Significant outcomes and limitations
-
Glucosamine, a common supplement for relieving atherosclerosis, could be a promising drug in the treatment of neuropathic pain. Antioxidant and anti-inflammatory activities are responsible for the antinociceptive effects of this drug.
-
Assessing thermal hyperalgesia by a radiant heat apparatus, which could have been directed at the injured paw, would have been more relevant.
-
Measuring mediators in the protein level will be the focus of further study.
-
Further clinical studies should be conducted to obtain optimal effective dose in human.
Introduction
Neuropathic pain is caused following trauma or disease of the peripheral or central nervous system. Population-based estimates of chronic pain with neuropathic characteristics range from 6.9% to 10% (van Hecke et al., Reference Van Hecke, Austin, Khan, Smith and Torrance2014). Two of the most common clinical pain symptoms are allodynia, a painful response to the non-noxious stimuli, and hyperalgesia, an exaggerated response to the noxious stimuli. Considering the adverse effects associated with current medications, neuropathic pain is still the most challenging aspect of pain management (Dworkin et al., Reference Dworkin, O'connor, Audette, Baron, Gourlay, Haanpaa, Kent, Krane, Lebel, Levy, Mackey, Mayer, Miaskowski, Raja, Rice, Schmader, Stacey, Stanos, Treede, Turk, Walco and Wells2010). Thus, defining optimal treatment and selecting rational and harmless analgesic drugs for patients suffering from chronic pain remain an ongoing research topic.
Accumulating evidence indicates that oxidative stress is a key determinant of behavioural and neuropathological consequences of neuropathic pain (Gwak et al., Reference Gwak, Hassler and Hulsebosch2013). Antioxidants may be a potential candidate for alleviation of neuropathic pain (Naik et al., Reference Naik, Tandan, Dudhgaonkar, Jadhav, Kataria, Prakash and Kumar2006, Valsecchi et al., Reference Valsecchi, Franchi, Panerai, Sacerdote, Trovato and Colleoni2008). Glucosamine (2-amino-2-deoxy-d-glucose; G\lcN), a natural amino monosaccharide synthesised from glucose, is an essential component of glycoproteins and proteoglycans. This compound stimulates synthesis of structural proteoglycans in the joint cartilage and inhibits cartilage degradation (Persiani et al., Reference Persiani, Roda, Rovati, Locatelli, Giacovelli and Roda2005). There are many types of evidence that support the positive effects of glucosamine in patients with rheumatoid arthritis (Hua et al., Reference Hua, Suguro, Hirano, Sakamoto and Nagaoka2005, Gilzad-Kohan & Jamali, Reference Gilzad-Kohan and Jamali2012, Tsuji et al., Reference Tsuji, Yoon, Kitano, Okura and Tanaka2015). Glucosamine has been shown to exert a protective effect against two models of toxic liver damage (Sal'nikova et al., Reference Sal'nikova, Drogovoz and Zupanets1990). Beneficial effects of this compound have been reported in the experimental autoimmune encephalomyelitis (EAE), an animal model of multiple sclerosis (Zhang et al., Reference Zhang, Yu, Gran and Rostami2005), as well as scopolamine-induced impairment of spatial learning and memory (Jamialahmadi et al., Reference Jamialahmadi, Sadeghnia, Mohammadi, Kazemabad and Hosseini2013). It has also shown a protective effect after middle cerebral artery occlusion in rats (Hwang et al., Reference Hwang, Shin, Hwang, Kim, Shin, Oh, Oh, Kim, Lee and Han2010), and in morphine tolerance (Basiri et al., Reference Basiri, Rad, Mahdian, Molavi and Amin2019).
This study was aimed to explore the effects of glucosamine in attenuating allodynia and hyperalgesia induced after sciatic nerve constriction as a model of neuropathic pain, in two regimens of prophylactic and therapeutic treatment. Gabapentin was used as a reference compound for positive control. As many studies indicated a connection between behavioural changes and spinal cord molecular changes (Pertovaara et al., Reference Pertovaara, Wei, Kalmari and Ruotsalainen2001), mRNA expression of inducible nitric oxide synthase (iNOS), a key enzyme generating free radical signalling molecule, nitric oxide (NO) was evaluated in the spinal cord of animals. Additionally, production of the pro-inflammatory cytokine, tumour necrosis factor alpha (TNF-α), was measured at the levels of mRNA and protein.
Materials and methods
Animals
Sixty-four adult male Wistar rats (weight: 250–270 g) were obtained from the animal house (Sabzevar University of Medical Sciences) and provided with free access to food and water, under a 12/12-h light-dark cycle (light cycle: 7:00 A.M.–7:00 P.M.). Animals were acclimated to the laboratory environment for 5–7 days before being used in the experiments and kept in groups of 2 in the open cages (24 × 40 × 20 cm). All experimental procedures were approved by the Institutional Animal Care and Use Committee of the Sabzevar University of Medical Sciences (IR.MEDSAB.REC.93.87). All experiments were conducted in accordance with the International Association for the Study of Pain (IASP) guidelines on the care and use of experimental animals (Zimmermann, Reference Zimmermann1983).
