Significant outcomes
Serum dynorphin and kappa opioid receptor (KOR) levels are significantly increased in depression (MDD) suggesting that dynorphin/KOR signaling is increased.
In MDD, dynorphin/KOR and β-endorphin/mu opioid (MOR) signaling are significantly intercorrelated and associated with immune activation.
It is hypothesised that both KOR and MOR systems participate in the pathophysiology of depression by exerting immune regulatory as well as emotional and behavioural effects.
Limitations
It would have been more interesting if we had measured more cytokines including those of M1 macrophage, T helper (Th)-1, Th-2, Th-17 and T regulatory phenotypes.
This study examined male subjects, and therefore our study deserves replication in females.
Introduction
Major depressive disorder (MDD) is a chronic relapsing disorder characterised by the recurrence of major depressive episodes. A recent meta-analysis reported that, from 1994 to 2014, the lifetime prevalence of depression was 10.8% (Lim et al., Reference Lim, Tam, Lu, Ho, Zhang and Ho2018). There is now evidence that MDD is associated with changes in immune functioning and activation of immune-inflammatory pathways comprising increased production of pro-inflammatory [e.g. interleukin-6 (IL-6)] as well as immune-regulatory (e.g. IL-10) cytokines (Maes et al., Reference Maes, Jacobs, Suy, Leclercq, Christiaens and Raus1990; Al-Hakeim et al., Reference Al-Hakeim2008; Catena-Dell’osso et al., Reference Catena-Dell’osso, Rotella, Dell’osso, Fagiolini and Marazziti2013; Young et al., Reference Young, Bruno and Pomara2014; Köhler et al., Reference Köhler, Freitas, Maes, de Andrade, Liu, Fernandes, Stubbs, Solmi, Veronese, Herrmann, Raison, Miller, Lanctôt and Carvalho2017; Al-Hakeim et al., Reference Al-Hakeim, Al-Kufi, Al-Dujaili and Maes2018a; Maes & Carvalho, Reference Maes and Carvalho2018). The latter are part of a regulatory system that down-regulates the primary immune-inflammatory response via multiple negative feedback systems, collectively named the ‘compensatory immune regulatory system’ (CIRS) (Al-Hakeim et al., Reference Al-Hakeim, Twayej and Al-Dujaili2018b; Maes & Carvalho, Reference Maes and Carvalho2018).
Aberrations in endogenous opioid peptides and their receptors may occur in MDD. The opioid system comprises different neuropeptide families including dynorphins, endorphins and enkephalins, and three receptor subtypes, namely kappa opioid receptor (KOR), mu opioid receptor (MOR) and delta opioid receptor (DOR) receptors (Snyder, Reference Snyder2004). β-Endorphin and enkephalins bind to MORs and DORs, while dynorphin binds predominately to KORs (Ninković, Reference Ninković2013). Both opioid receptors and opioid peptides are expressed throughout peripheral and central nervous systems (Le Merrer et al., Reference Le Merrer, Becker, Befort and Kieffer2009). A growing body of research indicates that those endogenous opioids and their receptors are involved in emotional and behavioural responses to stress, regulation of mood and the pathophysiology of MDD (Al-Fadhel et al., Reference Al-Fadhel, Al-Hakeim, Al-Dujaili and Maes2019; Peciña et al., Reference Peciña, Karp, Mathew, Todtenkopf, Ehrich and Zubieta2019). MDD is often accompanied by increased baseline and post-dexamethasone β-endorphin concentrations, although there are some reports that β-endorphin levels may be lowered (Facchinetti et al., Reference Facchinetti, Petraglia, Sances, Garuti, Tosca, Nappi and Genazzani1986; Maes et al., Reference Maes, Jacobs, Suy, Leclercq, Christiaens and Raus1990; Darko et al., Reference Darko, Irwin, Risch and Gillin1992; Lutz & Kieffer, Reference Lutz and Kieffer2013). In animal models, increased β-endorphin secretion is associated with depressive-like behaviours (Lutz & Kieffer, Reference Lutz and Kieffer2013). MOR is a key component of reward processing, while acute activation of MOR attenuates depressive-like behaviours in some but not all studies (Lutz & Kieffer, Reference Lutz and Kieffer2013). Recently, we reported that MOR levels were significantly higher in MDD patients than controls (Al-Fadhel et al., Reference Al-Fadhel, Al-Hakeim, Al-Dujaili and Maes2019), while post-mortem studies showed increased MOR binding in suicide victims (Escriba et al., Reference Escriba, Ozaita and García-Sevilla2004). Positron emission tomography, on the other hand, showed contradictory results with increased binding potential for [11C]-carfentanil during depression but decreased binding potential in women with MDD (Pecina & Zubieta, Reference Pecina and Zubieta2018). Subjects with a lower expression of MORs and a reduced capacity to release endogenous opioids may show increased vulnerability to develop depressive symptoms and show a poor treatment response to selective serotonin reuptake inhibitors (SSRIs) (Kennedy et al., Reference Kennedy, Koeppe, Young and Zubieta2006; Pecina et al., Reference Pecina, Love, Stohler, Goldman and Zubieta2015a). In addition, reduced baseline MOR availability in the nucleus accumbens predicts a lack of response to SSRIs (Pecina et al., Reference Pecina, Bohnert, Sikora, Avery, Langenecker, Mickey and Zubieta2015b). A variety of stressors may increase KOR signaling, which is involved in stress-induced alterations in brain reward systems and associated despair- and depression-like responses and depressive disorders (Carlezon & Thomas, Reference Carlezon and Thomas2009; Bruchas et al., Reference Bruchas, Land and Chavkin2010; Knoll & Carlezon, Reference Knoll and Carlezon2010; Lutz & Kieffer, Reference Lutz and Kieffer2013; Bailey & Husbands, Reference Bailey and Husbands2018). Consequently, KOR signaling is sometimes regarded as an ‘anti-reward dysphoric system’, which once activated may increase risk towards MDD (Lalanne et al., Reference Lalanne, Ayranci, Kieffer and Lutz2014; Taylor & Manzella, Reference Taylor and Manzella2016). The overall conclusion from animal models supports the idea that the KOR system may underpin aspects of sadness and dysphoria but that the cause of the opioid alterations in MDD remains inconclusive (Nummenmaa & Tuominen, Reference Nummenmaa and Tuominen2018). Nevertheless, in a pilot study, no differences in KOR binding (using [11C]GR103545) could be found between MDD patients and controls (Miller et al., Reference Miller, Zanderigo, Purushothaman, DeLorenzo, Rubin-Falcone, Ogden, Keilp, Oquendo, Nabulsi, Huang, Parsey, Carson and Mann2018), while prodynorphin mRNA was significantly lower in MDD and bipolar depression as compared with controls (Hurd, Reference Hurd2002). However, there is a lack of KOR measurements in the literature, which in part may be explained by limitations in techniques to measure KOR and inadequate knowledge about the exact source of KOR in plasma (Suzuki et al., Reference Suzuki, Chuang, Doi, Bidlack and Chuang2001; Rittner et al., Reference Rittner, Brack and Stein2008). There is now evidence that the immune and opioid systems show functional relationships. Monocytes, macrophages, granulocytes, and T and B-lymphocytes express opioid peptides, including β-endorphin and pro-opiomelanocortin (POMC) and all POMC processing enzymes (Mousa et al., Reference Mousa, Shakibaei, Sitte, Schäfer and Stein2004; Pomorska et al., Reference Pomorska, Gach and Janecka2014). Circulating leukocytes may produce opioid peptides and migrate into inflamed tissues where they exert immune stimulatory and regulatory activities (Rittner et al., Reference Rittner, Machelska and Stein2005; Pomorska et al., Reference Pomorska, Gach and Janecka2014). Lymphocytes functions involved in the host defense are modulated by β-endorphin and dynorphin (Bidlack et al., Reference Bidlack, Khimich, Parkhill, Sumagin, Sun and Tipton2006). Associations between both systems are also detected in patients with MDD. For example, lowered cell-mediated hypersensitivity and natural killer activity are significantly associated with increased β-endorphin levels in depression (Maes et al., Reference Maes, Vandervorst, Suy, Minner and Raus1991b; Pomorska et al., Reference Pomorska, Gach and Janecka2014). Castilla et al. (Reference Castilla, Subirà, Civeira, Cuende and Prieto1992) reported an increased opioid tone in depression and a concomitant suppression of monocytic functions. Recently, we reported significant associations between MOR levels and IL-10 in patients with major depression (Al-Fadhel et al., Reference Al-Fadhel, Al-Hakeim, Al-Dujaili and Maes2019). Nevertheless, the associations between immune activation and the KOR/dynorphin system were not studied in patients with MDD.
There is also a strong comorbidity between MDD and nicotine dependence (ND) with increased inflammatory potential and affiliated nitro-oxidative pathways in comorbid MDD and ND (Nunes et al., Reference Nunes, Vargas, Prado, Barbosa, de Melo, Moylan, Dodd and Berk2013; Nunes et al., Reference Nunes, Piccoli de Melo, Pizzo de Castro, Barbosa, Vargas, Berk and Maes2015a,b). Nicotine has a biphasic effect on the opioid system with anti-opioid effects at lower doses, while nicotine administration may entrain opioid activity ‘during the acquisition and re-acquisition of nicotine self-administration’ (Pomerleau, Reference Pomerleau1998). Nevertheless, the effects of comorbid MDD and ND on the KOR system have not been examined.
Hence, the present study was carried out to delineate (a) KOR/dynorphin system in association with MOR/endorphin system and serum IL-6 and IL-10 levels in MDD patients as compared to healthy controls and (b) examine the effects of comorbid MDD and ND on these associations.
Subjects and methods
Participants
The present study examined 60 drug-free MDD males and 30 age-matched healthy males. Participants were recruited at ‘The Psychiatry Unit’, Al-Hakeem General Hospital and at a private psychiatric clinic, Najaf Governorate, Iraq during the period of January–July 2017. This study is a first report on KOR and dynorphin values in this sample. These biomarkers were measured in the same study group on which we published MOR, β-endorphin, IL-6 and IL-10 data (Al-Fadhel et al., Reference Al-Fadhel, Al-Hakeim, Al-Dujaili and Maes2019), except that one patient and one control were substituted by two new cases with complete dynorphin/KOR measurements. The 4th edition of Diagnostic and Statistical Manual of Mental Disorders (American Psychiatric Association, 2000) was used to make the diagnosis of MDD. The 24-item Hamilton Depression Rating Scale (HDRS) was employed 1 or 2 days before blood was drawn to measure severity of depression. We included only patients who showed a HDRS score of more than 21. Patients were divided into those with and without ND, and all ND patients showed heavy nicotine use of more than 20 cigarettes/day.
