16%) are incorrectly classified as Clovis. For both Clovis and non-Clovis, the blades that are classified incorrectly all come from workshop sites. This suggests that, even though Clovis blades can be distinguished from non-Clovis blades at the assemblage level, individual blades do occasionally overlap morphologically. For Sinclair, 43 of 47 (91.5%) are classified as Clovis. This analysis supports the Clovis affinity of Sinclair blades.
Individual blades from Sinclair are larger than those from most other Clovis assemblages (Supplemental Table 4). The Sinclair assemblage falls within the known range of variation for Clovis blades and groups with other workshop sites (Figure 3). Although maximum dimensions and overall size may be affected by factors such as raw material package size, comparisons of morphological ratios and relative measures of assemblage variability more directly reflect technological aspects that are independent of such factors. Thus, they may provide more meaningful insight into blade technologies in general. Length-to-width ratios are highest in caches and lowest in campsites. Based on the calculated CVs, cached blade lengths are the most standardized (Figure 4). Campsite blade widths are most standardized, and cached blade thicknesses are most standardized. Campsite blade length-to-width ratios are most standardized. For all four measures, workshop blades are the most variable and the least standardized.

Figure 3. Scatterplots of blade means grouped by site type. Lines represent means within each site type.

Figure 4. Scatterplots of blade CVs grouped by site type. Lines represent means within each site type.
Discussion
Given sufficient raw material size and availability, it appears that the objective of Clovis blade technology was to produce standardized, long, narrow blades while maximizing overall size. Those standardized blades were then selected to be modified into tools and used at other sites. Late-stage blades, those that fit into what Waters and coauthors (Reference Waters, Pevny, Carlson, Collins, Jennings, Dickens, Minchak, Smallwood, Wiersema and Bartelink2011) refer to as cache-quality blades, were likely removed from Sinclair to be used as tools at other locations. Sain and Goodyear (Reference Sain and Goodyear2016) noted a similar pattern in the Allendale Brier Creek Clovis Complex.
The Sinclair, Gault, and Pavo Real workshop sites are dominated by large, earlier-stage blades with variable length-to-width ratios. Blackwater Draw, Keven Davis, and Anadarko caches are dominated by large, later-stage blades with more standardized length-to-width ratios, blade lengths, and blade thicknesses. The Paleo Crossing, Murray Springs, and Nuckolls campsite assemblages are dominated by blade tools of variable lengths that demonstrate extensive use and reduction, resulting in small length-to-width ratios. Campsite blades, however, still retain high width and length-to-width standardization.