Materials
Glucosamine was purchased from sigma-Aldrich (Germany). Gabapentin (donated by Tehran Darou Pharmaceutical Co., Tehran, Iran) was dissolved in saline solution (0.9% NaCl). Ketamine and xylazine were obtained from Alfasan Pharmaceutical Co., Woerden, Holland.
Chronic constriction injury
Peripheral nerve injury was created by loosely ligating the sciatic nerve in male rats after induction of anaesthesia by ketamine and xylazine cocktail (64 and 1.6 mg/kg, i.p. respectively). For monitoring the anaesthetic dept, we pinched the tail. The shaved area was scrubbed with alcohol, betadine and then mid-thigh was incised. After separation of the heads of the biceps femoris, the left sciatic nerve was exposed above the level of trifurcation. Four loose ligations with a double knot, 1 mm spacing were carefully applied to the nerve using absorbable chromic gut. Constriction of the nerve was minimal until a brief twitch was detected. The surgical site was closed; muscle and skin were then tightly ligated with 4–0 silk thread. Eventually, rats were returned to their cages to recover (Bennett et al., Reference Bennett, Chung, Honore and Seltzer2003).
Sample size calculation
The group sizes were based on previous studies in the lab using similar models (Charan & Kantharia, Reference Charan and Kantharia2013; Basiri et al., Reference Basiri, Rad, Mahdian, Molavi and Amin2019).
Experimental protocol
Animals were randomly allocated to eight groups, each comprising eight male Wistar rats.
1. Chronic constriction injury (CCI) animals: Rats were subjected to the surgical procedure to the left sciatic nerve and treated daily with vehicle (normal saline 9%), via gavage route, 1 day before CCI, up to day 14.
2. Sham animals: Rats that their left sciatic nerve were exposed at the same level without any manipulation on it.
3, 4, 5. CCI animals treated with daily glucosamine at the doses of 500, 1000 and 2000 mg/kg, via gavage route, 1 day before CCI, up to day 14 post-CCI (prophylactic groups).
6. CCI animals treated with daily gabapentin (180 mg/kg) via gavage route, 1 day before CCI, up to day 14 post-CCI.
7. CCI animals treated with daily glucosamine, 1000 mg/kg from day 5 to 14 post-CCI (therapeutic group).
8. CCI animals treated with daily gabapentin (180 mg/kg) via gavage route, from day 5 to 14 post-CCI.
Selected drug dosages were based on earlier studies (Jamialahmadi et al., Reference Jamialahmadi, Sadeghnia, Mohammadi, Kazemabad and Hosseini2013; Al-Mahmood et al., Reference Al-Mahmood, Abdullah, Nik Ahmad, Mohamed and Tariq2016). All the tests were conducted at the same time in the afternoon (between 9 A.M. and 2 P.M.), by a person who was blinded to the experiment, to minimise the effects of subjective bias.
Mechanical allodynia test
The Von Frey filaments (Steeling, Wood Dale, IL, USA), in the range of 2.0, 4.0, 6.0, 8.0, 10.0, 15.0, 26.0 and 60 g forces, were used to measure mechanical allodynia by testing the paw withdrawal threshold (up-down method). For this purpose, rats were individually placed in an elevated transparent box (diameter: 33 cm), with a wire mesh floor and allowed to adapt to testing environment for 10 min. Experiment was started with the filament produced the lowest force. The filament was applied to the plantar surface of the hind paw until the filament buckled slightly. The absence of a response after ≈5 s prompted use of the next filament of increasing weight. It was defined as the response, if three withdrawals were detected following five times application of a filament. To prevent tissue injury, 60 g was considered as the cut-off value (Nielsen et al., Reference Nielsen, Lewis, Alewood, Drinkwater, Palant, Patterson, Yaksh, McCumber and Smit2005).
Cold allodynia test
Cold allodynia was evaluated 15 min after performing previous test (mechanical allodynia). A drop of acetone was placed in the centre of injured hind paw. This trial was repeated five times with an interval of 1 min. The frequency of foot withdrawal was expressed as a percentage: (number of trials accompanied by brisk foot withdrawal) × 100/(number of total trials) (Amin et al., Reference Amin, Heravi Taheri and Hosseinzadeh2014).
Thermal paw withdrawal test
After passing 15 min of previous test (cold allodynia), for measuring the hotplate latency, a rectangular glass surface was heated to a temperature of 52±0.5°C (Model 39D Analgesia meter IITC, Borjsanat, Iran). The animals were placed on the hot plate surrounded by a Plexiglas cylinder and the response latency was the latency observed from the time the rat was placed on the heated surface until the first reflex such as (i) the rat licking a hind paw, (ii) vocalisation, or (iii) an escape response. The timer was stopped by a foot-operated pedal and the rat was immediately removed from the hotplate. A maximum hotplate latency of 30 s was used to prevent tissue damage to the rat’s paws (Baliki et al., Reference Baliki, Calvo, Chialvo and Apkarian2005; Eddy & Leimbach, Reference Eddy and Leimbach1953). Average of the 3–4 values was measured to identify thermal hyperalgesia.