We completed a full medical history using a semi-structured interview. Exclusion criteria for patients and controls were immune disorders including diabetes mellitus, autoimmune disorders, inflammatory bowel disorder, psoriasis, chronic obstructive pulmonary diseases (COPD) and chronic kidney disease. Excluded were also subjects with neurodegenerative/neuroinflammatory diseases including stroke, multiple sclerosis, and Alzheimer’s and Parkinson’s diseases. Only drug-free patients were included in this study. MDD patients were excluded when they showed other-axis I diagnosis including bipolar disorder, substance abuse, psycho-organic disorders and schizophrenia. Serum C-reactive protein (CRP) levels were lower than 6 mg/l, thereby excluding subjects with overt inflammation. Written informed consent was obtained from all participants, according to the guidelines laid down in the current version of the Declaration of Helsinki, after approval from the ethics committee (IRB) of the College of Science, University of Kufa, Iraq (229-1/2017).
Methods
Five milliliters of venous blood samples was drawn, utilising disposable needle and plastic syringes, from patients and controls. The samples were transferred into a clean plain tube. Blood was left at room temperature for 15 min for clotting, centrifuged 3000 rpm for 10 min and then serum was separated and transported into two Eppendorf tubes to be stored at −80 °C until thawed for assay. Serum CRP was measured using a kit supplied by Spinreact®, Girona, Spain. This test is based on the principle of the latex agglutination. Commercial ELISA kits were used to measure KOR (Human Kappa-Type Opioid ELISA Kit) and MOR (Human Mu-Type Opioid Receptor ELISA Kit) (MyBioSource, San Diego CA, USA). The sensitivity of the KOR and MOR assays was 0.019 and 0.029 ng/ml, respectively, and the detection ranges were 0.05–15 ng/ml and 0.05–30 ng/ml, respectively. MyBioSource specifies that ‘no significant cross-reactivity or interference between human OPRM1 and analogues was observed’. Dynorphin was assayed using the Human Dynorphin ELISA Kit supplied by (Cusabio Co, Wuhan, China) and the β-Endorphin was assayed by Human β-Endorphin ELISA Kit supplied by (Melsin Medical Co., Ltd Changchun, China) which show a sensitivity of 7.8 and 0.1 pg/ml, respectively. IL-6 and IL-10 were assayed using the Human Interleukin-6 and Interleukin-10 ELISA Kits (Cusabio Co) which have a sensitivity of 2.4 and 3.1 pg/ml, respectively. We followed all procedures according to the manufacturer’s instructions. The intra-assay coefficients of variation (precision within-assay) were <8.0% for all analytes.
Statistical analysis
Differences in scale variables between diagnostic groups were examined using the analysis of variance. Associations between nominal variables were assessed using the analysis of contingency tables (χ2 test). We used Pearson’s product moment correlation coefficients to check associations between scale variables. Multivariate general linear model (GLM) analysis was used to assess the effects of diagnosis (MDD with and without ND versus controls) as primary explanatory variable, while adjusting for extraneous variables (age and BMI). Tests for between-subject effects were employed to assess the effects of significant explanatory variables on biomarkers. Multiple post-hoc comparisons among treatment means were assessed using protected least significant difference (LSD) values. Differences in the biomarkers among classes are displayed as mean (SE) values computed on their z scores. Binary logistic regression analysis was employed to delineate the most important predictors of MDD versus controls, with computation of odds ratios and 95% confidence intervals. We computed the area under the curve (AUC) to determine the diagnostic accuracy of the biomarkers as well as the bootstrapped (2000 bootstraps) AUC values. An optimal diagnostic tool will have an AUC of 1, which indicates 100% sensitivity and specificity (Bewick et al., Reference Bewick, Cheek and Ball2004). Tests were two-tailed and a p-value of 0.05 was used for statistical significance. All statistical analyses were performed using IBM SPSS windows version 25, 2017.
Based on the measurements of IL-6, IL-10, β-endorphin, dynorphin, KOR and MOR, we computed z unit weighted composite scores as follows:
DYN-KOR: index of KOR signaling computed as z transformation of dynorphin (zDYN) + zKOR.
DYN-END: integrated index of circulating opioid peptides, computed as zDYN + z β-endorphin.
KOR-MOR: index of opioid receptor status computed as zKOR + zMOR.
IL6-IL10: index of immune activation computed as zIL-6 + zIL-10.
Results
Descriptive statistics
Table 1 shows the socio-demographic data as well as the raw values of the biomarkers used in this study. Patients were divided into those with (MDD + ND) and without ND (MDD). There were no significant differences in age, BMI and urban/rural ratio between the three study groups. There were somewhat more married subjects in MDD + ND than in controls, while there were more unemployed people in both depressed subgroups than in controls. This table also shows the raw measurements (not adjusted for extraneous variables) of the different biomarkers. Figure 1 shows the differences in biomarker profile between the three study groups. Shown are the group mean values (±SE) after z transformations were made. The Beck depression inventory (BDI-II) score was not significantly different between both MDD subgroups. In the MDD group, there was no significant correlation between the BDI score and any of the biomarkers.
Table 1. Socio-demographic, clinical and biomarker data in major depressed patients with nicotine dependence (MDD + ND), patients without ND (MDD) and healthy controls (HC)

BMI, body mass index; KOR, kappa opioid receptor; END, β-endorphins; MOR, mu opioid receptor; IL, interleukin; BDI-II, Beck depression inventory.
A,B,CResults of pairwise comparisons between group means.
Results are shown as mean (±SD).