These assemblage-level differences reveal how blade technology was organized across the landscape (Figure 5). The Sinclair, Gault, and Pavo Real sites are chert quarries where primary reduction of raw materials occurred; the assemblages there reflect early-stage reduction. Workshop assemblages are highly variable in terms of blade dimensions and ratios and contain very few blade tools. Keven Davis, Blackwater Draw, and Anadarko are cache sites where middle- to late-stage blades were intentionally deposited in anticipation of future use (Kilby Reference Kilby, Huckell and Kilby2014). All three assemblages are made up of relatively large, very standardized blades and few blade tools. The Murray Springs, Nuckolls, and Paleo Crossing sites are locations where a variety of activities occurred. These assemblages are dominated by late-stage blades and have substantially higher quantities of blade tools.
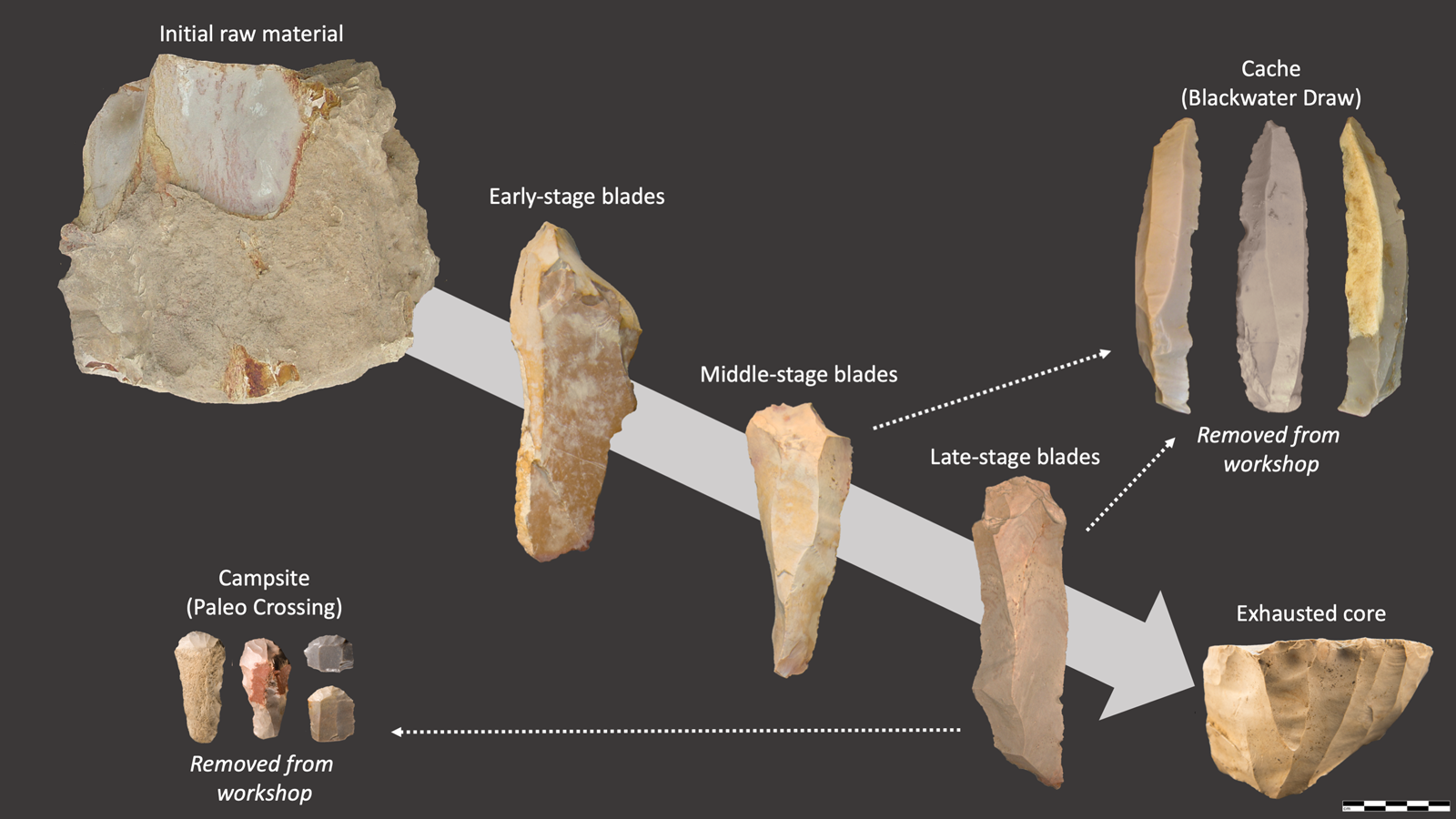
Figure 5. Generalized organization of Clovis prismatic blade technology (images of Paleo Crossing artifacts used with the permission of Metin I. Eren, Kent State University: images of Blackwater Draw artifacts used with the permission of David Kilby, Texas State University). (Color online)
Conclusion
The Sinclair site in Tennessee is a chert quarry and workshop that were extensively used during the Late Pleistocene. Although Early Paleoindian through Early Archaic biface fragments are documented at Sinclair, it appears that one of the main activities at the site was the production of prismatic blades. We show that these blades are technologically and morphologically Clovis. Early-stage blades dominate the Sinclair assemblage, suggesting that late-stage blades were removed from the site for use elsewhere. As more sites are being reported in the region, it is clearer that the Midsouth was a substantial center of Clovis technological innovation and potentially of its diffusion. We propose that the Midsouth, specifically the Lower Tennessee River drainage, was a core region of Clovis blade technology.
Acknowledgments
We thank Rex Moore, who donated the artifacts analyzed here to the Tennessee Division of Archaeology. This has allowed for a detailed study of the assemblage and facilitates access to future researchers. John Broster and Mark Norton recognized the significance of the Sinclair site and worked to make this dataset available to researchers. David Kilby and Metin I. Eren graciously provided some images used in Figure 5. We acknowledge the Indigenous peoples who have called North America home for at least ~13,500 years. We are grateful for the opportunity to study the material culture left behind by people who lived here so long ago. Thanks to Metin Eren and anonymous reviewers for providing comments to improve this article.
Data Availability Statement
All data used in this study are available in in-text tables and supplemental online material. A complete inventory with accession numbers is provided in the supplemental online material. The Sinclair dataset discussed here is permanently curated at the Tennessee Division of Archaeology.
Competing Interests
The authors declare none.
Supplemental Material
For supplemental material accompanying this article, visit https://doi.org/10.1017/aaq.2022.16.
Supplemental Table 1. Inventory and Description of the Sinclair Blade Assemblage.
Supplemental Table 2. Descriptive Statistics for Complete Prismatic Blades from the Sinclair Assemblage.
Supplemental Table 3. Results of DFA Using Gault as a Clovis Proxy and Barton and Kirchmeyer as Non-Clovis Proxies.
Supplemental Table 4. Descriptive Statistics for Clovis Prismatic Blade Assemblages.