Assessment of sensory thresholds tests was taken at the beginning of the experiment (day 0) and on days 3, 5, 7, 10 and 14 after that. No discrimination was made between responses related to the nerve-injured or uninjured paws, but any response was considered equally.
Real-time polymerase chain reaction (qRT-PCR)
After final behavioural experiments, animals were decapitated; samples were rapidly collected and frozen in the liquid nitrogen. A piece (2 cm) of spinal cord at the lumbar section was harvested from each animal (Schmittgen & Livak, Reference Schmittgen and Livak2008). Samples were then stored at −80°C until usage for extraction of total RNA and protein. Groups sizes were 8 for behaviour work. However, due to the destruction of 1–2 samples in some groups, we omitted data belonging to these samples in all groups and n = 6 from each group was included for tissue analysis. RT-PCR was performed with total RNA extracted from the homogenised sample (approximately, 1 mg tissue), using RNX-plus (MR7713C, Sinaclon, Iran). Although no band of DNA was observed in gel, we designed exon-exon junction primers and ruled out DNAs contamination with minus RT controls in each experiment. Complimentary DNA (cDNA) was generated by reverse transcription from 2 μg RNA using the PrimeScript™ RT reagent Kit (Takara Bio Inc., Japan) following manufacturer’s guidelines. RT-PCR was run using 2 μg cDNA and SYBR green PCR master mix (YTA, Iran) on CFX96 Touch™ Real-Time PCR Detection System (Bio-Rad, Philadelphia, PA USA), using a programme of 10 min at 95°C, 35 cycles of 15 s at 95°C, 30 s at 57°C and 45 s at 72°C. The data were normalised by glyceraldehyde 3-phosphate dehydrogenase expression, using ΔΔCT method to evaluate the fold change of gene expression (Schmittgen & Livak, Reference Schmittgen and Livak2008). Each reaction was performed in triplicates. The PCR primer sequences are presented in Table 1.
Table 1. Primers specific for rat inducible nitric oxide synthase (iNOS), tumour necrosis factor alpha (TNF-α) and glyceraldehyde 3-phosphate dehydrogenase (GAPDH), forward and reverse

F, forward primer sequence; R, reverse primer sequence.
Enzyme-linked immunosorbent assay (ELISA) analysis
At the time of experiment, samples were thawed at room temperature and homogenised using a tissue homogeniser (Heidolph, Germany) in a homogenisation buffer consisting 20 mmol/L Tris (pH 7.4; Sigma-Aldrich), 150 mmol/L NaCl (Sigma-Aldrich), 1 mmol/L ethylene diamine tetraacetic acid (Sigma-Aldrich), 2 mmol/L 2-N-morpholinoethanesulfonic acid or 2 ME (Sigma-Aldrich) and protease inhibitor (Sigma-Aldrich) (Rahbardar et al., Reference Rahbardar, Amin, Mehri, Mirnajafi-Zadeh and Hosseinzadeh2018). Total protein contents were determined by the Bradford assay and adjusted (Bradford, Reference Bradford1976). Cytokine levels were measured by the commercially available ELISA specific for TNF-α (Diaclone, France). Analysis of protein content was performed according to the manufacturer’s instructions from the standard curve.
Statistical analysis
Statistical testing was carried out using Prism 6.0 (GraphPad, San Diego, CA). All data are expressed as the mean ± standard error of the mean. Statistical significance was set at a p value less than 0.05, confidence interval (CI) 90%. For the behavioural tests of cold allodynia and thermal hyperalgesia, the two-way analysis of variance (ANOVA) with repeated measure followed by Bonferroni post-hoc test was used. Kruskal–Wallis test followed by Mann–Whitney for multiple comparisons was used to evaluate mechanical allodynia. For molecular measurements, one-way ANOVA with Tukey’s multiple comparisons was used.
Results
Effect of glucosamine on the CCI-induced tactile allodynia
One day before CCI, there was not any significant difference among the six groups of rats. There was a significant difference after the sciatic nerve injury among groups for days 3 (H(5) = 15.53), 5 (H(5) = 19.47), 7 (H(5) = 21.4), 10 (H(5) = 19.67) and 14 (H(5) = 19.75), p = 0.001. CCI + vehicle rats exhibited robust mechanical allodynia relative to sham animals which was detected from day 3 (11 ± 1.34 vs. 41.62 ± 8.75; p < 0.01) and continued up to day 14 post-CCI (3 ± 0.7 vs. 47.25 ± 7.3; p < 0.01) (Fig. 1A).