Fig. 1. Differences in biomarker profile between controls (HC) and major depressed patients with (MDD + ND) and without (MDD) nicotine dependence. Shown are the group mean values (±SE) after z transformations were made. DYN, dynorphin; KOR, kappa opioid receptor; DYN-KOR, index of KOR signaling computed as z transformation of dynorphin (zDYN) + zKOR; END, β-endorphin; MOR, mu opioid receptor; DYN-END, integrated index of circulating opioid peptides, computed as zDYN + zEND; KOR-MOR, index of opioid receptor status computed as zKOR + zMOR; IL, interleukin; IL6-IL10, index of immune activation computed as z interleukin-6 (zIL6) + zIL10.
Table 2 shows the intercorrelation matrix (obtained using partial correlation coefficients after adjusting for age, BMI and ND) between different biomarkers in the 90 subjects included in this study. Dynorphin (DYN) was significantly correlated with β-endorphin (END), MOR, KOR-MOR and IL6-IL10. KOR was significantly associated with END, MOR, DYN-END and IL6-IL10. DYN-KOR was significantly correlated with END, MOR and IL6-IL10. END levels were significantly correlated with MOR, KOR-MOR and IL6-IL10. DYN-END was significantly associated with KOR-MOR and IL6-IL10, while KOR-MOR was positively associated with IL6-IL10. Figure 2 shows the association between KOR and IL6-IL10, while Fig. 3 shows the correlation between DYN-END and IL6-IL10. Figure 4 shows the association between KOR-MOR and IL6-IL10. These correlations in the figures were not adjusted for covariates.
Table 2. Inter-correlation matrix among biomarkers data

KOR, kappa opioid receptor; DYN-KOR, index of KOR signaling computed as z transformation of dynorphin (zDYN) + zKOR; END, β-endorphin; MOR, mu opioid receptor; DYN-END, integrated index of circulating opioid peptides, computed as zDYN + zEND; KOR-MOR, index of opioid receptor status computed as zKOR + zMOR; IL6-IL10, index of immune activation computed as z interleukin-6 (zIL6) + zIL10.
All p < 0.001, except *: p < 0.01 (n = 90).
Shown are the partial correlation coefficients after adjusting for age, body mass index and nicotine dependence.

Fig. 2. Association between kappa opioid receptor (KOR) levels and immune activation as indicated by a z unit weighted composite (IL6–IL10) score based on interleukin (IL)-6 and IL-10 assays.

Fig. 3. Association between opioid peptides as indicated by a z unit weighted composite score based on dynorphin (DYN) and β-endorphin (END) assays (KOR-MOR) and immune activation as indicated by a z unit weighted composite score based on interleukin (IL)-6 and IL-10 assays (IL6-IL10).
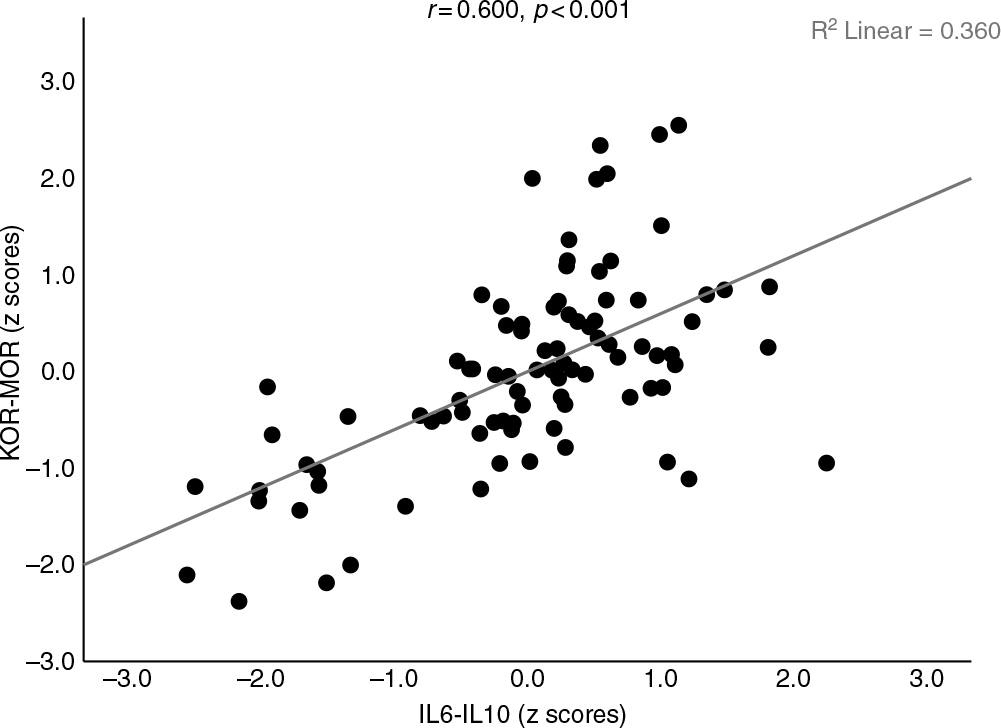
Fig. 4. Association between opioid receptor levels as indicated by a z unit weighted composite score based on kappa (KOR) and mu (MOR) opioid receptor levels (KOR-MOR) and immune activation as indicated by a z unit weighted composite score based on interleukin (IL)-6 and IL-10 assays (IL6-IL10).