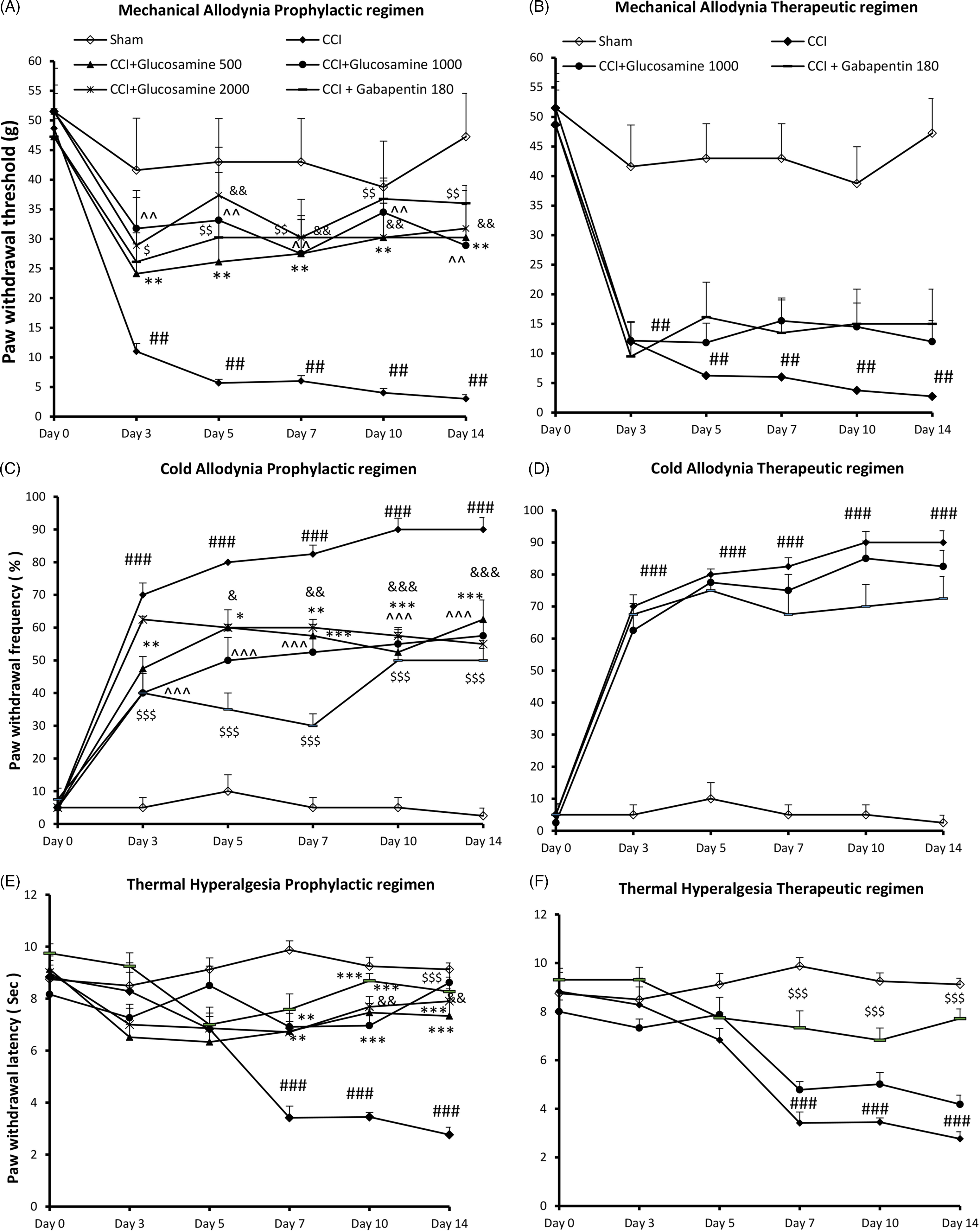
Fig. 1. Time course of (A, B): tactile allodynia, (C, D): cold allodynia and (E, F): thermal hyperalgesia responses, in ipsilateral hind paws of CCI rats following administration of vehicle, 500, 1000, 2000 mg/kg glucosamine via gavage route, either in prophylactic (from 1 day before CCI until day 14 post-CCI) or therapeutic regimens (from 5 days after CCI until day 14 post-CCI). Each point represents mean ± SEM. n = 8 rats in each group. Statistical test was repeated measures ANOVA with Bonferroni multiple comparison. ##p < 0.01, ###p < 0.001 indicate comparison between sham and vehicle CCI group. *p < 0.05, **p < 0.01, ***p < 0.001 glucosamine 500 vs. vehicle CCI group. ^p < 0.05, ^^p < 0.01, ^^^p < 0.001 glucosamine 1000 vs. vehicle CCI group. &p < 0.05, &&p < 0.01, &&&p < 0.001 glucosamine 2000 vs. vehicle CCI group. $p < 0.05, $$p < 0.01, $$$p < 0.001 gabapentin 180 vs. vehicle CCI group.