Biomarker differences between controls and patients
In order to examine differences in the 10 biomarkers between the diagnostic groups, we have carried out multivariate GLM analysis while adjusting for age and BMI. Table 3 shows the outcome of the multivariate as well as univariate (tests for between-subject effects) GLM analyses. The biomarkers entered in this analysis were DYN, END, KOR, MOR, IL-6 and IL-10 levels as well as z unit weighted composite scores, namely DYN-KOR, DYN-END, KOR-MOR and IL6-IL10. We found a highly significant effect of diagnosis with an effect size of 0.483, while age and BMI were not significant. There were highly significant associations between all 10 biomarkers and diagnosis, with the strongest associations between diagnosis and IL6-IL10, END, DYN-END, IL-10 and KOR-MOR (with effect sizes > 0.300). Table 4 shows the model-generated estimated marginal mean values (in z scores) obtained by the GLM analyses shown in Table 3. As such, the z transformed biomarker data were adjusted for age and BMI and the differences observed between the study groups may be expressed in standard deviations. Pairwise multiple post-hoc analyses showed that DYN was significantly higher in MDD as compared with controls and MDD + ND. KOR, DYN-KOR and DYN-END were significantly different between the three study groups and increased from controls → MDD + ND → MDD. END, MOR, KOR-MOR, IL-6, IL-10 and IL6-IL10 were significantly higher in both MDD groups than in controls.
Table 3. Results of multivariate GLM analysis with the biomarkers as dependent variables and diagnosis as explanatory variable while adjusting for extraneous variables

Diagnosis, healthy controls versus depression with and without nicotine dependence; DYN, dynorphin; KOR, kappa opioid receptor; DYN-KOR, index of KOR signaling computed as z transformation of dynorphin (zDYN) + zKOR; END, β-endorphin; MOR, mu opioid receptor; DYN-END, integrated index of circulating opioid peptides, computed as zDYN + zEND; KOR-MOR, index of opioid receptor status computed as zKOR + zMOR; IL6-IL10, index of immune activation computed as z interleukin-6 (zIL6) + zIL10.
Table 4. Model-generated estimated marginal means of the 10 biomarkers obtained by GLM analysis shown in Table 2 in controls (HC) and major depressed (MDD) patients, divided into those with (MDD + ND) and without (MDD) nicotine dependence
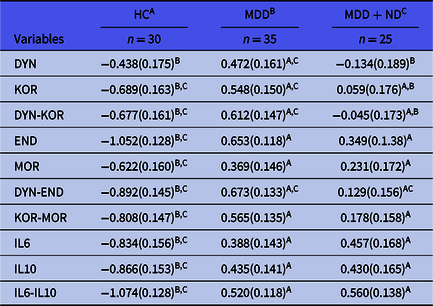
DYN, dynorphin; KOR, kappa opioid receptor; DYN-KOR, index of KOR signaling computed as z transformation of dynorphin (zDYN) + zKOR; END, β-endorphin; MOR, mu opioid receptor; DYN-END, integrated index of circulating opioid peptides, computed as zDYN + zEND; KOR-MOR, index of opioid receptor status computed as zKOR + zMOR; IL6-IL10, index of immune activation computed as z interleukin-6 (zIL6) + zIL10.
A,B,CResults of pairwise comparisons between group means.
All results are shows as mean (SE) and as z scores.
In order to delineate the most important biomarkers predicting MDD as well their odds ratios, we performed binary logistic regression analysis. Table 5 shows the outcome of a stepwise binary logistic regression analyses with MDD (versus controls) as dependent variable. IL6-IL10 and DYN-END were the best predictors of MDD (χ2 = 77.16, df = 2, p < 0.001) with an effect size of 0.800 (Nagelkerke value).
Table 5. Results of binary logistic regression analysis with major depression (controls as reference group) as dependent variable

OR, Odds ratio with 95% confidence intervals; IL6-IL10, computed as z (z interleukin-6 (zIL6) + zIL10)); DYN-END, computed as z (z dynorphin (zDYN) + z β-endorphin (zEND)).
Table 6 shows the results of receiver operating characteristic (ROC) analyses discriminating MDD from controls. The AUC ROC curves for DYN, KOR, DYN-KOR and DYN-END were computed on non-smoking MDD patients versus controls (because ND has an effect on these biomarkers). The other biomarkers were examined in all MDD patients versus controls. In the same table, we also show the bootstrapped AUC values after 2000 bootstraps. We found that DYN-END, END, KOR-MOR and IL6-IL10 yielded very high (bootstrapped) AUC (all > 0.849) separating MDD from controls. The best bootstrapped AUC was delineated for the combination of IL6-IL10 and DYN-END (0.972).
Table 6. Results of ROC

ROC, receiver operating characteristic; DYN, dynorphin; KOR, kappa opioid receptor; DYN-KOR, index of KOR signaling computed as z transformation of dynorphin (zDYN) + zKOR; END, β-endorphin; MOR, mu opioid receptor; DYN-END, integrated index of circulating opioid peptides, computed as zDYN + zEND; KOR-MOR, index of opioid receptor status computed as zKOR + zMOR; IL6-IL10, index of immune activation computed as z interleukin-6 (zIL6) + + zIL10; IL6-IL10 and DYN-END, as obtained using binary logistic regression (shown in Table 5).
Analysis discriminating major depression from normal controls.
* Performed in non-smokers only (all other analyses in all participants).