As compared to vehicle-treated CCI animals, those receiving glucosamine (500 mg/kg, 1 day before surgery up to day 14 post-CCI) showed attenuated mechanical allodynia on days 3 (24.12 ± 6.9), 5 (26.12 ± 6.9), 7 (27.5 ± 6.4; p < 0.01), 10 (30.25 ± 5.7; p < 0.01) and 14 (3.25 ± 5.8; p < 0.01). Compared to vehicle-treated CCI animals, rats receiving glucosamine (1000 mg/kg, 1 day before surgery up to day 14 post-CCI) showed attenuated mechanical allodynia on days 3 (28.8 ± 6.42; p < 0.05), 5 (37.37 ± 8.1; p < 0.01), 7 (3.25 ± 5.8; p < 0.01), 10 (29.5 ± 6.8; p < 0.01) and 14 (31.5 ± 6.9; p < 0.01). Glucosamine 2000 mg/kg also produced a significant mechanical anti-allodynic effect compared to vehicle, on days 3 (31.05 ± 8.1), 5 (32.12 ± 8.1), 7 (27.5 ± 6.42; p < 0.01), 10 (31.5 ± 5.78; p < 0.01) and 14 (28.8 ± 6.42; p < 0.01). Anti-allodynic effect of gabapentin 180 mg/kg when administered 1 day before surgery up to day 14 post-CCI produced from day 3 (26.12 ± 6.42) and remained up to day 14 (35 ± 8.74; p < 0.01; Fig. 1A).
Administration of glucosamine (1000 mg/kg) 5 days after CCI for a period of 10 days (therapeutic group) failed to reverse mechanical allodynia in CCI animals, as compared to vehicle-treated CCI animals. Gabapentin as a reference drug was also not able to attenuate induced mechanical allodynia when administered from day 5 post-CCI (Fig. 1B).
Effect of glucosamine on the CCI-induced cold allodynia
On day 0, before CCI, there was not a significant difference among the six groups of rats. There was a significant effect with time effect (F (5, 25) = 109.6, p < 0.001), treatment effect (F (5, 25) = 44.7, p < 0.001) and time*treatment interaction (F (25, 125) = 4.5, p < 0.001) after the sciatic nerve injury (Fig. 1C). In comparison to sham group, CCI + vehicle animals significantly exhibited an increased frequency of response to acetone drop on day 3 (70 ± 3.6 vs. 6.7 ± 3.4; p < 0.001) which continued until the end of study (90 ± 10.9 vs. 3.5 ± 2.7; p < 0.001).
Relative to vehicle, glucosamine 500 and 1000 mg/kg attenuated cold allodynia on days 3 (47.5 ± 3.6, 40 ± 5.9; p < 0.01, p < 0.001), 5 (60 ± 5.4, 50 ± 6.9; p < 0.05, p < 0.001), 7 (57.5 ± 5, 52.5 ± 5.1; p < 0.001), 10 (52.5 ± 6.8, 55 ± 5.1; P < 0.001) and 14 (62.5 ± 5.9, 57.5 ± 4.2; p < 0.001), respectively. Glucosamine 2000 mg/kg attenuated cold allodynia in CCI animal as compared to vehicle, on days 5 (60 ± 8.9; p < 0.05), 7 (60 ± 5.9; p < 0.01), 10 (57.5 ± 4.6; p < 0.001) and 14 (55 ± 2.5; p < 0.001) (Fig. 1C). Anti-allodynic effect of gabapentin against acetone drop produced from day 3 (40 ± 6.5; p < 0.001) and remained up to day 14 (50 ± 3.6; p < 0.001) (Fig. 1C).
For the therapeutic groups, there was a significant effect with time effect (F (5, 25) = 75.75, p < 0.001), treatment effect (F (3, 15) = 90.78, p < 0.001) and time*treatment interaction (F (15, 75) = 16.27, p < 0.001) after the sciatic nerve injury. Glucosamine (1000 mg/kg) in the therapeutic regimen, after the induction of neuropathic pain, was not able to attenuate hypersensitivity to acetone drop as compared to CCI + vehicle animals. Hypersensitivity to acetone drop was also did not changed by gabapentin 180 mg/kg when administered after 5 days of CCI (Fig. 1D).
Effect of glucosamine on the CCI-induced thermal hyperalgesia
There was a significant effect with time effect (F (5, 25) = 6.39, p < 0.01), treatment effect (F (5, 25) = 20.12, p < 0.001) and time * treatment interaction (F (25, 125) = 3.96, p < 0.001) after the sciatic nerve injury. Before CCI, on day 0, there was not a significant different among the six groups of rats. Compared to sham group, rats underwent CCI and treated with vehicle exhibited a late-onset thermal hyperalgesia, which started on 7 (3.4 ± 0.2 vs. 12 ± 0.76; p < 0.001) and remained up to the end of study (2.8 ± 0.3 vs. 12 ± 0.95, p < 0.001) (Fig. 1E). In contrast, treatment with glucosamine 500 and 1000 mg/kg attenuated thermal hyperalgesia in comparison to CCI + vehicle animals on days 7 (5.1 ± 0.5, 5.2 ± 0.2; p < 0.01), 10 (7.4 ± 0.3, 6.6 ± 0.5, p < 0.001) and 14 (8.5 ± 0.47, 7.7 ± 0.4; p < 0.001). Moreover, glucosamine 2000 mg/kg attenuated thermal hyperalgesia on days 7 (6.5 ± 0.35), 10 (7.7 ± 0.45) and 14 (7 ± 0.48; p < 0.001). In comparison to CCI + vehicle animals, gabapentin 180 mg/kg (1 day before surgery up to day 14 post-CCI) attenuated thermal hyperalgesia on days 7 (7.1 ± 0.57), 10 (5.68 ± 1) and 14 (6.45 ± 0.4; p < 0.001) (Fig. 1E).