Discussion
The first major finding of this study is that serum dynorphin and KOR were significantly increased in MDD as compared to controls and that increases in those opioid peptides and their receptors are interrelated, suggesting that dynorphin/KOR signaling is significantly increased in MDD. However, two previous clinical studies were unable to observe signs of increased dynorphin/KOR signaling in depression (see Introduction: Hurd, Reference Hurd2002; Miller et al., Reference Miller, Zanderigo, Purushothaman, DeLorenzo, Rubin-Falcone, Ogden, Keilp, Oquendo, Nabulsi, Huang, Parsey, Carson and Mann2018). As described in the Introduction, increased KOR signaling results in anti-reward and dysphoric effects, thereby contributing to depressive behaviours (Carlezon & Thomas, Reference Carlezon and Thomas2009; Bruchas et al., Reference Bruchas, Land and Chavkin2010; Knoll & Carlezon, Reference Knoll and Carlezon2010; Lutz & Kieffer, Reference Lutz and Kieffer2013; Lalanne et al., Reference Lalanne, Ayranci, Kieffer and Lutz2014; Taylor & Manzella, Reference Taylor and Manzella2016; Bailey & Husbands, Reference Bailey and Husbands2018). Increased KOR signaling in limbic brain regions as a consequence of acute stress may induce many behavioural and pathophysiologic alterations while release of dynorphin may occur secondary to stress or may cause stress hormone release (Bilkei-Gorzo et al., Reference Bilkei-Gorzo, Racz, Michel, Mauer, Zimmer, Klingmüller and Zimmer2008; Land et al., Reference Land, Bruchas, Lemos, Xu, Melief and Chavkin2008). Dynorphin-mediated KOR activation may mediate the acute effects of stress while KOR activation may decrease neuronal activity (Knoll & Carlezon, Reference Knoll and Carlezon2010). Excitatory inputs are negatively regulated by dendritic dynorphin release in the hypothalamus and hippocampus via activation of presynaptic KORs (Iremonger & Bains, Reference Iremonger and Bains2009) and this mechanism may generalise to neuronal circuits that are involved in mood and motivation (Reyes et al., Reference Reyes, Chavkin and Van Bockstaele2009). Moreover, KORs may also regulate serotonergic (Bilkei-Gorzo et al., Reference Bilkei-Gorzo, Racz, Michel, Mauer, Zimmer, Klingmüller and Zimmer2008) and noradrenergic (Reyes et al., Reference Reyes, Johnson, Glaser, Commons and Van Bockstaele2007) systems, which may play a role in MDD. In animal models of the forced swim test, blockade of KORs decreases the immobility time (Mague et al., Reference Mague, Pliakas, Todtenkopf, Tomasiewicz, Zhang, Stevens, Jones, Portoghese and Carlezon2003). In stress conditions, KOR signaling elevates physical capacity (through analgesia) and the motivation to escape threat (through increasing aversion) thereby facilitating adaptive responses (Knoll & Carlezon, Reference Knoll and Carlezon2010).
The results of the current study on dynorphin and KOR are in agreement with findings that β-endorphin and MOR are upregulated in MDD and that the increases in β-endorphin and MOR are significantly intercorrelated, indicating increased β-endorphin/MOR signaling in MDD (Al-Fadhel et al., Reference Al-Fadhel, Al-Hakeim, Al-Dujaili and Maes2019). Previously, one paper showed increased expression of MOR in suicide victims, whereas positron emission tomography (PET) scan studies showed controversial results on MOR binding profiles (see Introduction). As reviewed in the Introduction, there are also some studies reporting lowered β-endorphin levels and suggesting lowered MOR signaling in MDD. These contradictory results may be explained by differences in the source (type of tissue or cells) used to measure these receptors (Stein et al., Reference Stein, Schäfer and Machelska2003; Rittner et al., Reference Rittner, Brack and Stein2008). Interestingly, MOR activation may elevate mood and therefore may improve depressive states (Shippenberg et al., Reference Shippenberg, Lefevour and Chefer2008). All in all, the results of different studies indicate that the opioid system as a whole is dysregulated in MDD with an upregulated dynorphin/KOR system and both up- and downregulation of the β-endorphin/MOR system. A plausible reason for the disparity of reported changes in β-endorphin levels in blood is the use of different inclusion criteria for patients and the heterogeneity of MDD.
The second major finding of this study is that the indices of dynorphin/KOR and β-endorphin and MOR levels are significantly intercorrelated and are strongly associated with immune inflammatory response system (IRS)/CIRS activation. Heightened IRS and CIRS responses are commonly observed in patients with MDD, with increased levels of immune-inflammatory biomarkers and immune-regulatory compounds (Krishnadas & Cavanagh, Reference Krishnadas and Cavanagh2012; Al-Hakeim et al., Reference Al-Hakeim, Al-Rammahi and Al-Dujaili2015; Haapakoski et al., Reference Haapakoski, Mathieu, Ebmeier, Alenius and Kivimaki2015; Köhler et al., Reference Köhler, Freitas, Maes, de Andrade, Liu, Fernandes, Stubbs, Solmi, Veronese, Herrmann, Raison, Miller, Lanctôt and Carvalho2017), including serum IL-6 and IL-10 (Köhler et al., Reference Köhler, Freitas, Maes, de Andrade, Liu, Fernandes, Stubbs, Solmi, Veronese, Herrmann, Raison, Miller, Lanctôt and Carvalho2017; Maes & Carvalho, Reference Maes and Carvalho2018; Al-Fadhel et al., Reference Al-Fadhel, Al-Hakeim, Al-Dujaili and Maes2019). Moreover, we observed that these opioid and IRS/CIRS responses yielded a good diagnostic performance for MDD with a bootstrapped (n = 2000) AUC of 0.972 (both opioid peptides combined with both cytokines). In this respect, it is intersting to note that classical neurotransmitters transduce information between neurons while cytokines and neuropeptides coordinate neuronal circuits or networks (Ludwig & Leng, Reference Ludwig and Leng2006; Leonard & Maes, Reference Leonard and Maes2012). This may explain that combinations of immune and opioid biomarkers have a higher sensitivity, specificity and diagnostic performance for MDD versus controls. Nevertheless, our data are insufficient to conclude that these measurements may be used as a biomarker for MDD because this conclusion entails that a biomarker set should be highly sensitive and specific for MDD versus other medical and neuropsychiatric disorders which are often characterised by aberrations in KOR/MOR and IRS/CIRS systems.