For the therapeutic groups, there was a significant effect with time effect (F (5, 25) = 19.37, p < 0.001), treatment effect (F (3, 15) = 31.45, p < 0.001) and time*treatment interaction (F (15, 75) = 4.66, p < 0.001) after the sciatic nerve injury. Glucosamine (1000 mg/kg) given to animals 5 days after CCI for 10 days, was not able to attenuate thermal hyperalgesia relative to CCI + vehicle animals. In contrast, hypersensitivity to thermal stimulus was significantly mitigated by gabapentin (5 days after CCI for 10 days), on days 7 (7.3 ± 0.7), 10 (6.8 ± 0.5) and 14 (7.7 ± 0.4; p < 0.001) (Fig. 1F).
Effect of glucosamine on the spinal iNOS expression
ANOVA showed that there was a significant effect among groups (F (7, 47) = 3.6, p < 0.01). Spinal cord iNOS was higher in CCI animals treated with vehicle as compared to sham animals (1.7 ± 0.27 vs. 1; p < 0.01). The injury-induced increase in the iNOS expression was significantly decreased in those treated with glucosamine at three doses of 500 (1.6 ± 0.0.05; p < 0.05), 1000 (1 ± 0.11; p < 0.05) and 2000 mg/kg in prophylactic regimen, as compared to CCI vehicle (1.3 ± 0.1; p < 0.05) (Fig. 2A). Animals treated with gabapentin (180 mg/kg) also exhibited a significant reduction in iNOS gene compared to CCI + vehicle animals (1.2 ± 0.05; p < 0.05). Neither glucosamine 1000 mg/kg nor gabapentin 180 mg/kg in the therapeutic regimen could attenuate mRNA iNOS in the spinal cord of CCI animals (Fig. 2A).

Fig. 2. Effect of glucosamine (500, 1000, 2000 mg/kg, via gavage route), on the (A): mRNA levels of iNOS (inducible nitric oxide synthase) and (B): TNF-α (tumour necrosis factor alpha) in the spinal cords of CCI animals. GAPDH (glyceraldehyde 3-phosphate dehydrogenase) is a reference gene and was used for normalisation as an internal control. Results (mean ± SEM) are expressed in terms of relative quantification (fold change) using the 2-ΔΔCT method. (C): TNF-α protein level was measured using ELISA assay. Values are means ± SEM (n = 6). One-way ANOVA and post-hoc Tukey’s were used. #p < 0.05, ##p < 0.01, indicate comparison between sham and vehicle CCI group. *p < 0.05, **p < 0.01 vs. vehicle CCI group.
Effect of glucosamine on the spinal TNF-α gene expression and protein content
ANOVA showed that there was a significant effect among groups (F (7, 47) = 10.4, p < 0.001). Spinal cord TNF-α was higher in CCI animals treated with normal saline than in sham animals (1.7 ± 0.27 vs. 1; p < 0.01). mRNA TNF-α was enhanced in CCI-vehicle animals compared to sham ones (2.4 ± 0.2 vs. 1; p < 0.01). Glucosamine 500 (1.42 ± 0.16; p < 0.01), 1000 (1.7 ± 0.16; p < 0.05) and 2000 mg/kg (1.5 ± 0.1; p < 0.01) decreased TNF-α expression compared to CCI + vehicle animals. Gabapentin in prophylactic regimen was also able to attenuate gene expression level of TNF-α (1.7 ± 0.13; p < 0.01) (Fig. 2B).
Neither glucosamine 1000 mg/kg nor gabapentin 180 mg/kg in the therapeutic regimens could attenuate mRNA TNF-α in the spinal cord of CCI animals (Fig. 2B).
CCI injury caused a significant increase in the concentration of TNF-α at the protein level (79 ± 7.6 vs. 16.2 ± 4.96; p < 0.01) as compared to sham group; however, the increased TNF-α was inhibited by glucosamine at three doses of 500 (36 ± 8.2; p < 0.01), 1000 (47.3 ± 5.57; p < 0.01) and 2000 mg/kg (36 ± 6.5; p < 0.001) in prophylactic regimen, as compared to CCI + vehicle animals. Similar observation was detected in those animals treated with gabapentin 180 mg/kg in the prophylactic regimen as compared to CCI-vehicle animals (45.7 ± 7.5; p < 0.01).
TNF-α protein was not changed in the spinal cord of CCI animals treated with glucosamine (1000 mg/kg) or gabapentin 180 mg/kg in the therapeutic regimen (Fig. 2C).
Discussion
The CCI animals reliably showed an enhancement of withdrawal reflexes in response to von Frey filaments (tactile allodynia), acetone drop (cold allodynia) and hot plate (thermal hyperalgesia), relative to sham-operated rats. In rats exposed to CCI, 15 days administration of glucosamine (500, 1000, 2000 mg/kg) via gavage rout, started 1 day before the induction of nerve injury up to day 14 post-CCI, attenuated mechanical allodynia, cold allodynia and thermal hyperalgesia. Treatment with glucosamine (1000 mg/kg, via gavage route), after the induction of pain, from days 5 to 14, showed no significant improvement in the parameters of pain.
Mechanisms underlying neuropathic pain remain largely unknown; however, oxidative stress has been demonstrated to play a key role in neuropathic conditions (Naik et al., Reference Naik, Tandan, Dudhgaonkar, Jadhav, Kataria, Prakash and Kumar2006; Rajanandh et al., Reference Rajanandh, Kosey and Prathiksha2014). It has become increasingly clear that NO over-production by iNOS is deleterious after nerve injury. NO as a free radical activates glia to release inflammatory mediators which increase sensitivity and excitability of primary sensory neurons and synaptic plasticity in central nervous system. All of these changes have been known as the central sensitisation (Conti et al., Reference Conti, Miscusi, Cardali, Germano, Suzuki, Cuzzocrea and Tomasello2007). Eventually, appearance of peripheral and central sensitisations is responsible for allodynia and hyperalgesia. Inhibition of iNOS has shown beneficial effects in attenuating pain of neuropathic rats (Staunton et al., Reference Staunton, Barrett-Jolley, Djouhri and Thippeswamy2018). In agreement with this, in the current study gene expression level of iNOS was increased in the spinal cords of CCI animals, whereas it was attenuated in those animals prophylactically treated with glucosamine.
Spinal glial cells activation upon a traumatic nerve injury releases pro-inflammatory cytokines such as TNF-α, which contributes to the maintenance of chronic neuropathic pain via stimulating sensory neuronal excitability (Carniglia et al., Reference Carniglia, Ramirez, Durand, Saba, Turati, Caruso, Scimonelli and Lasaga2017, Rahbardar et al., Reference Rahbardar, Amin, Mehri, Mirnajafi-Zadeh and Hosseinzadeh2018). In this study, the increased gene expression level of TNF-α in the spinal cord following CCI was dampened by glucosamine in the prophylactic regimen. The expression of TNF-α at the protein level determined by ELISA assay showed a significant increase in CCI animals compared to sham rats. However, treatment with glucosamine in prophylactic regimen attenuated its protein content which confirms the results from the qRT-PCR. In contrast, glucosamine (1000 mg/kg) after the induction of neuropathic pain was not able to reduce inflammatory mediators in the spinal cord of CCI animals which is in line with the behavioural results.
Anti-inflammatory effects of glucosamine have been investigated in previous studies. In agreement with our results, different routes administration of glucosamine (oral, i.p., or i.v.) significantly suppressed microglia activation and decreased TNF-α, IFN-γ, interleukin-17 (IL-17), whereas up-regulated Th2 cytokines (IL-5 and IL-10) in an acute but not chronic phase of EAE (Zhang et al., Reference Zhang, Yu, Gran and Rostami2005).
Whether glucosamine has multiple targets or it only affects a special target and consequently activates other signals are not totally clarified. It is possible that a combination of factors involves in the antinociceptive effects observed by glucosamine.
Proposed anti-inflammatory and antioxidant effects of glucosamine could be done by 1) chelating ions such as Fe2+ (Yan et al., Reference Yan, Wanshun, Baoqin, Changhong, Chenwei, Bing and Liehuan2007), 2) increasing the amounts of a protein called O-GlcNAcylation, which regulates neuronal synaptic strength and attenuates hyperexcitability of neurons in brain (Allen & Rapraeger, Reference Allen and Rapraeger2003), 3) inhibiting gene expression of NLRP3, a protein promotes the pathogenesis of metabolic, neurodegenerative and infectious diseases (Chiu et al., Reference Chiu, Li, Hsieh, Rao, Chen, Chen, Ka and Hua2019), 4) inhibiting the activity of NF-κB, responsible for transactivation of the iNOS and TNF-α gene (Hwang et al., Reference Hwang, Shin, Hwang, Kim, Shin, Oh, Oh, Kim, Lee and Han2010; Largo et al., Reference Largo, Alvarez-Soria, Diez-Ortego, Calvo, Sanchez-Pernaute, Egido and Herrero-Beaumont2003; Shin et al., Reference Shin, Hwang, Kim, Oh, Nam and Han2013), as well as 5) a significant upregulation of immunomodulatory molecule, galectin-3 (Gal-3) (Rahimian et al., Reference Rahimian, Lalancette-Hébert, ChengWeng, Sato and Kriz2020).