As described in the Introduction, there are many reciprocal relationships between the opioid and immune systems. Prossin et al. (Reference Prossin, Koch, Campbell, McInnis, Zalcman and Zubieta2011) reported that, in MDD, increased IL-18 (another pro-inflammatory protein) was positively associated with baseline MOR nondisplaceable binding potential and with sadness-associated MOR activation in amygdala, subgenual anterior cingulate and ventral basal ganglia. During inflammation, pro-inflammatory cytokines including IL-1β and IL-6 may stimulate hypothalamic corticotrophin releasing hormone (CRH) release (Maes et al., Reference Maes, Jacobs, Suy, Leclercq, Christiaens and Raus1990; Turnbull & Rivier, Reference Turnbull and Rivier1999). In response to CRH and cytokines, peripheral blood mononuclear cells may secrete opioids (Rittner et al., Reference Rittner, Machelska and Stein2005). Nevertheless, the major sources of local endogenous opioid ligands including β-endorphin and dynorphin are leukocytes (Machelska et al., Reference Machelska, Schopohl, Mousa, Labuz, Schäfer and Stein2003). For example, secretory granules with β-endorphin are present at the periphery of granulocytes, monocytes, macrophages and lymphocytes, and may undergo exocytosis (Ninković, Reference Ninković2013). During early inflammation, neutrophils contain most opioids while during later stages macrophages and monocytes and activated T and B cells are more important sources (Stein et al., Reference Stein, Schäfer and Machelska2003; Rittner et al., Reference Rittner, Brack and Stein2008). This may explain that leukocytes have analgesic effects by releasing opioids that consequently activate opioid receptors of nociceptors in the periphery (Ninković, Reference Ninković2013).
Dynorphins produced during inflammatory conditions display anti-inflammatory effects by attenuating translocation of nuclear factor-KB and consequently production of tumour necrosis factor-α and IL-1β (Rahiman et al., Reference Rahiman, Morgan, Gray, Shaw and Cabot2017). Inhibition of nuclear-factor KB is likely a major mechanism explaining the anti-inflammatory effects of opioids (Rahiman et al., Reference Rahiman, Morgan, Gray, Shaw and Cabot2017). Also, activation of KOR by dynorphins negatively regulates many immune cell functions including cytokine production by macrophages (Alicea et al., Reference Alicea, Belkowski, Eisenstein, Adler and Rogers1996), T cell proliferation (Guan et al., Reference Guan, Eisenstein, Adler and Rogers1997), phagocytoses (Szabo et al., Reference Szabo, Rojavin, Bussiere, Eisenstein, Adler and Rogers1993) and antibody production (Morgan, Reference Morgan1996). Moreover, dynorphins may exert some of their activities by binding to other receptors, including N-methyl-D-aspartate (NMDA) receptors, activating the hypothalamic–pituitary–adrenal (HPA) axis, inhibiting dopaminergic neurons and mediating changes in calcium channels leading to increased intracellular calcium levels (Jin et al., Reference Jin, Terman and Chavkin1997; Schwarzer, Reference Schwarzer2009).
Immune cells may upregulate POMC mRNA and contain all enzymes to process POMC into β-endorphin and adrenocorticotropic hormone (ACTH) (Machelska et al., Reference Machelska, Schopohl, Mousa, Labuz, Schäfer and Stein2003). These neuropeptides are secreted by the pituitary and arcuate nucleus (Stengaard-Pedersen & Larsson, Reference Stengaard-Pedersen and Larsson1981) leading to a concomitant increase in ACTH, β-endorphin and cortisol in MDD (Maes et al., Reference Maes, Jacobs, Suy, Leclercq, Christiaens and Raus1990; Maes et al., Reference Maes, Bosmans, Suy, Minner and Raus1991a; Maes et al., Reference Maes, Claes, Vandewoude, Schotte, Martin, Blockx and Cosyns1992; Al-Hakeim, Reference Al-Hakeim2008; Al-Fadhel et al., Reference Al-Fadhel, Al-Hakeim, Al-Dujaili and Maes2019). During inflammatory conditions, ‘CRH stimulates peripheral release of β-endorphin from immune cells’ (Berczi & Quintanar-Stephano, Reference Berczi and Quintanar-Stephano2016) and, therefore, activation of immune-inflammatory pathways likely contributes to circulating levels of β-endorphin. Animal data show that β-endorphins have immune-regulatory effects (Silberstein et al., Reference Silberstein, Vogl, Bonfiglio, Wurst, Holsboer, Arzt, Deussing and Refojo2009), which may explain the protective effects of CRH-stimulated elevations of β-endorphin during immune activation (Khairova et al., Reference Khairova, Machado-Vieira, Du and Manji2009). Inflammation also induces MOR transcription and increased MOR potency as observed in intestinal inflammation (Pol et al., Reference Pol, Alameda and Puig2001). MORs, in turn, have anti-inflammatory effects and MOR agonists may be used to dampen immune-inflammatory responses in, for example, inflammatory bowel disease (Philippe et al., Reference Philippe, Dubuquoy, Groux, Brun, Chuoï-Mariot, Gaveriaux-Ruff, Colombel, Kieffer and Desreumaux2003). All in all, our findings suggest that, in MDD, immune activation is associated with a simultaneous upregulation of dynorphin/KOR and β-endorphin/MOR signaling, which both may have immune-regulatory properties and, therefore, it may be hypothesised that increased signaling of both opioid systems may contribute to CIRS activities in MDD. Moreover, it may be hypothesised that increased dynorphin/KOR and β-endorphin/MOR signaling may play a role in depressive symptomatology whereby KOR has detrimental effects and MOR more protective effects.