In the present work, considering that late administration of glucosamine, 5 days after the nerve injury failed to attenuate existing pain, it might reflect the inability of glucosamine to inhibit activated inflammatory responses after nerve injury. As reported by Raghavendra et al. (Reference Raghavendra, Tanga and Deleo2003), inhibition of activated microglia with minocycline failed to attenuate existing pain in neuropathic rats.
Our results indicated that prophylactic administration of the reference drug, gabapentin not only produced anti-allodynic and antihyperalgesic effects but also inhibited both TNF-α and iNOS in the spinal cord of CCI animals, which is supported by previous studies (Wodarski et al., Reference Wodarski, Clark, Grist, Marchand and Malcangio2009). Furthermore, administration of gabapentin starting on day 5 (therapeutic regimen) was able to attenuate thermal hyperalgesia but not mechanical and cold allodynia. However, spinal TNF-α and iNOS were not reduced in this regimen. Based on our results, it might be suggested that different mechanisms are involved in the CCI-induced hyperalgesia and allodynia (Ossipov et al., Reference Ossipov, Lai, Malan and Porreca2000).
In this study, administration of gabapentin 5 days after CCI was not able to attenuate mechanical allodynia. Considering that gabapentin as a reference drug has shown anti-allodynic affects after injury, it might be suggested that higher doses should be administered for anti-allodynic effects of gabapentin.
One of the advantages of glucosamine is its safety profile. According to Setnikar et al. (Reference Setnikar, Cereda, Pacini and Revel1991) study, glucosamine showed an anti-inflammatory effect with very low gastrointestinal adverse effects compared to NSAIDs (non stroidal anti inflammatory drugs). In agreement with this, no serious toxicity of this drug has been reported previously (Persiani et al., Reference Persiani, Roda, Rovati, Locatelli, Giacovelli and Roda2005).
However, gabapentin as a common analgesic drug prescribed in neuropathic patients has many adverse effects (Lindner et al., Reference Lindner, Bourin, Chen, McElroy, Leet, Hogan, Stock and Machet2006). In a Cochrane review study, gabapentin at high doses ranging from 1800 to 3600 mg daily produced moderate benefit (at least 30% pain relief) in participants with diabetic neuropathic pain. Additionally, this drug was accompanied with side effects such as dizziness (19%), somnolence (14%), peripheral oedema (7%) and gait disturbance (14%) compared to placebo, which lower compliance of patients (Wiffen et al., Reference Wiffen, Derry, Bell, Rice, Tolle, Phillips and Moore2017).
One of limitations of our study using hot plate for measuring thermal hypersensitivity was that there was no discrimination between responses from the injured vs uninjured paws, and that the animal could in theory have limited contact between the injured paw and the plate. A laser-evoked stimulus, which could have been placed directly at the injured paw, would have been more relevant. It should be noted that this study is an experimental study on rats; therefore, more examinations should be performed to obtain optimal doses in human.
A concern about glucosamine is its effect on glucose haemostasis and decreased insulin sensitivity which should be considered in diabetic patients (Dostrovsky et al., Reference Dostrovsky, Towheed, Hudson and Anastassiades2011).
Considering that combination therapy is very important in neuropathic pain (Holbech et al., Reference Holbech, Jung, Jonsson, Wanning, Bredahl and W.Bach2017), adding glucosamine to common approved analgesic drugs could achieve better improvement in patients with neuropathic pain. Combining different drugs can interact with multiple targets and decrease side effects (Chaparro et al., Reference Chaparro, Wiffen, Moore and Gilron2012). It has been reported that antioxidant supplementation has a higher outcome in combination therapy (Meymandi et al., Reference Meymandi, Sepehri, Abdolsamadi, Shaabani, Heravi, Yazdanpanah and Aghtaei2017).
Conclusion
The current findings suggest that prophylactic treatment with glucosamine could prevent nerve injury-induced neuropathic pain, and that this may happen via modulating the gene transcription of iNOS and pro-inflammatory cytokine, TNF-α. Considering that combination therapy for neuropathic pain has attracted much attention in recent years, in further studies evaluating combination of this drug with other analgesics with different mechanisms could result in a better analgesic effect with lower doses and therefore lower unwanted effects.
Acknowledgements
We are grateful to vice chancellor of research, Sabzevar University of Medical Sciences, Sabzevar, Iran.
Author contributions
Ehsan Mohebbi: conducted handling of animals, behavioural and biochemical tests. Mehdi Molavi: contributed to the preparation of final manuscript. Mohammad Sahebkar: performed data analysing and contributed to the preparation of final manuscript. Bahareh Amin: presented the idea and contributed to the preparation and revision of final manuscript and supervised the project.
Conflict of interest
The authors declare that there is no conflict of interest in this work.