The third major finding of this study is that KOR and the dynorphin/KOR signaling index are both significantly decreased in MDD subjects with comorbid ND, while there are no significant effects on β-endorphin or MOR levels (although there is a trend towards a decrease). Smoking is highly prevalent among people with MDD (Nunes et al., Reference Nunes, Vargas, Brum, Prado, Vargas, de Castro, Dodd and Berk2012) and is regarded as a means to cope with depressed mood and counteract fatigue and inactivity (Piper et al., Reference Piper, Piasecki, Federman, Bolt, Smith, Fiore and Baker2004). As explained in the Introduction, smoking is also a risk factor for depression through activated immune-inflammatory and oxidative pathways, while comorbid MDD and ND are characterised by lowered antioxidant and increased neuro-immune, neuro-oxidative and degenerative biomarkers (Rittner et al., Reference Rittner, Machelska and Stein2005; Bidlack et al., Reference Bidlack, Khimich, Parkhill, Sumagin, Sun and Tipton2006; Nunes et al., Reference Nunes, Vargas, Brum, Prado, Vargas, de Castro, Dodd and Berk2012). Isola et al., (Reference Isola, Zhang, Tejwani, Neff and Hadjiconstantinou2009) proposed that nicotine administration may influence dynorphin through alterations in dopamine and glutamate metabolism. Nicotine exposure increases dopamine release in mesolimbic terminal fields (Di Chiara, Reference Di Chiara2000) and, therefore, lowered dynorphin/KOR levels in comorbid MDD and nicotine use may be due to nicotine-induced dopamine release. Moreover, KOR activation may impact the reinforcing effects of nicotine (Ismayilova & Shoaib, Reference Ismayilova and Shoaib2010), while KOR agonists may lower locomotor activity induced by nicotine (Hahn et al., Reference Hahn, Stolerman and Shoaib2000). Mice without the prodynorphin gene show an increased intake of nicotine as compared to wild-type mice, indicating that dynorphin/KOR signaling may attenuate the reinforcing effects of nicotine (Galeote et al., Reference Galeote, Berrendero, Bura, Zimmer and Maldonado2009). Therefore, it is also possible that MDD patients with relatively lower dynorphin/KOR signaling display increased reinforcement effects of nicotine and thus more ND behaviours. Furthermore, due to lowered dynorphin/KOR signaling, heavy smokers may show lowered anti-inflammatory protection and, therefore, may be more vulnerable to the detrimental effects of smoking-induced IL-6 and TNF-α signaling (Lesperance et al., Reference Lesperance, Frasure-Smith, Theroux and Irwin2004; Nunes et al., Reference Nunes, Vargas, Brum, Prado, Vargas, de Castro, Dodd and Berk2012; Gaspersz et al., Reference Gaspersz, Lamers, Wittenberg, Beekman, van Hemert, Schoevers and Penninx2017).
Limitations of the study
The results of this study should be discussed with respect to its limitations. One limitation is that it would have been more interesting if we had measured DOR and many more cytokines including those produced by M1 macrophage, T helper (Th)-1, Th-2, Th-17 and T regulatory phenotypes in order to examine the associations of opioids with the full spectrum of IRS and CIRS cytokines and their ratios. Secondly, this study examined male subjects only and therefore, our study should be replicated in females. Thirdly, while the manufacturer of the kit used to assay MOR stated that there is no significant cross-reactivity between human MOR and analogues no such statement was reported for the KOR kit and therefore, cross-reactivity is not ruled out completely. Fourthly, it would be important to examine the effects of dynorphin and β-endorphin on the production of IRS/CIRS cytokines and the effects of immune activation (by mitogen or lipopolysaccharides (LPS) stimulation) on KOR-MOR protein production by stimulated and unstimulated whole blood cultures of patients and controls.
Conclusions
Serum dynorphin and KOR and β-endorphin and MOR are significantly increased in MDD as compared with controls. The increases in dynorphin/KOR and β-endorphin/MOR signaling are significantly intercorrelated with signs of IRS/CIRS activation. KOR and the dynorphin/KOR signaling index are both significantly decreased in depressed subjects with comorbid nicotine use. The findings of the present study suggest that the immune activation in MDD is associated with a simultaneous activation of dynorphin/KOR and β-endorphin/MOR signaling and that these opioid systems may participate in the pathophysiology of depression by exerting immune-regulatory activities as well as emotional and behavioural effects.
Acknowledgements
None.
Author contributions
All authors contributed significantly to the paper and approved the final version.
Financial support
There was no specific funding for this specific study.
Conflict of interest
The authors have no financial conflicts of interests.
Ethical standards
The authors assert that all procedures contributing to this work comply with the ethical standards of the relevant national and institutional committees on human experimentation and with the Helsinki Declaration of 1975, as revised in 2008.